Preclinical models of Wilson’s disease, why dogs are catchy alternatives
Introduction
Initiated by the discovery of the causative gene for Wilson disease (WD), the copper transporter ATPase2 (ATP7B), in 1993 various model animals have been developed and/or discovered by accident, that can be used to study specific aspects of WD (1,2). ATP7B is mainly expressed in the liver, although other organs such as the brain, kidney, placenta and, as recently described, parts of the small intestine also express the ATP7B protein. Interestingly the function varies between the different organs (3). The experimental animals for WD express either one or two copper transporters, with Caenorhabditis elegans and Drosophila melanogaster (only one ATP7A/B orthologue), whereas Xenopus laevis, Dario rerio and rodent models express both ATP7A and ATP7B genes (4). The most frequently studied rodent models are the “Toxic milk” mouse with a mutation in the ATP7B gene, a variant of this mouse TX-J mice with a different mutation in the ATP7B gene, and the ATP7B knockout (ATP7B-/-) mouse developed in 1999 (5). The Long-Evans Cinnamon (LEC) rat is also positioned as a relevant rodent model due to a 900 bp deletion within the 3’-end of the ATP7B gene (6). Copper secretion in LEC rats was restored after adenovirus mediated ATP7B expression (7).
These rodent models have been instrumental to describe in great detail the progression of the disease and molecular mechanism of action of the ATP7B protein. These genetically highly standardized experimental animal does not reflect the large number of mutations in the ATP7B gene (www.hgmd.cf.ac.uk/ac/index.php). In view of the variable clinical presentation and age of onset of Wilson’s disease, the predictable value of the ATP7b −/− mice or LEC rats for most of the WD-patients is suboptimal (8,9). Moreover, the size and longevity of mice is an order of magnitude different from men. Size differences between rodents and men do not always allow to perform surgical strategies that might be relevant for human patients, longevity issues hamper the analysis of long-term effects of certain treatment options.
Some of these drawbacks can be tackled by using dogs (Canis lupus familiaris) as preclinical animal model for WD. By definition a preclinical study is a study to test a drug, a procedure, or another medical treatment in animals. The aim of a preclinical study is to collect data in support of the safety of the new treatment. Preclinical studies are required before clinical trials in humans can be started. This means that a model, irrespective being fundamental or preclinical by nature, does not need to be identical to the human disease.
What makes dogs, especially for WD, a rare disease in men, so well-suited? In order to fully appreciate the potential of these animals some insights into canine breeding and genetics is needed. Following the domestication of dogs, the timing of which is however still a matter of great dispute, these animals have been exposed to severe artificial selection for specific traits through breeding strategies. The end-result of this resulted in fact in isolated genetic populations of dog breeds (10). Together with the selection for a unique trait, such as excessive muscle formation, short limbs or a specific coat color, an increased risk for the development of specific disorders with a simple and/or complex inheritance pattern arose within breeds. Whereas the genetic variation over the various breeds remained intact, the reduced genetic variability within breeds works as a genetic dissection microscope (11). Exploiting the downside of inbreeding may therefore be instrumental for the discovery causative and modifier genes involved in complex diseases such as WD. In other words, it may very well lead to an improved genotype-phenotype correlation (9).
The comparable size and the similar environmental exposure of men and dogs is a no-brainer. Especially the size allows to design and test procedures at a humanized size, which could for instance be crucial for transplantation studies, as the readers might be aware that the first liver transplantations were performed in dogs (12).
In summary both genetic and technical arguments are in favor to utilize dogs as important preclinical models for WD. This review describes in more detail the recent work on two dog breeds, Bedlington terrier (simple genetics) and Labrador retriever (complex genetics), for which causative mutations involved in hepatic copper toxicosis are described and weighs the pros and cons of both breeds regarding liver stem cell transplantation (preclinical study to test a procedure) and long-term safety effects of a novel copper chelating compound.
Copper accumulation in Bedlington terriers is caused by a Mendelian inherited mutation in the COMMD-gene
Veterinarians were aware of copper disorders in sheep and dogs for decades (13,14). The increased levels of hepatic copper are presented in a number of dog breeds including Bedlington terriers, Skye terriers, West-Highland White terriers, Doberman, Dalmatians and Labrador retrievers (15-20). Pedigree analysis revealed a complex form of copper-mediated hepatitis, viz the phenotypic expression not only depends on genetic factors but also on environmental factors, in most breeds except the Bedlington terrier.
In 1999 genetic mapping studies revealed that the copper toxicosis locus win Bedlington terriers was located on chromosome 10 region 2p26 (21). Positional cloning identified, three years later, a 13 kB deletion covering exon-2 of the murr1 gene as the causative mutation of Bedlington terrier copper toxicosis (22). Soon after this discovery this mutation was analysed in other copper storage diseases, it turned out that neither in Indian Childhood Cirrhosis (ICC), Endemic Tyrolean Infantile Cirrhosis (ETIC), or Idiopathic copper toxicosis (ICT) this mutation was causative (23). The role of murr1 mutations in WD is a matter of debate (24-26). This gene product, currently called COMMD1, copper metabolism murr1 domain-containing protein 1, had an unknown function at the time it was discovered. Nowadays a plethora of functions are related to the COMMD1 protein, including sodium transport trafficking of cystic fibrosis transmembrane conductance regulator (CFTR), inhibition of Cu/Zn-SOD, NF-κB signalling, hypoxia inducing factor (HIF1) regulation and HIV-replication (27-30). Direct evidence for a crucial role in cellular copper regulation obtained after siRNA-mediated commd1 silencing in human embryonic kidney (HEK-293) cells and more resembling liver pathology in BDE-cells, a canine hepatocyte-like cell line (31,32). Finally, liver specific ATP7B deficient mice had increased hepatic copper levels (33). With respect to hepatic copper accumulation it turned out that COMMD1 protein functions as a chaperone for ATP7B, and later on also ATP7A was shown to be guided by COMMD1 within the hepatocyte (34-36). The fetal lethality of COMMD1 −/− mice, possibly caused by defect in the placenta development exemplifies the necessity for non-mice models (33).
Longitudinal study on COMMD1 deficient dogs depict in detail the molecular and cellular changes during copper-induced progression towards chronic hepatitis
In order to investigate the development of liver pathology in time, an in-house breeding colony of five COMMD1 deficient dogs was followed for over 4 years. Although these animals are not geno-copies of human WD patients and their hepatic copper levels exceed 10,000 mg copper per kg dry weight liver (dwl), neurological features are absent as are Kayser-Fleischer rings, their size allows for a true longitudinal study permitting liver biopsy sampling twice a year. Per time point several biopsies were taken for histology, copper measurements, immunohistochemistry, quantitative RT-PCR and Western blotting (37-39) (Figure 1). Maximum copper accumulation was reached at 12 months of age, which coincided with the first signs of hepatitis and increased levels of mt1A (copper scavenger metallothionein) mRNA. Hepatic stellate cells were activated (α-SMA positivity) from 18 months onwards, with increasing reticulin deposition and hepatocytic proliferation in later stages. Hepatitis and pro-apoptotic caspase-3 activity (first noticed at 18 months) increased over time. Both Hepatocyte Growth Factor and TGF-β1 gene expression peaked at 24 months, and decreased gradually at later time points. Both STAT3 and c-MET proteins showed an increased time-dependent activation. Smad2/3 phosphorylation, indicative for fibrogenesis, was present at all time-points. Interestingly a transient increase in atox1 (antioxidant 1 copper chaperone), ccs (copper chaperone for cytochrome C oxidase), cox17 (cyclooxygenase 17), atp7A and atp7B mRNA levels was observed, indicating attempts to cope with intracellular copper overload. To minimize the detrimental effects of reactive oxygen species, mRNA levels of sod, cat, and gpx1, were also temporarily increased. Together, thus unique longitudinal study established that COMMD1-deficient dogs develop copper-induced chronic liver disease and cirrhosis comparable to human WD patients, although at much higher pace and more extensively. This positioned this breeding colony as genetically-defined large animal model to test clinical applicability of new therapeutics developed in rodent models.
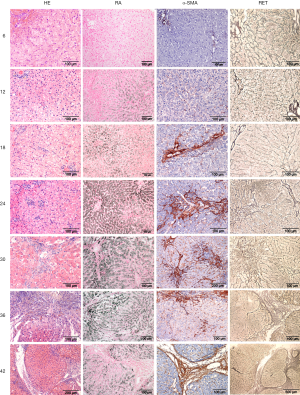
As will be outlined below, this colony was extended for preclinical studies on autologous liver stem cell transplantations.
Mutations in both ATP7A and ATP7B titrate hepatic copper levels in Labrador retrievers
Notwithstanding the advantages of the COMMD1 deficient dogs, some aspects do not mimic WD, apart from the genetic background and the excessive hepatic copper levels. This prompted researchers to dissect the genetic background of the Labrador retriever, one of the most popular dog breeds in the Western world. In this breed copper levels (600 mg copper per kg dry weight liver, rarely exceeds 5,000 mg copper per kg dry weight liver) are in the range of hepatic copper levels in WD patients. Moreover, female dogs are at a higher risk for this disease and the age of onset of hepatic cirrhosis is variable but usually in middle aged dogs (20). As for other dog breeds, Kaiser-Fleischer rings and neurological symptoms are nor presented. Early signs of hepatic damage are increased levels of alanine aminotransferase (ALT). It turned out that around one-third of the first-line relative of Labradors retrievers with copper toxicosis had elevated copper levels too (20). Finally, urine copper/zinc ratio was associated with hepatic copper levels, but the diagnostic values remains low, since its overlaps with urinary copper/zinc ratios and normal hepatic copper levels (40). This opened the question whether or not Labrador retrievers were new model animals for WD (41).
The genes involved in Labrador retriever copper toxicosis were largely unknown until recently, but the hereditary pattern clearly suggested a complex genetic disorder. Since canine specific genetic tools such as a SNP-array platform to perform a Genome Wide Association Study (GWAS) were available together with large and well-described pedigrees, one of us aimed to identify gene variants involved Labrador retriever copper toxicosis.
The GWAS included an analysis of 235 Labrador retrievers (154 female, 81 male) in the Netherlands that were genotyped on an Illumina Canine HD Bead Chip, with about 170k SNPs (42). The replication set of Labrador retrievers consisted of 37 female and 22 male dogs. These numbers shown that whereas WD is a rare disease in humans, it is fairly frequent in dogs. Homozygous and heterozygous mutations in the ATP7A gene (ATP7A:c980C>Y) and in the ATP7B gene (ATP7B:c4358G>A) were discovered in both sexes. Interestingly individuals had either one or both of the genes affected which was clearly associated with the hepatic copper levels. The ATP7A variant partially protected against copper accumulation, whereas the ATP7B mutant was associated with increased hepatic copper levels. The ATP7B:pArg1453Gln mis-localized in the endoplasmic reticulum, the ATP7A:pThr327Ile variant had delayed copper excretion, at least in dermal fibroblasts. The partial elucidation of the genetic background provided another large animal model for WD, one representing mutations in Menkes and Wilson disease causative genes, and suggested that mutations in theATP7A can act as modifier genes for WD.
Approximately 12% of the phenotype can be explained by these two mutations in this breed, mutations in genes that were already known to be involved in copper related disorders. It remains to be seen what other as yet unidentified genetic mutations are involved and whether other currently undiscovered modifiers genes and/or environmental factors will be discovered.
Transplantation of autologous gene-corrected liver organoids into copper-laden livers of dogs
Since the Pavlov dog (Nobel prize in 1904) and the discovery of (canine) insulin (Nobel prize in 1923), this species has been outnumbered by rodents as an animal models for human diseases. The discovery of the COMMD1-mutation in Bedlington terriers facilitated researchers at the Faculty of Veterinary Medicine Utrecht the Netherland to create an in-house breeding colony of homozygous COMMD1 deficient dogs. As a follow-up of the longitudinal studies with regard to the molecular and cellular description of copper induced chronic hepatitis, the idea arose to use these animals for autologous gene-corrected liver stem cells transplantation studies, a preclinical model for such like studies in men. This preclinical study was designed in close collaboration with human liver cell transplantation surgeons (Prof Fox, Pittsburgh MA, USA) and human pediatric hepatologists (Prof. Houwen, Utrecht, NL) to evaluate the most effective transplantation routing of organoid of the most optimal expansion/differentiation status. This first hurdle was the creation of large quantities of autologous liver stem cells. Unfortunately, it was a decade ago impossible to culture functionally active hepatocytes or liver progenitor cells. In a landmark paper by Huch et al. in 2013 (43), it was established that long term culture of mouse liver progenitor cells as 3D mini-organs, the so-called organoids, was feasible and despite high proliferation rates these cells were deemed genetically stable and it was possible to differentiate the progenitor cells into a hepatocyte-like phenotype. Two years later both liver organoids from men and dogs were described (44,45), see Figure 2 depicting canine liver organoids. With these innovative tools at hand, the in-house breeding colony of COMMD1-deficient dogs are currently receiving autologous and gene-corrected liver organoid transplantations. This study allows us to follow five individual dogs up to 2 years after the transplantation. The biopsies taken after transplantation indicate that the autologous and gene-corrected organoids survive in the hostile copper laden environment. Importantly there are no signs of uncontrolled proliferation of the donor cells in the recipient liver. More comprehensive data on most effective differentiation status of the transplanted cells (stem or hepatocyte-like cells), route of administration (direct injection or hepatic vein), and long-term effects are expected midway 2019. After the termination of this experiment, due to legal restrictions, the brains will be analysed by LA-ICP-MS to investigate the accumulation of metals in the brain (46). Although no neurological features are described, apart from encephalopathy, accumulation of copper in canine brains due to COMMD1 deficiency has not been proven or excluded thus far.
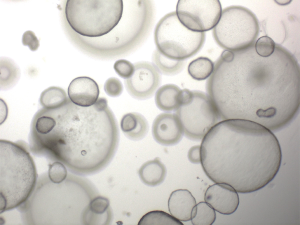
This experiment is illustrative for the potential of this species as preclinical model to test a surgical procedure in a relative standardized experimental setting.
Together, the molecular and histological description of the progress of liver cirrhosis in these COMMD1-deficient dogs provided a rationally designed and possibly highly relevant preclinical model to study the liver aspects of WD.
Pros and cons of both breeds for preclinical studies
Each model has its limitations, for instance it is a simplification or exaggeration of the reality. The beauty (and relevance) of a model is in the eye of the beholder. Yet in order to make a rational decision on which large animal to be used for various preclinical studies to benefit WD patients some aspects of both dog breeds are compared in Table 1.
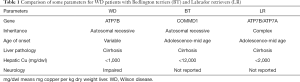
Full table
Future of novel preclinical models, a DOGmatic shift?
Recently a reference network for rare liver diseases (ERN-RARE-LIVER) was established by the European Commission. This shows the EC’s perseverance to address the issues associated with rare diseases such as their limited research resources, their lack of understanding and a simple lack of public awareness. Time will tell if (research in) dogs can truly participate in the combined efforts to improve the quality of life of patients with WD.
Acknowledgements
Funding: This study was funded by the Netherlands Organization for Scientific Research NWO ZON/MW (116004121).
Footnote
Conflicts of Interest: The authors have no conflicts of interest to declare.
References
- Bull PC, Thomas GR, Rommers JM, et al. The Wilson disease gene is a putative copper transporting P-type ATPase similar to the Menkes gene. Nat Genet 1993;5:327-37. [Crossref] [PubMed]
- Tanzi RE, Petrukhin K, Chernov I, et al. The Wilson disease gene is a copper transporting ATPase with homology to the Menkes disease gene. Nat Genet 1993;5:344-50. [Crossref] [PubMed]
- Pierson H, Nuchenditsi A, Byung-Eun K, et al. The function of ATPase copper transporter ATP7B in intestine. Gastroenterology 2018;154:168-80.e5. [Crossref] [PubMed]
- Reed E, Lutsenko S, Bandmann O. Animal models of Wilson disease. J Neurochem 2018;146:356-73. [Crossref] [PubMed]
- Buiakova OI, Xu J, Lutsenko S, et al. Null mutation of the mouse ATP7B (Wilson disease) gene results in intracellular copper accumulation and late-onset hepatic nodular transformation. Hum Mol Genet 1999;8:1665-71. [Crossref] [PubMed]
- Wu J, Forbes JR, Chen H, et al. The LEC rat has a deletion in the copper transporting ATPase gene homologous to the Wilson disease gene. Nat Genet 1994;7:541-5. [Crossref] [PubMed]
- Terada K, Nakako T, Yang XL, et al. Restoration of holoceruloplasmin synthesis in LEC rat after infusion of recombinant adenovirus bearing WND cDNA. J Biol Chem 1998;273:1815-20. [Crossref] [PubMed]
- Ferenci P. Phenotype-genotype correlations in patients with Wilson’s disease. Ann N Y Acad Sci 2014;1315:1-5. [Crossref] [PubMed]
- Lutsenko S. Modifying factors and phenotypic diversity in Wilson’s disease. Ann N Y Acad Sci 2014;1315:56-63. [Crossref] [PubMed]
- Larson G, Karlsson EK, Perri A, et al. Rethinking dog domestication by integrating genetics, archeology, and biogeography. Proc Natl Acad Sci U S A 2012;109:8878-83. [Crossref] [PubMed]
- Parker HG, Shearin AL, Ostrander EA. Man’s best friend becomes biology’s best in show: genome analyses in the domestic dog. Annu Rev Genet 2010;44:309-36. [Crossref] [PubMed]
- Starzl TE, Kaupp HA Jr, Brock DR, et al. Studies on the rejection of the transplanted homologous dog liver. Surg Gynecol Obstet 1961;112:135-44. [PubMed]
- Haywood S, Muller T, Muller W, et al. Copper-associated liver disease in north ronaldsay sheep: a possible animal model for non-wilsonian hepatic copper toxicosis of infancy and childhood. J Pathol 2001;195:264-9. [Crossref] [PubMed]
- Fuentealba IC, Aburto EM. Animal models of copper-associated liver disease. Comp Hepatol 2003;2:5. [Crossref] [PubMed]
- Twedt DC, Sternlieb I, Gilbertson SR. Clinical, morphologic, and chemical studies on copper toxicosis of Bedlington Terriers. J Am Vet Med Assoc 1979;175:269-75. [PubMed]
- Haywood S, Rutgers HC, Christian MK. Hepatitis and copper accumulation in Skye terriers. Vet Pathol 1988;25:408-14. [Crossref] [PubMed]
- Thornburg LP, Rottinghaus G, Dennis G, et al. The relationship between hepatic copper content and morphologic changes in the liver of West Highland White Terriers. Vet Pathol 1996;33:656-61. [Crossref] [PubMed]
- Thornburg LP. Histomorphological and immunohistochemical studies of chronic active hepatitis in Doberman Pinschers. Vet Pathol 1998;35:380-5. [Crossref] [PubMed]
- Webb CB, Twedt DC, Meyer DJ. Copper-associated liver disease in Dalmatians: a review of 10 dogs (1998-2001). J Vet Intern Med 2002;16:665-8. [PubMed]
- Hoffmann G, van den Ingh TS, Bode P, et al. Copper-associated chronic hepatitis in Labrador Retrievers. J Vet Intern Med 2006;20:856-61. [Crossref] [PubMed]
- van de Sluis BJ, Breen M, Nanji M, et al. Genetic mapping of the copper toxicosis locus in Bedlington terriers to dog chromosome 10, in a region syntenic to human chromosome region 2p13-p16. Hum Mol Genet 1999;8:501-7. [Crossref] [PubMed]
- van De Sluis B, Rothuizen J, Pearson PL, et al. Identification of a new copper metabolism gene by positional cloning in a purebred dog population. Hum Mol Genet 2002;11:165-73. [Crossref] [PubMed]
- Müller T, van de Sluis B, Zhernakova A, et al. The canine copper toxicosis gene MURR1 does not cause non-Wilsonian hepatic copper toxicosis. J Hepatol 2003;38:164-8. [Crossref] [PubMed]
- Stuehler B, Reichert J, Stremmel W, et al. Analysis of the human homologue of the canine copper toxicosis gene MURR1 in Wilson disease patients. J Mol Med 2004;82:629-34. [Crossref] [PubMed]
- Lovicu M, Dessi V, Lepori MB, et al. The canine copper toxicosis gene MURR1 is not implicated in the pathogenesis of Wilson disease. J Gastroenterol 2006;41:582-7. [Crossref] [PubMed]
- Wu ZY, Zhao GX, Chen WJ, et al. Mutation analysis of 218 Chinese patients with Wilson disease revealed no correlation between the canine copper toxicosis gene MURR1 and Wilson disease. J Mol Med (Berl) 2006;84:438-42. [Crossref] [PubMed]
- de Bie P, van de Sluis B, Klomp L, et al. The many faces of the copper metabolism protein MURR1/COMMD1. J Hered 2005;96:803-11. [Crossref] [PubMed]
- Maine GN, Burstein E. COMMD proteins: COMMing to the scene. Cell Mol Life Sci 2007;64:1997-2005. [Crossref] [PubMed]
- Fedoseienko A, Bartuzi P, van de Sluis B. Functional understanding of the versatile protein copper metabolism MURR1 domain 1 (COMMD1) in copper homeostasis. Ann N Y Acad Sci 2014;1314:6-14. [Crossref] [PubMed]
- Riera-Romo M. COMMD1: A Multifunctional Regulatory Protein. J Cell Biochem 2018;119:34-51. [Crossref] [PubMed]
- Ganesh L, Burstein E, Guha-Niyogi A, et al. The gene product Murr1 restricts HIV-1 replication in resting CD4+ lymphocytes. Nature 2003;426:853-7. [Crossref] [PubMed]
- Spee B, Arends B, van Wees AM, et al. Functional consequences of RNA interference targeting COMMD1 in a canine hepatic cell line in relation to copper toxicosis. Anim Genet 2007;38:168-70. [Crossref] [PubMed]
- Vonk WI, Bartuzi P, de Bie P, et al. Liver-specific Commd1 knockout mice are susceptible to hepatic copper accumulation. PLoS One 2011;6:e29183. [Crossref] [PubMed]
- de Bie P, van de Sluis B, Burstein E, et al. Distinct Wilson's disease mutations in ATP7B are associated with enhanced binding to COMMD1 and reduced stability of ATP7B. Gastroenterology 2007;133:1316-26. [Crossref] [PubMed]
- Weiss KH, Lozoya JC, Tuma S, et al. Copper-induced translocation of the Wilson disease protein ATP7B independent of Murr1/COMMD1 and Rab7. Am J Pathol 2008;173:1783-94. [Crossref] [PubMed]
- Vonk WI, de Bie P, Wichers CG, et al. The copper-transporting capacity of ATP7A mutants associated with Menkes disease is ameliorated by COMMD1 as a result of improved protein expression. Cell Mol Life Sci 2012;69:149-63. [Crossref] [PubMed]
- Favier RP, Spee B, Penning LC, et al. Copper-induced hepatitis: the COMMD1 deficient dog as a translational animal model for human chronic hepatitis. Vet Q 2011;31:49-60. [Crossref] [PubMed]
- Favier RP, Spee B, Schotanus BA, et al. COMMD1-deficient dogs accumulate copper in hepatocytes and provide a good model for chronic hepatitis and fibrosis. PLoS One 2012;7:e42158. [Crossref] [PubMed]
- Favier RP, Spee B, Fieten H, et al. Aberrant expression of copper associated genes after copper accumulation in COMMD1-deficient dogs. J Trace Elem Med Biol 2015;29:347-53. [Crossref] [PubMed]
- Fieten H, Hugen S, van den Ingh TS, et al. Urinary excretion of copper, zinc and iron with and without D-penicillamine administration in relation to hepatic copper concentrations in dogs. Vet J 2013;197:468-73. [Crossref] [PubMed]
- Fieten H, Penning LC, Leegwater PA, et al. New canine models of copper toxicosis: diagnosis, treatment, and genetics. Ann N Y Acad Sci 2014;1314:42-8. [Crossref] [PubMed]
- Fieten H, Gill Y, Martin AJ, et al. The Menkes and Wilson disease genes counteract in copper toxicosis in Labrador retrievers: a new canine model for copper-metabolism disorders. Dis Model Mech 2016;9:25-38. [Crossref] [PubMed]
- Huch M, Dorrell C, Boj SF, et al. In vitro expansion of single Lgr5+ liver stem cells induced by Wnt-driven regeneration. Nature 2013;494:247-50. [Crossref] [PubMed]
- Huch M, Gehart H, van Boxtel R, et al. Long-term culture of genome-stable bipotent stem cells from adult human liver. Cell 2015;160:299-312. [Crossref] [PubMed]
- Nantasanti S, Spee B, Kruitwagen HS, et al. Disease Modeling and Gene Therapy of Copper Storage Disease in Canine Hepatic Organoids. Stem Cell Reports 2015;5:895-907. [Crossref] [PubMed]
- Boaru SG, Merle U, Uerlings R, et al. Simultaneous monitoring of cerebral metal accumulation in an experimental model of Wilson's disease by laser ablation inductively coupled plasma mass spectrometry. BMC Neurosci 2014;15:98. [Crossref] [PubMed]