A deeper understanding of the tumor microenvironment in pancreatic cancer: the key to developing effective immunotherapies
Dismal statistics in pancreatic ductal adenocarcinoma (PDAC)
The number of pancreatic cancer related deaths is projected to increase while many other cancers fall (1). Pancreatic cancer is the third leading cause of cancer related death and projected to overtake second by 2020 (1). Despite intense research efforts over many years, five-year survival after diagnosis with pancreatic cancer remains grim at around 8% (2). Only 20% of patients present with surgically resectable disease, and even for those, five-year survival is a mere 24.6% (3). For those with more advanced disease, median survival is just 4.2 months (3). Of the small minority of patients who present with resectable disease, standard of care includes surgical resection followed by a limited number of chemotherapeutic options which yield a 19-month median survival (3). Response to available chemotherapeutic regimens varies between patients and the reasons behind these varied responses are unclear. This leave clinicians with a strategy of administering a chemotherapy and abandoning it in lieu of another after disease progression. Regardless of therapeutic approach, and particularly in metastatic disease, the prognosis remains poor (4). There has been little improvement in these dismal statistics despite decades of research.
Immunotherapy in cancer treatment
Immunotherapy for cancer treatment has gained much interest in recent years. Outside the classic treatment with surgery, chemotherapy, and radiation therapy, immunotherapy offers another mechanism for fighting cancer. The National Cancer Institute (NCI) defines immunotherapy as “A type of therapy that uses substances to stimulate or suppress the immune system to help the body fight cancer, infection, and other diseases.” This can include monoclonal antibodies, vaccines, checkpoint inhibitors, and adoptive cell transfer. The past decade has seen fervent investigation into the relationship between the immune system and cancer. Targeting these pathways provides novel mechanisms for cancer therapies. The 2018 Nobel Prize in Physiology or Medicine was jointly awarded to James P. Allison and Tasuku Honjo for their efforts in checkpoint inhibition. The year of 2018 alone saw Food and Drug Administration (FDA) approval of immunotherapy in hepatocellular carcinoma, small cell lung cancer, colorectal cancer, lymphoma, and cervical cancer. Some of these efforts have yielded stunning outcomes with long-term survival in patients with previously poor prognoses. The results of a phase 1 trial on the use of recombinant poliovirus in the treatment of recurrent glioblastoma was well publicized in the mainstream media and demonstrated an increase from 4% to 21% in three-year survival in those treated with the recombinant poliovirus (5). While these results are promising, the typical treatment response of the FDA approved immunotherapies is subtler and leave much work to be done.
Current efforts in pancreatic cancer immunotherapy
The only FDA approved immunotherapy for patients diagnosed with pancreatic cancer is pembrolizumab, a programmed cell death protein 1 (PD-1) inhibitor. It is only available to patients with a mismatch repair deficiency, which represents less than 1% of patients with PDAC (6). Other available immunotherapies have been tested and failed to demonstrate any appreciable improvement in outcomes. A phase I trial of an anti-programmed death-ligand 1 (anti-PD-L1) antibody in multiple advanced cancers failed to demonstrate a benefit in pancreatic cancer (7). A phase II trial of ipilimumab, a monoclonal antibody that targets cytotoxic T-lymphocyte–associated antigen 4 (CTLA-4) and is used in the treatment of melanoma, demonstrated it is ineffective in pancreatic cancer (8).
Other immunotherapies have been explored and also failed to provide meaningful benefit. GVAX is a cancer vaccine composed of granulocyte-macrophage colony stimulating factor (GM-CSF) secreting whole tumor cells. A phase II trial of GVAX demonstrated no benefit over historical controls (9). Combination therapies have gained enthusiasm in pancreatic cancer. Attacking multiple defense strategies of pancreatic cancer simultaneously may prove to be the most efficacious. GVAX and checkpoint inhibitors in combination have shown early promise in preclinical models but further investigation is needed (10). The lack of effective immunotherapies demand investigation of novel pathways within the unique pancreatic cancer tumor microenvironment.
PDAC and the tumor microenvironment
The mechanisms behind the poor response of PDAC to conventionally used immunotherapies are not well understood. PDAC is known to be a poorly immunogenic tumor. This is thought to be, in part, due to the lower neoantigenic profile of pancreatic cancer cells and immunosuppressive nature of the tumor microenvironment. The complex relationship between pancreatic cancer cells and the surrounding desmoplastic stroma is the subject of considerable investigation. This tumor-associated stroma (TAS) is composed of fibroblasts, extracellular matrix, and immune cells and represents as much as 80% of tumor volume in PDAC (11). It is thought to provide a protective barrier to cancer cells and contribute to cell growth and chemoresistance (12). Depletion of the TAS should then contribute to improved ability of the immune system to recognize and fight neoantigens. Contradictory to this, depletion of the stroma accelerates pancreatic cancer growth and is associated with worse survival (13). While it has become clear that the relationship between cells in the PDAC tumor microenvironment is quite complex, efforts are focused on understanding this relationship. The CAFs and pancreatic cancer cells use a variety of mechanisms to communicate and evade immune defenses. A number of mechanisms under current investigation are summarized in Table 1.
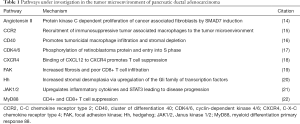
Full table
βig-h3 as a potential target for immunotherapy
In their recent paper in Gut, Goehrig et al. describe the impact of the stromal protein βig-h3 in pancreatic cancer (23). They make a compelling case for the importance of βig-h3 in immune tolerance to pancreatic cancer in a murine model and in culture. First, they demonstrate that βig-h3 is strongly expressed in Cre;KrasG12D;Ink4a/Arffl/fl (KIC) and pdx1-Cre;KrasG12D;p53R172H (KPC) mice compared to control, as well as human pancreatic cancer biopsies. βig-h3 is secreted primarily by the cancer-associated fibroblasts (CAF). The effects of βig-h3 within the tumor microenvironment highlight its potential as a target for future therapies. βig-h3 suppresses proliferation of antitumoral CD8+ T cells in vivo and in vitro, controls activation of macrophages and CD8+ T cells, and promotes M2 macrophage differentiation. Depletion of βig-h3 in vivo led to a decrease in neoplastic cells and reduced tumor volume. Further analysis of the tumors from Big-h3 depleted mice demonstrated more infiltrating CD8+ T cells with a less exhausted phenotype. The summation of these findings suggests that βig-h3 is a critical protein secreted by CAFs and involved in tumorigenesis as well as promoting an immunosuppressive tumor microenvironment through modulation of both the adaptive and innate immune systems.
βig-h3 represents an important target for future immunotherapies and the authors should be commended for their work. Blocking βig-h3 may help improve immune clearance of neoplastic cells in future therapies. A deeper understanding of the mechanisms through which this protein acts to promote an immunosuppressed tumor microenvironment may identify other biologic targets of similar importance.
Conclusions
PDAC presents many unique challenges for development of effective immunotherapies when compared to other more immunogenic cancers. Investigators have described many mechanisms of immune evasion by PDAC and our understanding of this phenomenon continues to grow. Continued work to identify and attack these mechanisms of immune evasion and tumor progression may provide the breakthrough needed to help the immune system fight this deadly malignancy.
Acknowledgements
None.
Footnote
Conflicts of Interest: The authors have no conflicts of interest to declare.
References
- Rahib L, Smith BD, Aizenberg R, et al. Projecting cancer incidence and deaths to 2030: the unexpected burden of thyroid, liver, and pancreas cancers in the United States. Cancer Res 2014;74:2913-21. [Crossref] [PubMed]
- Siegel RL, Miller KD, Jemal A. Cancer statistics, 2018. CA Cancer J Clin 2018;68:7-30. [Crossref] [PubMed]
- Bilimoria KY, Bentrem DJ, Ko CY, et al. National failure to operate on early stage pancreatic cancer. Ann Surg 2007;246:173-80. [Crossref] [PubMed]
- Conroy T, Desseigne F, Ychou M, et al. FOLFIRINOX versus gemcitabine for metastatic pancreatic cancer. N Engl J Med 2011;364:1817-25. [Crossref] [PubMed]
- Desjardins A, Gromeier M, Herndon JE 2nd, et al. Recurrent Glioblastoma Treated with Recombinant Poliovirus. N Engl J Med 2018;379:150-61. [Crossref] [PubMed]
- Hu ZI, Shia J, Stadler ZK, et al. Evaluating Mismatch Repair Deficiency in Pancreatic Adenocarcinoma: Challenges and Recommendations. Clin Cancer Res 2018;24:1326-36. [Crossref] [PubMed]
- Brahmer JR, Tykodi SS, Chow LQ, et al. Safety and activity of anti-PD-L1 antibody in patients with advanced cancer. N Engl J Med 2012;366:2455-65. [Crossref] [PubMed]
- Royal RE, Levy C, Turner K, et al. Phase 2 trial of single agent Ipilimumab (anti-CTLA-4) for locally advanced or metastatic pancreatic adenocarcinoma. J Immunother 2010;33:828-33. [Crossref] [PubMed]
- Lutz E, Yeo CJ, Lillemoe KD, et al. A lethally irradiated allogeneic granulocyte-macrophage colony stimulating factor-secreting tumor vaccine for pancreatic adenocarcinoma. A Phase II trial of safety, efficacy, and immune activation. Ann Surg 2011;253:328-35. [Crossref] [PubMed]
- Soares KC, Rucki AA, Wu AA, et al. PD-1/PD-L1 blockade together with vaccine therapy facilitates effector T-cell infiltration into pancreatic tumors. J Immunother 2015;38:1-11. [Crossref] [PubMed]
- Chu GC, Kimmelman AC, Hezel AF, et al. Stromal biology of pancreatic cancer. J Cell Biochem 2007;101:887-907. [Crossref] [PubMed]
- Delitto D, Wallet SM, Hughes SJ. Targeting tumor tolerance: A new hope for pancreatic cancer therapy? Pharmacol Ther 2016;166:9-29. [Crossref] [PubMed]
- Özdemir BC, Pentcheva-Hoang T, Carstens JL, et al. Depletion of carcinoma-associated fibroblasts and fibrosis induces immunosuppression and accelerates pancreas cancer with reduced survival. Cancer Cell 2014;25:719-34. [Crossref] [PubMed]
- Hama K, Ohnishi H, Aoki H, et al. Angiotensin II promotes the proliferation of activated pancreatic stellate cells by Smad7 induction through a protein kinase C pathway. Biochem Biophys Res Commun 2006;340:742-50. [Crossref] [PubMed]
- Nywening TM, Wang-Gillam A, Sanford DE, et al. Targeting tumour-associated macrophages with CCR2 inhibition in combination with FOLFIRINOX in patients with borderline resectable and locally advanced pancreatic cancer: a single-centre, open-label, dose-finding, non-randomised, phase 1b trial. Lancet Oncol 2016;17:651-62. [Crossref] [PubMed]
- Beatty GL, Torigian DA, Chiorean EG, et al. A phase I study of an agonist CD40 monoclonal antibody (CP-870,893) in combination with gemcitabine in patients with advanced pancreatic ductal adenocarcinoma. Clin Cancer Res 2013;19:6286-95. [Crossref] [PubMed]
- Witkiewicz AK, Borja NA, Franco J, et al. Selective impact of CDK4/6 suppression on patient-derived models of pancreatic cancer. Oncotarget 2015;6:15788-801. [Crossref] [PubMed]
- Feig C, Jones JO, Kraman M, et al. Targeting CXCL12 from FAP-expressing carcinoma-associated fibroblasts synergizes with anti-PD-L1 immunotherapy in pancreatic cancer. Proc Natl Acad Sci U S A 2013;110:20212-7. [Crossref] [PubMed]
- Jiang H, Hegde S, Knolhoff BL, et al. Targeting focal adhesion kinase renders pancreatic cancers responsive to checkpoint immunotherapy. Nat Med 2016;22:851-60. [Crossref] [PubMed]
- Olive KP, Jacobetz MA, Davidson CJ, et al. Inhibition of Hedgehog signaling enhances delivery of chemotherapy in a mouse model of pancreatic cancer. Science 2009;324:1457-61. [Crossref] [PubMed]
- Hurwitz HI, Uppal N, Wagner SA, et al. Randomized, Double-Blind, Phase II Study of Ruxolitinib or Placebo in Combination With Capecitabine in Patients With Metastatic Pancreatic Cancer for Whom Therapy With Gemcitabine Has Failed. J Clin Oncol 2015;33:4039-47. [Crossref] [PubMed]
- Delitto D, Delitto AE, DiVita BB, et al. Human Pancreatic Cancer Cells Induce a MyD88-Dependent Stromal Response to Promote a Tumor-Tolerant Immune Microenvironment. Cancer Res 2017;77:672-83. [Crossref] [PubMed]
- Goehrig D, Nigri J, Samain R, et al. Stromal protein betaig-h3 reprogrammes tumour microenvironment in pancreatic cancer. Gut 2019;68:693-707. [Crossref] [PubMed]