Clinical significance of pretreatment tumor growth rate for locally advanced non-small cell lung cancer
Introduction
Locally advanced non-small cell lung cancer (NSCLC) harbors a relatively poor prognosis, with 5-year survival rates of 13–32% after definitive chemoradiation therapy (CRT) (1,2). On sequential imaging studies from diagnosis to therapy, clinicians often observe disease growth during this interval. For example, Everitt et al. noted disease progression (TNM stage) in 11 out of 28 (39%) patients with NSCLC at a median of 24 days between the diagnostic positron emission tomography (PET)/computed tomography (CT) scan and radiation treatment planning PET/CT scan. Similarly, Geiger et al. noted new nodal or metastatic disease in 24 out of 47 (51%) stage II–III NSCLC patients at a median interscan interval of 45 days (3,4). Timely diagnosis, staging, and initiation of CRT for locally advanced NSCLC are critical, as a longer delay in CRT initiation can lead to a higher-risk of pretreatment disease progression (3,4).
The risk of rapid progression of disease with NSCLC has piqued an interest in better characterizing the prognostic significance of pretreatment growth rate though measurements like tumor doubling time and specific growth rate (SGR). The ability to predict which patients may be more likely to experience disease progression could allow clinicians to tailor the treatment timetable and therapies accordingly. Tumor doubling time has been used as a surrogate to measure growth rate. Two early Japanese trials demonstrated the independent prognostic value of tumor doubling time for stage I–IV NSCLC patients (5,6). Most patients in these trials were treated with surgery, not definitive CRT, and tumor volumes were estimated from bi-dimensional measurements on chest radiographs instead of from CT scans.
Mehrara et al. suggested that SGR, a percentage increase in volume per unit time, represents a more relevant parameter than tumor doubling time as it appears less affected by uncertainties in measurements of tumor volume and the presence of short time intervals (7,8). These investigators found that doubling time largely overestimated the rate of slowly growing tumors and underestimated the rate of rapidly growing tumors, while SGR more uniformly estimated the difference between growth rates throughout all ranges while also remaining more biologically relevant in interpreting heterogeneities within the tumor tissue (7). For early stage NSCLC treated with stereotactic body radiation therapy (SBRT), Atallah et al. found that higher pretreatment SGR correlated with a poorer overall survival (OS) and failure-free survival (9). However, it remains unclear whether pretreatment SGR correlates with clinical outcomes after CRT for locally advanced NSCLC. If SGR shows predictive value, then it could serve as a pretreatment risk stratification factor. We hypothesized that pretreatment SGR would predict for survival and recurrence rates for locally advanced NSCLC patients treated with definitive CRT.
Methods
Patient selection
Using an institutional review board-approved protocol, we retrospectively reviewed our institutional database to identify patients with locally advanced, unresectable NSCLC treated with definitive concurrent CRT from November 2009 to February 2015. We identified a total of 89 patient records for review. All patients underwent a full staging work-up, which included PET/CT scan, brain magnetic resonance imaging and mediastinal lymph node sampling. Clinical staging was based on the American Joint Committee on Cancer 7th edition criteria. Additionally, all patients received at least one diagnostic chest CT scan >14 days before the initiation of radiation therapy (RT). In cases of multiple available pretreatment scans, we used the earliest scan showing disease as the diagnostic CT scan. We excluded patients who received any type of anticancer treatment between the diagnostic CT and planning CT scans (i.e., induction chemotherapy), those with significant atelectasis, and a few whose diagnostic chest CT scans were either unavailable or could not be fused with the planning CT scans. This left 42 patients for inclusion in the study.
CRT
RT was administered over 5.5 to 7.5 weeks using daily fractions of 180–200 cGy for a median total dose of 6,120 cGy (range, 5,200–7,400 cGy). Additional details on RT planning at our institution are described in a previous publication (10). The typical chemotherapy regimen consisted of intravenous infusional drug delivery with either paclitaxel (45 mg/m2 per week) or carboplatin (area under the curve 2 per week) or cisplatin (50 mg/m2 on days 1, 8, 29 and 36) and etoposide (50 mg/m2 on days 1–5 and 29–33).
Follow-up
Patients typically followed up with the radiation and medical oncologists every 1–3 months for the first year after CRT, every 3–6 months for the next two years, and every 6–12 months afterwards. Follow-up CT scans were obtained 6–8 weeks after completion of CRT, every 3–4 months for the first year, and every 6–12 months thereafter. The CT scans were evaluated by thoracic radiologists and further reviewed by medical and radiation oncologists. Two study investigators independently reviewed follow-up notes and imaging reports to score recurrences as locoregional and/or distant.
Tumor volume and SGR calculations
We imported each patient’s diagnostic CT scan into the treatment planning system (Eclipse Treatment Planning, Varian Medical Systems, Palo Alto, California, USA) and fused it with the radiation planning CT scan. One study investigator manually contoured the primary gross tumor volume (GTV) on both the diagnostic and planning CT scans. To ensure consistency and reproducibility, we used lung window settings for all images, and 2 additional study investigators reviewed all contours.
We calculated tumor SGR (%/day) using the following equation, where GTV1 derives from the diagnostic CT scan, GTV2 derives from the radiation planning CT scan, and t is the time in days between the two scans (7):
Study endpoints
The primary endpoint was progression-free survival (PFS), with secondary endpoints of OS, time to locoregional recurrence and time to distant recurrence. We measured all endpoints from the start of RT to the event of interest or last known follow-up.
Statistical methods
We stratified patients into two groups: those with SGR ≥ upper tercile value (high SGR), and those with SGR < upper tercile (low SGR). We first compared baseline characteristics between the two partitioned groups and summarized categorical variables using frequencies and percentages. We summarized continuous variables using mean and standard deviation, or using median and range, depending on normality of distribution. We assessed differences in categorical variables between the two groups with the Chi square or Fisher exact test. We assessed differences in continuous variables between the two groups with an independent two-sample t-test or Wilcoxon rank-sum test.
To investigate the association between tumor SGR and time-to-event outcomes (PFS, OS, time to locoregional recurrence, and time to distant recurrence), we used univariate Cox regression models, with SGR stratified by the upper tercile value. We also assessed the effect of certain baseline characteristics (age, gender, histology, N stage, and primary GTV) on PFS through univariate analysis. Covariates with the lowest P values in the univariate setting were considered for inclusion in the multivariate models for PFS. We reported hazard ratios (HR) and 95% confidence intervals (CI). We assessed differences in OS, PFS, freedom from locoregional recurrence, and freedom from distant recurrence among SGR strata using the Kaplan-Meier method and log-rank test. All hypothesis tests were two-sided, and we defined P<0.05 as statistically significant for the purpose of identifying a signal of interest. All analyses were performed using SAS 9.4 (Cary, NC, USA).
Results
Table 1 displays baseline patient and tumor characteristics. The median SGR was 0.61%/day (range, –0.32%/day to 3.14%/day), and the upper tercile value was 0.94%/day. The median time from diagnostic CT scan to radiation planning CT scan was 45 days (range, 15.0–246.0 days). The median primary GTV from the diagnostic scan was 56.6 cm3 and the median primary GTV from the radiation planning scan was 74.5 cm3, representing a significant difference (P<0.0001, Wilcoxon signed-rank test). Apart from SGR, there were no significant differences in baseline characteristics between the high and low SGR groups. Median follow-up was 47.5 months for the high SGR group and 42.5 months for the low SGR group. Figure 1 shows a pair of contoured primary GTVs from the diagnostic and planning CT scans for a patient in the high SGR group, as well as a pair for a patient in the low SGR group.
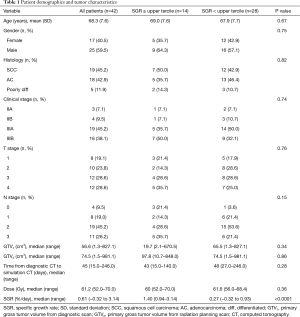
Full table
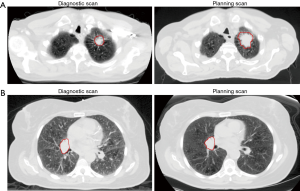
PFS
Median PFS for the cohort was 9.8 months (95% CI: 7.4–14.0 months). PFS was significantly worse for the high versus low SGR group (median PFS, 5.6 vs. 13.6 months, P=0.0163). Figure 2 depicts Kaplan-Meier PFS curves for each group. On univariate analysis, SGR ≥ upper tercile was associated with inferior PFS (HR 2.39, 95% CI: 1.15–4.97, P=0.020). On multivariate analysis, after adjusting for age, N stage, and primary GTV from the radiation treatment-planning CT scan, SGR ≥ upper tercile remained associated with inferior PFS (adjusted HR 2.37, 95% CI: 1.07–5.25, P=0.034) (Table 2).
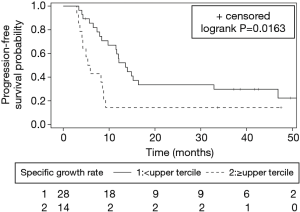
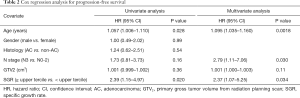
Full table
OS
Median OS for the cohort was 19.0 months (95% CI: 13.8–40.8 months). Median OS was 14.6 months for the high SGR group compared to 32.9 months for the low SGR group, but this difference did not reach statistical significance (P=0.1799). Figure 3 depicts Kaplan-Meier survival curves for each group. The risk of death was higher, but not significantly so, in the high SGR group (HR 1.69, 95% CI: 0.78–3.55, P=0.18).
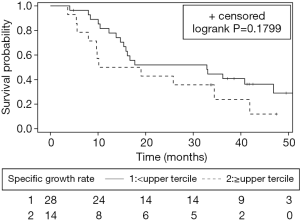
Locoregional and distant recurrence
To understand why PFS was lower in the high SGR group, we analyzed locoregional and distant recurrences. Locoregional recurrence occurred in 14 patients. There was no difference in the risk of locoregional recurrence between the high and low SGR groups (HR 1.16, 95% CI: 0.36–3.71, P=0.80). Three-year freedom from locoregional recurrence was 62.3% versus 59.0%, respectively (P=0.8033).
Distant recurrence occurred in 20 patients. The risk of distant recurrence was significantly higher for the high versus low SGR group (HR 2.62, 95% CI: 1.08–6.38, P=0.033). Three-year freedom from distant recurrence was 31.1% versus 55.3%, respectively (P=0.0270). Figure 4 depicts Kaplan-Meier curves for freedom from locoregional recurrence and distant recurrence.
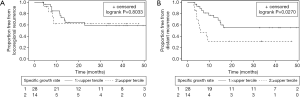
Discussion
This study suggests that a higher pretreatment tumor growth rate, as measured by SGR, is associated with poorer PFS and an increased risk of distant recurrence for patients with locally advanced NSCLC receiving definitive CRT. To our knowledge, this is the first study to report on the possible prognostic value of SGR for patients with locally advanced NSCLC.
Among patients with early stage NSCLC treated with SBRT, Atallah et al. found that SGR ≥ median correlated with both poorer OS and failure-free survival (9). The median SGR in their study was 0.43%/day, slightly lower than the median SGR in our study of 0.61%/day. One notable difference is that they found a significant increase in regional failures and a slight increase in local failures in the high SGR group, whereas we found an increase in distant recurrences but no difference in locoregional recurrences in the high SGR group.
The differences between our results and those of Atallah et al. may reflect the differences in disease stage between our patient populations, locally advanced in our study versus early stage NSCLC in the Atallah et al. study, so our study is novel in this regard. Distant metastasis is the predominant pattern of failure in locally advanced NSCLC, occurring in >50% of patients (1). The aforementioned study by Geiger et al. included patients with locally advanced NSCLC and found new nodal or metastatic disease in 51% of patients (4). In our cohort, 20 out of 42 (47.6%) patients experienced distant recurrence, whereas 14 out of 42 (33.3%) patients experienced locoregional recurrence. Thus, growth of the primary tumor and involved lymph nodes may either correlate with or indicate seeding of distant metastasis (11). For early stage NSCLC, distant metastasis typically occurs in fewer patients (≤20%) (12); consequently, the growth of the primary tumor is potentially more predictive of locoregional failure.
Our study is not without limitations. First, our study was retrospective and performed at a single institution, with a limited sample size of 42 patients. However, these characteristics apply to two studies by Jabbour et al. and Wald et al., which included 38 and 52 patients, respectively. Both assessed the prognostic value of reduction in tumor volume seen on daily cone beam CT imaging during CRT for locally advanced NSCLC (10,13), and have important implications for treatment intensification strategies, similar to our study. Through this study, we add to the existing literature of prognostic factors for patients with locally advanced NSCLC and provide clinical value in that a higher pretreatment SGR is associated with a decreased PFS and an increased chance of distant recurrence in patients receiving this therapeutic regimen. Second, we excluded patients for a variety of reasons, which might arguably introduce selection bias. However, the most common reason for exclusion was if a patient received induction chemotherapy, which is not considered the standard of care for locally advanced NSCLC and does not change survival. We excluded these patients since induction chemotherapy is a potential confounder. We also excluded patients with significant atelectasis, as well as a few of those whose diagnostic scans were either unavailable or could not be fused with the planning scans, similar to Wald et al. which excluded patients for technical reasons such as poor image quality and unreliable tumor volume contours (13).
In light of these limitations, and because we chose to dichotomize patients by the upper tercile SGR value, we consider our findings hypothesis generating, and plan to further elucidate the prognostic value of SGR in patients with locally advanced NSCLC. We acknowledge that an SGR of 0.94%/day (the upper tercile value in our cohort) is not necessarily the ideal cut-point for prognostication. Future studies with larger sample sizes are needed to gain a clearer understanding of the relationship between SGR and clinical outcomes for patients with locally advanced NSCLC. However, we are progressing toward a better understanding of growth rates of NSCLC and provide additional insight into this sizeable subset of NSCLC patients with locally advanced, unresectable disease.
If validated as a prognosticator, SGR could aid in identifying a patient population more likely to benefit from a more aggressive therapy. For example, the PACIFIC trial recently found that the addition of consolidation durvalumab after concurrent CRT for stage III NSCLC improved 2-year OS by 10.7% and median PFS by 11.6 months (14). However, it remains unclear whether earlier integration of immunotherapy into CRT regimens can further improve outcomes, and which patients stand to benefit the most (15).
Additionally, the growth rates that were calculated demonstrate the importance of prompt initiation of therapy, particularly CRT. From the diagnostic to radiation treatment-planning CT scan (median, 45 days), the median primary GTV among all patients increased by almost 20 cm3. This increase was particularly prominent for those with SGR ≥ upper tercile, as the median primary GTV for this group increased from 19.7 to 97.8 cm3 between the two scans. Comparing the residual tumor volume visualized on follow-up imaging to the tumor volume on diagnostic imaging alone will underestimate the efficacy of radiation treatment since the interim growth between the diagnostic and planning scans may be sizeable.
Delays in the initiation of concurrent CRT were multifactorial. Prolonged intervals in imaging were not necessarily due to delays among oncologists, but often patients experienced delays in initial diagnosis (e.g., delay in lung cancer biopsy or transfer of care from primary care physician to pulmonologist), necessitating repeating a chest CT scan to ascertain the baseline status of disease. Furthermore, induction chemotherapy is not considered the standard of care for this group of patients, so chemoradiation was initiated as soon as possible.
In conclusion, a higher SGR correlated with an inferior PFS and an increased risk of distant recurrence for patients with locally advanced NSCLC receiving concurrent CRT. Supplementary studies assessing SGR as a potential prognosticator for this patient population appear warranted.
Acknowledgements
Funding: Services, results and/or products in support of the research project were generated by the Rutgers Cancer Institute of New Jersey Biometrics Shared Resource, supported, in part, with funding from NCI-CCSG P30CA072720.
Footnote
Conflicts of Interest: Dr. Jabbour has received research funding from Merck and Nestle. Dr. Malhotra is on the advisory board of Astra-Zeneca and Pfizer and has received research funding from Oncoceutics. The other authors have no conflicts of interest to declare.
Ethical Statement: Lung cancer IRB (Pro20170001559) approved by Rutgers University, New Brunswick Health Sciences IRB.
References
- Bradley JD, Paulus R, Komaki R, et al. Standard-dose versus high-dose conformal radiotherapy with concurrent and consolidation carboplatin plus paclitaxel with or without cetuximab for patients with stage IIIA or IIIB non-small-cell lung cancer (RTOG 0617): a randomised, two-by-two factorial phase 3 study. Lancet Oncol 2015;16:187-99. [Crossref] [PubMed]
- Curran WJ Jr, Paulus R, Langer CJ, et al. Sequential vs. concurrent chemoradiation for stage III non-small cell lung cancer: randomized phase III trial RTOG 9410. J Natl Cancer Inst 2011;103:1452-60. [Crossref] [PubMed]
- Everitt S, Herschtal A, Callahan J, et al. High rates of tumor growth and disease progression detected on serial pretreatment fluorodeoxyglucose-positron emission tomography/computed tomography scans in radical radiotherapy candidates with nonsmall cell lung cancer. Cancer 2010;116:5030-7. [Crossref] [PubMed]
- Geiger GA, Kim MB, Xanthopoulos EP, et al. Stage migration in planning PET/CT scans in patients due to receive radiotherapy for non-small-cell lung cancer. Clin Lung Cancer 2014;15:79-85. [Crossref] [PubMed]
- Arai T, Kuroishi T, Saito Y, et al. Tumor doubling time and prognosis in lung cancer patients: evaluation from chest films and clinical follow-up study. Japanese Lung Cancer Screening Research Group. Jpn J Clin Oncol 1994;24:199-204. [PubMed]
- Usuda K, Saito Y, Sagawa M, et al. Tumor doubling time and prognostic assessment of patients with primary lung cancer. Cancer 1994;74:2239-44. [Crossref] [PubMed]
- Mehrara E, Forssell-Aronsson E, Ahlman H, et al. Specific growth rate versus doubling time for quantitative characterization of tumor growth rate. Cancer Res 2007;67:3970-5. [Crossref] [PubMed]
- Mehrara E, Forssell-Aronsson E, Ahlman H, et al. Quantitative analysis of tumor growth rate and changes in tumor marker level: specific growth rate versus doubling time. Acta Oncol 2009;48:591-7. [Crossref] [PubMed]
- Atallah S, Cho BC, Allibhai Z, et al. Impact of pretreatment tumor growth rate on outcome of early-stage lung cancer treated with stereotactic body radiation therapy. Int J Radiat Oncol Biol Phys 2014;89:532-8. [Crossref] [PubMed]
- Jabbour SK, Kim S, Haider SA, et al. Reduction in Tumor Volume by Cone Beam Computed Tomography Predicts Overall Survival in Non-Small Cell Lung Cancer Treated With Chemoradiation Therapy. Int J Radiat Oncol Biol Phys 2015;92:627-33. [Crossref] [PubMed]
- Prenzel KL, Monig SP, Sinning JM, et al. Lymph node size and metastatic infiltration in non-small cell lung cancer. Chest 2003;123:463-7. [Crossref] [PubMed]
- Chi A, Liao Z, Nguyen NP, et al. Systemic review of the patterns of failure following stereotactic body radiation therapy in early-stage non-small-cell lung cancer: clinical implications. Radiother Oncol 2010;94:1-11. [Crossref] [PubMed]
- Wald P, Mo X, Barney C, et al. Prognostic Value of Primary Tumor Volume Changes on kV-CBCT during Definitive Chemoradiotherapy for Stage III Non-Small Cell Lung Cancer. J Thorac Oncol 2017;12:1779-87. [Crossref] [PubMed]
- Antonia SJ, Villegas A, Daniel D, et al. Overall Survival with Durvalumab after Chemoradiotherapy in Stage III NSCLC. N Engl J Med 2018;379:2342-50. [Crossref] [PubMed]
- Jabbour SK, Berman AT, Simone CB. Integrating immunotherapy into chemoradiation regimens for medically inoperable locally advanced non-small cell lung cancer. Transl Lung Cancer Res 2017;6:113-8. [Crossref] [PubMed]