A novel macrophage subtype directs hematopoietic stem cell homing and retention
Early embryonic hematopoiesis in vertebrates proceeds in two consecutive waves (Figure 1). The first wave, defined as primitive hematopoiesis, takes place in the extraembryonic yolk sac and generates transitory hematopoietic cell populations consisting of primitive erythrocytes. During this first wave, small number of myeloid cells (e.g., primitive monocytes/macrophages and megakaryocytes) are also generated. The second wave, referred to as definitive hematopoiesis, temporarily occurs in the fetal liver and transitions to the bone marrow and thymus, where multipotent hematopoietic stem cells (HSCs) contribute to the generation of all blood lineages (1-3). In zebrafish, primitive hematopoiesis occurs at early somite stages in the intermediate cell mass (ICM) and the rostral blood island (RBI). The ICM cells originate from the posterior lateral plate mesoderm (LPM) and differentiate into primitive erythrocytes, neutrophils, and thrombocytes; and the RBI cells are derived from the lateral mesoderm of the zebrafish head and differentiate into macrophages (4-8). Across vertebrate species, definitive HSCs are believed to be generated in the aorta-gonad-mesonephros (AGM) region. These HSCs are primarily derived from endothelial cells within the ventral wall of the dorsal aorta. The definitive HSCs in mammals first migrate to and repopulate in the fetal liver and then home to the bone marrow, while the definitive HSCs in zebrafish migrate first to the caudal hematopoietic tissue and then to their definitive anatomical sites in the kidney and thymus (5-8). Despite these apparent differences in definitive hematopoiesis between fish and mammals, the definitive HSC niche functions to actively recruit stem cells and maintain their plasticity throughout adulthood (2,4,5). While much is known regarding these processes, many of the factors which compose the HSC milieu, as well as the precise signaling events that direct HSC migration and preserve stemness, remain undefined. There is growing evidence that during primitive hematopoiesis in the developing embryo that macrophages generated in the yolk sac are involved in the regulation of primitive HSC trafficking—a mechanism suggested to involve in the secretion of inflammatory factors, extracellular matrix (ECM) components, and metalloproteinases (3,9,10). In fact, it is established in zebrafish that macrophages are responsible for the appearance of HSCs in the caudal tissue (10,11). However, the precise macrophage-specific signals regulating HSC recruitment to definitive sites of hematopoiesis are not well defined.
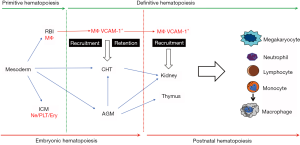
In adults, HSCs residing in the bone marrow may become activated and mobilize into peripheral blood in response to ischemic injury or infection. These HSCs may competently home to extramedullary hematopoietic sites (such as the spleen) or return to their bone marrow HSC niches (12-14). Similarly, HSCs transplanted intravenously into lethally irradiated subjects, home to bone marrow niches to restore hematopoiesis. The homing of HSCs to bone marrow or extramedullary sites is a multistep process of trans-endothelial migration. Vascular cell adhesion molecule-1 (VCAM-1) is a glycoprotein that is primarily expressed in endothelial cells, whose expression is activated in response to proinflammatory cytokines (TNF-α) but also by exposure to reactive oxygen species (ROS), shear stress, or toll like receptor agonists (13). Pro-inflammatory responses can also induce VCAM-1 expression in other cell types, including macrophages. VCAM-1 plays an important role in HSC rolling and adhesion to endothelium via binding to α4β1 integrins. Inhibition of VCAM-1-α4β1 integrin interaction results in impaired HSC trans-endothelial migration and homing to bone marrow and/or extramedullary sites of hematopoiesis (13). Recently, it has also been revealed that VCAM-1+ macrophages, residing in the red pulp of the spleen, are responsible for retention of HSCs and the establishment of extramedullary hematopoiesis. Inhibition of VCAM-1 or depletion of splenic macrophages results in release of HSCs from the spleen to peripheral blood (15). Although, the role of VCAM-1 in adult HSC biology is well studied, there is little known about its role in directing embryonic hematopoiesis.
In this study by Li et al., the authors conducted a lineage tracing of hematopoietic and stem progenitor cells (HSPCs) in zebrafish embryos to understand the mechanism of HSC homing to sites of definitive hematopoiesis (16). Employing genetic models (e.g., forward genetics screening, CRISPR/Cas9 system), the authors followed the fates of HSPCs in caudal hematopoietic tissue—equivalent to fetal liver in mammals. By carefully tracking the location of HSPCs and comparing them to integrin alpha 4 (itga4)-mutant embryos (which displayed HSPC homing defects based on forward genetics screening), the authors identified a niche in which HSPCs home, proliferate, and differentiate into their specialized cell types. The authors defined these niches as “hot spots”. As previous studies have identified a host of secreted regulatory factors from niche cells and extracellular matrix components important for the differentiation of HSPCs (17-19), the authors focused on one particular molecule, VCAM-1, for further study. Interestingly, vcam1-mutants displayed similar defects in homing and differentiation of HSPCs to itga4-mutants, confirming that the ITGA4-VCAM-1 axis is important for homing and retention of HSPCs. When these VCAM-1+ cells were analyzed carefully, these cells were always localized next to HSPCs. Furthermore, these VCAM-1+ cells were not endothelial cells, but in fact, a subset of macrophages based on labeling experiments using cell-type-specific transgenic lines (kdr1 and mpeg1 for endothelial cells and macrophages, respectively).
To elucidate the role of these VCAM-1+ macrophages in the homing and retention of HSPCs, the authors conducted loss- and gain-of-function experiments by depleting macrophages using metronidazole (MTZ) and by expressing wildtype vcam1 in mpeg1+ macrophages in vcam1 mutants, respectively. These experiments showed that: (I) depletion of macrophages did not affect HSPC emergence but caused impaired lodgment of HSPCs and hematopoiesis, demonstrating that macrophages are required for the retention of HSPCs; (II) although HSPCs in macrophage-depleted embryos did not lodge in the caudal hematopoietic tissue, HSPCs could slowly flow in the vasculature, suggesting the role of endothelial VCAM-1 for the initiation of HSPC rolling on the dorsal endothelium bed as reported previously (20); and (III) the retention of HSPCs was restored when vcam1 was re-expressed in mpeg1+ cells. Utilizing mutant and transgenic lines, the authors further investigated the appearance and the retention of VCAM-1+ macrophages and HSPCs. As result, they uncover that ~75% of the lodged HSPCs (in the caudal hematopoietic tissue) interacted with VCAM-1+ macrophages at the entrance of dorsal venous capillaries. By examining the timing of the entrance into the venous capillaries, the authors further defined time of retention of HSPCs and named VCAM-1+ macrophages, usher cells.
The authors convincingly identified a novel subset of macrophages, usher cells, that regulate the homing of HSPCs to a vascular niche. As noted by the authors, the current imaging technology and transgenic zebrafish lines do not allow the distinction between symmetrical and asymmetrical division of cells. The concept of asymmetrical cell division is well known in the field of hematology, referring to the event in which an HSPC divides asymmetrically to give rise to one cell that maintains the stem cell pool and another that is committed to differentiate (21,22). Thus, one way to overcome this problem is to perform in vitro culture of HSPCs and follow their fates in culture using single-cell imaging (23). Also noted by the authors, it is not clear what molecules other than VCAM-1 are expressed by usher cells and niche cells to recruit HSPCs. Since single-cell RNA sequencing (scRNA-seq) has matured in recent years, more molecular analysis of the cells identified in this study using scRNA-seq may provide some clues to identify such molecules. Along the same line, usher cells should be isolated and characterized for their surface markers using flow cytometry. If a specific set of surface markers can be identified, the existence of usher cells (or a similar subset of macrophages) can be confirmed in mammals, which broaden the perspective of the present study.
Acknowledgments
Funding: This study was supported in part by National Institutes of Health Grant (R01-HL141191 & P01-HL078825 to M Wysoczynski; R01-HL141081 to JB Moore IV; P30GM127607 to S Uchida), V.V. Cooke Foundation (Kentucky, USA), and the startup funding from the Mansbach Family, the Gheens Foundation, and other generous supporters at the University of Louisville (to S Uchida.
Footnote
Conflicts of Interest: The authors have no conflicts of interest to declare.
References
- Frame JM, Lim SE, North TE. Hematopoietic stem cell development: Using the zebrafish to identify extrinsic and intrinsic mechanisms regulating hematopoiesis. Methods Cell Biol 2017;138:165-92. [Crossref] [PubMed]
- Golub R, Cumano A. Embryonic hematopoiesis. Blood Cells Mol Dis 2013;51:226-31. [Crossref] [PubMed]
- Cumano A, Godin I. Ontogeny of the hematopoietic system. Annu Rev Immunol 2007;25:745-85. [Crossref] [PubMed]
- Paik EJ, Zon LI. Hematopoietic development in the zebrafish. Int J Dev Biol 2010;54:1127-37. [Crossref] [PubMed]
- Bertrand JY, Traver D. Hematopoietic cell development in the zebrafish embryo. Curr Opin Hematol 2009;16:243-8. [Crossref] [PubMed]
- Murayama E, Kissa K, Zapata A, et al. Tracing hematopoietic precursor migration to successive hematopoietic organs during zebrafish development. Immunity 2006;25:963-75. [Crossref] [PubMed]
- Kissa K, Murayama E, Zapata A, et al. Live imaging of emerging hematopoietic stem cells and early thymus colonization. Blood 2008;111:1147-56. [Crossref] [PubMed]
- Ma D, Zhang J, Lin HF, et al. The identification and characterization of zebrafish hematopoietic stem cells. Blood 2011;118:289-97. [Crossref] [PubMed]
- Wynn TA, Chawla A, Pollard JW. Macrophage biology in development, homeostasis and disease. Nature 2013;496:445-55. [Crossref] [PubMed]
- Li Y, Esain V, Teng L, et al. Inflammatory signaling regulates embryonic hematopoietic stem and progenitor cell production. Genes Dev 2014;28:2597-612. [Crossref] [PubMed]
- Travnickova J, Tran Chau V, Julien E, et al. Primitive macrophages control HSPC mobilization and definitive haematopoiesis. Nat Commun 2015;6:6227. [Crossref] [PubMed]
- Robbins CS, Chudnovskiy A, Rauch PJ, et al. Extramedullary hematopoiesis generates Ly-6C(high) monocytes that infiltrate atherosclerotic lesions. Circulation 2012;125:364-74. [Crossref] [PubMed]
- Leuschner F, Rauch PJ, Ueno T, et al. Rapid monocyte kinetics in acute myocardial infarction are sustained by extramedullary monocytopoiesis. J Exp Med 2012;209:123-37. [Crossref] [PubMed]
- Dutta P, Courties G, Wei Y, et al. Myocardial infarction accelerates atherosclerosis. Nature 2012;487:325-9. [Crossref] [PubMed]
- Dutta P, Hoyer FF, Grigoryeva LS, et al. Macrophages retain hematopoietic stem cells in the spleen via VCAM-1. J Exp Med 2015;212:497-512. [Crossref] [PubMed]
- Li D, Xue W, Li M, et al. VCAM-1(+) macrophages guide the homing of HSPCs to a vascular niche. Nature 2018;564:119-24. [Crossref] [PubMed]
- Xue Y, Lv J, Zhang C, et al. The Vascular Niche Regulates Hematopoietic Stem and Progenitor Cell Lodgment and Expansion via klf6a-ccl25b. Dev Cell 2017;42:349-62.e4. [Crossref] [PubMed]
- Mahony CB, Fish RJ, Pasche C, et al. tfec controls the hematopoietic stem cell vascular niche during zebrafish embryogenesis. Blood 2016;128:1336-45. [Crossref] [PubMed]
- Nombela-Arrieta C, Pivarnik G, Winkel B, et al. Quantitative imaging of haematopoietic stem and progenitor cell localization and hypoxic status in the bone marrow microenvironment. Nat Cell Biol 2013;15:533-43. [Crossref] [PubMed]
- Berlin C, Bargatze RF, Campbell JJ, et al. alpha 4 integrins mediate lymphocyte attachment and rolling under physiologic flow. Cell 1995;80:413-22. [Crossref] [PubMed]
- Suda T, Suda J, Ogawa M. Disparate differentiation in mouse hemopoietic colonies derived from paired progenitors. Proc Natl Acad Sci U S A 1984;81:2520-4. [Crossref] [PubMed]
- Schroeder T. Heterogeneity of sister cell fates. Nat Rev Mol Cell Biol 2013;14:327. [Crossref] [PubMed]
- Schroeder T. Long-term single-cell imaging of mammalian stem cells. Nat Methods 2011;8:S30-5. [Crossref] [PubMed]