Inhibition of pyroptosis attenuates Staphylococcus aureus-induced bone injury in traumatic osteomyelitis
Introduction
Osteomyelitis is an infection of bone or bone marrow and leads to progressive bone destruction, aberrant bone neoformation and systemic inflammatory reaction (1). Among the bacteria species isolated from osteomyelitis, S. aureus (Staphylococcus) is the most prevalent bacterial subtype, accounting for approximately 80% of all osteomyelitis pathogen, especially for septic osteomyelitis due to trauma (2). Although our understanding towards the pathophysiology and treatment of osteomyelitis has progressed significantly, it remains a clinical conundrum for orthopedic surgeons, especially cases caused by open fracture trauma (3).
It is well-established that osteomyelitis is closely associated with infection, though the precise mechanism by which bacterial infection leads to bone destruction and aberrant bone neoformation remains unclear (4). Previous studies illustrated that bacterial infection led to inflammatory bone loss due to decreased activity of osteoblasts and increased formation of osteoclasts (5). Bacterial invasion decreased the activity and viability of osteoblasts through apoptosis-dependent and apoptosis-independent pathways. On the other hand, inflammatory mediators produced by infiltrating leukocytes and metabolic products secreted by bacteria promoted the formation and activity of osteoclast (6).
Currently, increasing studies suggested that the administration of anti-inflammatory drugs like antibiotics could not remarkably alleviate the traumatic osteomyelitis induced bone injury, along with a high recurrence rate (1). Thus, investigations on the molecular mechanisms contributing to osteoblast and osteoclast disequilibrium in osteomyelitis may provide additional insight into the pathophysiology of osteomyelitis. Recently, some studies indicated that multiple cell death pathways played essential roles in the regulation of various pathophysiologic process in bacteria-induced inflammation (7,8). Furthermore, previous studies showed that SpA (Staphylococcal protein A), a cell wall anchored protein released from the bacterial surface, caused the excessive release of cytokines and apoptosis of osteoblast (9). As a typical form of programmed cell death, apoptosis was then found to be involved in the inflammatory reaction and bone destruction in osteomyelitis (10).
Pyroptosis, a novel programmed cell death, has been discovered and classified in recent years (11). Pyroptosis is characterized by cell swelling, rapid plasma-membrane rupture and release of proinflammatory contents including IL-1β, IL-6 and IL-18 (12). This newly defined type of programmed cell death is regarded as gasdermin-mediated programmed necrosis in response to external insults such as bacteria, virus and fungus (13). Additionally, several studies have demonstrated that pyroptosis plays a crucial role in many infectious diseases (12). Recent study illustrated that inhibition of pyroptosis in S. aureus pneumonia and acute septic liver injury could partially alleviate the inflammatory injury in animal models (14). However, little is known about the effects of pyroptosis on osteomyelitis.
In summary, our results present experimental evidence that pyroptosis plays an important role in osteomyelitis both in human and murine model. Furthermore, the inhibition of pyroptosis can partially attenuate S. aureus induced bone destruction and restore the balance between osteoblast and osteoclast in osteomyelitis.
Methods
Animals
Male C57BL/6 mice, aged 6 weeks, 20±2 g, were purchased from the Model Animal Research Center of Nanjing University. Mice were housed at 21–23 °C under a 12-hour light/dark cycle with free access to food and water. All animal experiments were performed according to the guidelines for the care and use of animals and approved by the Animal Care and Use Committee of the Nanjing University in accordance with the Institutional Animal Care and Use Committee guidelines.
Bacterial culture conditions
S. aureus strain 6,850 (ATCC 53,657; ATCC, Middlesex, UK) was used in this study. It was cultured in tryptic soy broth (TSB) at 37 °C with shaking. The average number of phage per bacterium and the multiplicity of infection (MOI) was determined by dividing the number of phage (PFU/mL) by the number of bacteria (cells/mL). For cell infection, cells were incubated with prepared bacterial suspensions at a MOI of 100. Infected cells were pre-incubated in 37 °C for assays.
Bone marrow macrophages (BMMs) isolated osteoclast differentiation and tartrate-resistant acid phosphatase (TRAP) staining
Bone marrow cells were obtained from femurs of 4-week-old mice and maintained in α-MEM complete media supplemented with 10% fetal bovine serum (FBS; Gibco BRL, Gaithersburg, MD, U lassified as BMMs. BMMs (7×103 cells/well) were cultured in complete medium in the presence of M-SA), 100 U/mL penicillin in the presence of M-CSF (50 ng/mL) for 3 days. Adherent cells on bottoms were cCSF (50 ng/mL) and RANKL (50 ng/mL) in a 6-well plate treated with DMSO, bacteria medium, bacteria medium with Glyburide or Ac-YVAD-CMK. After 7 days, cells were fixed with 4% paraformaldehyde and then stained with TRAP using a TRAP Assay Kit (Keygen, China). TRAP-positive multinucleated cells were viewed as mature osteoclast.
Murine osteomyelitis model
Briefly, after anesthesia with isoflurane in O2, femoral condyles were exposed through a lateral parapatellar arthrotomy with medial displacement of the quadriceps-patellar complex as described before (15). The fossa inter-condyloid was perforated using a high-speed drill with a 0.5-mm sharp steel burr (Fine Science Tools Inc., Foster city, CA, USA). Then, a channel was created using a 23-gauge (external diameter, 0.6 mm) needle, through which the bioluminescent strain of S. aureus (1.0×108 CFU) in 1 µL medium was injected into the medullary cavity of the femur using a 1 mL syringe. Finally, the hole was filled with bone wax and the muscle and skin were closed by sutures. Phosphate-buffered saline (PBS) was administered to the control group. Mice were sacrificed at 3 or 7 days after surgery as planned.
Microcomputed tomography (µCT) analysis
The femurs were excised, cleaned of soft tissue and stored in paraformaldehyde overnight. The vivaCT 80 (Scanco Medical, Bruettisellen, Switzerland) was used to analyze the bone destruction. The scanner was operated at 55 keV, 145 µA, 32 mm FOV, an integration time of 200 ms and a nominal isotropic image voxel size of 15.6 µm.
For bone destruction analysis, the region of 1.0 mm height was chosen in the femur midshaft. Regions of interest for each compartment were manually marked and bone destruction volume fraction was generated. The analyses were performed with the software provided by the manufacturer of the µCT (V6.5-3, Scanco Medical, Bruettisellen, Switzerland).
Enzyme linked immunosorbent assay (ELISA)
A total of 600 µL of blood was collected from the hearts of the mice following anesthesia. ELISA assay kits were used to measure the concentration of the biomarkers IL-1β and C-reactive protein (CRP) according to the manufacturer’s instructions (Multi Sciences, China). The optical absorbance at 450 and 570 nm was determined using a microplate absorbance reader (Model 680 Microplate Reader, Bio-Rad).
Quantitative real-time PCR
Total RNA was extracted using TRIzol (Takara, Japan). The cDNA was synthesized from total RNA by a reverse transcriptase cDNA synthesis kit (Takara, Japan). Quantitative PCR was carried out on a 7500 Real-Time PCR System (Applied Biosystems, MA, USA) using SYBR Premix Ex Taq (Takara, Shiga, Japan). Forward and reverse primers were listed in Table 1. All data were normalized to β-actin and assays were performed in triplicate.
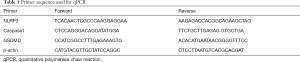
Full table
Human samples
Normal human bone tissues were isolated from bone fragments of femoral neck fracture patients. The infectious bone tissues were collected from osteomyelitis patients undergoing debridement. All samples were obtained from the Drum Tower Hospital of Nanjing University, the Affiliated Hospital of Nanjing University. This study was approved by the hospital ethics review committee (2009022).
Western blot analysis
Western blot assays of bone tissues or cultured MC3T3-E1 cells with various treatments were performed as before (16). The blots were processed with primary antibodies to Caspase 1 (Santa Cruz, USA), GSDMD, NLRP3 (Abcam, Cambridge, USA), Runx2 (Proteintech, USA) and collagen I (Abclonal, USA) respectively at the predetermined dilutions and followed by incubation with horse radish peroxidase (HRP)-conjugated secondary antibodies. Western blots were visualized using an ECL plus Western blotting detection system.
Statistical analysis
Data are presented as means ± SD of at least three independent experiments. The statistical difference was analyzed by Student’s t-test for comparisons of two groups or one-way ANOVA for two factor experiments or one-way ANOVA followed by Tukey’s test for comparisons of multiple groups. Values of P<0.05 were considered statistically significant.
Results
Expression of pyroptosis-associated proteins was increased in both human and murine osteomyelitis
To detect whether pyroptosis is involved in osteomyelitis, we extracted proteins from bone fragments of osteomyelitis patients undergoing debridement and non-infectious patients undergoing femoral neck fractures and examined the expression of pyroptosis-associated proteins including NLRP3, caspase1 and GSDMD by immunoblotting. The results of immunoblotting demonstrated that the expression of NLRP3 and GADMD was significantly higher in osteomyelitis patients than that in femoral neck fracture patients (Figure 1A,B). Additionally, we also detected the expression of caspase3 and RIP3 to verify the role of apoptosis and necroptosis in osteomyelitis (Figure 1A,B). The results illustrated that there was no significant difference between femoral neck fracture patients and osteomyelitis patients in expression of caspase3 and RIP3 (Figure 1B).
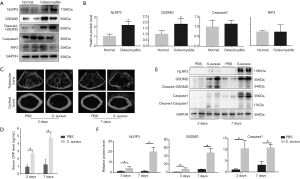
To further explore the effect of pyroptosis on osteomyelitis, we established murine septic osteomyelitis model. The µCT reconstructed images presented an obvious bacterial invasion in both bone marrow cavity and cancellous bone, along with a clear bone destruction in bacteria-treated group (Figure 1C). Besides, the systemic inflammatory marker CRP was also significantly higher in bacteria-treated group both 3 and 7 days after surgery (Figure 1D). To evaluate whether pyroptosis was involved in the bone injury caused by osteomyelitis in mice, we then detected three important proteins of pyroptosis, including NLRP3, caspase1 and GSDMD (Figure 1E). Obviously, compared with the PBS group, the S. aureus treated group presented significantly higher expression of NLRP3, caspase1 and GSDMD, both 3 and 7 days after surgery (Figure 1F).
Caspase1 inhibitor Ac-YVAD-CMK alleviated S. aureus-induced pyroptosis both in vivo and in vitro
To evaluate the key role of caspase1 in pyroptosis, MC3T3-E1 cells were pretreated with specific caspase1 inhibitor Ac-YVAD-CMK at an increasing dose in an infectious cell model. The results of real-time qPCR (Figure 2A) and Western blot showed that Ac-YVAD-CMK significantly downregulated the expression of caspase1 and downstream GSDMD in a dose-dependent manner both in transcriptional and translational level (Figure 2B,C). Furthermore, Ac-YVAD-CMK was then intraperitoneally injected into mice to verify the impact of caspase1 on pyroptosis in vivo. The results of immunoblotting indicated that the expression of caspase1 and GSDMD were remarkably elevated by bacterial stimulation both 3 and 7 days after surgery. Interestingly, pretreatment with Ac-YVAD-CMK significantly reduced the elevated expression of caspase1 and GSDMD (Figure 2D,E).
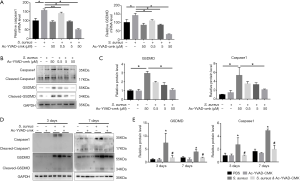
NLRP3 inhibitor glyburide attenuated S. aureus induced pyroptosis both in vivo and in vitro
To evaluate the key role of NLRP3 in pyroptosis, MC3T3-E1 cells were pretreated with specific NLRP3 inhibitor glyburide at an increasing dose in an infectious cell model. Glyburide significantly downregulated the expression of NLRP3, Caspase1 and GSDMD in a dose-dependent manner both in transcriptional and translational level (Figure 3A,B,C). Meanwhile, Glyburide was then intraperitoneally injected into mice to test the impact of NLRP3 on pyroptosis in vivo. The results of western blot indicated that the expression of NLRP3, caspase1 and GSDMD were significantly increased by S. aureus-induced osteomyelitis both 3 and 7 days after surgery. Meanwhile, pretreatment with Glyburide significantly decreased the high expression of NLRP3, caspase1 and GSDMD (Figure 3D,E).
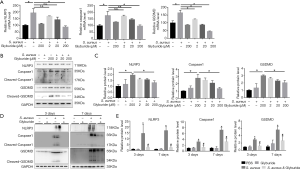
Pyroptosis inhibitors reversed bone injury caused by bacteria-induced pyroptosis in osteomyelitis
To evaluate the bone destruction induced by pyroptosis in osteomyelitis, we first tested the bone formation marker in MC3T3-E1 cell line. The results illustrated that the expression of two bone formative marker, Runx2 and Col I, were significantly decreased upon treated with S. aureus and the downregulation of Runx2 and Col I could be partially reversed by pyroptosis-associated protein inhibitors (Figure 4A,B,C,D), which indicated that inhibition of caspase1 and NLRP3 could not only alleviate pyroptosis, but also partially reversed the pyroptosis-induced downregulation of bone formative activity. Meanwhile, to further explore the balance between osteoblast and osteoclast in pyroptosis, we also tested the activity of osteoclast in both presence and absence of pyroptosis inhibitors by TRAP staining. In control group, Bone marrow derived monocytes/macrophages (BMMs) pretreated with DMSO began to differentiate into representative TRAP-positive multinucleated osteoclasts after 7 days treated with M-CSF and RANKL. Meanwhile, the formation of TRAP-positive multinucleated osteoclasts was significantly activated after S. aureus treatment. However, when pretreated with pyroptosis-associated protein inhibitor Ac-YVAD-CMK or Glyburide, the activation of TRAP-positive multinucleated osteoclasts by S. aureus was inhibited (Figure 4E). Generally, µCT reconstructed images proved that the inhibition of pyroptosis reversed the bone injury in osteomyelitis (Figure 4F). In addition, the inhibition of pyroptosis also attenuated the elevation of systemic inflammatory factors like IL-1β and IL-18 both 3 and 7 days after surgery (Figure 4G).
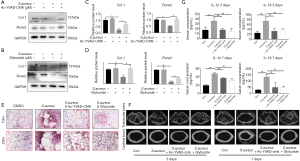
Discussion
As traumatic osteomyelitis brings about severe clinical complications, increasing researchers have concentrated on the pathophysiology of osteomyelitis. Our study presents several novel findings compared with previous studies. Firstly, pyroptosis is reported as a novel characterized type of cell programmed death and we verified its key role in osteomyelitis for the first time. Secondly, inhibition of caspase1 or NLRP3, two key mediators in pyroptosis pathway, could obviously relieve pyroptosis and subsequently attenuate inflammatory response and bone injury in osteomyelitis. Thus, considering the encouraging therapeutic results following caspase1 and NLRP3 inhibitor administration, these two inhibitors have the potential to be therapeutic targets towards osteomyelitis.
Surgical treatment has been shown as an acceptable option to relieve the complications of osteomyelitis. However, it still retains several side effects, including poor soft tissue coverage and infectious nonunion (17). Considering the unsatisfying results of surgical treatment, increasing researchers focused on the pathophysiology of osteomyelitis and tried to block the cascaded effect caused by bacterial infection. Previous studies indicated that apoptosis, a major form of programmed cell death, plays a crucial role in the inflammatory response of septic osteomyelitis (18). However, whether pyroptosis, another important form of programmed cell death, is involved in septic osteomyelitis has not been characterized yet. In present study, we found that compared to patients without osteomyelitis (femoral neck fracture), osteomyelitis patients presented a significantly higher expression of NLRP3 and GSDMD in the infected bone, indicating that pyroptosis was involved in the pathogenesis of osteomyelitis. Moreover, there was no significant difference in the expression of caspase3 and RIP3, typical proteins of apoptosis and necroptosis respectively, between osteomyelitis patients and normal patients, which was different from previous researches. We speculated that the reason for this inconsistence is that the control group in this study were patients with femoral neck fractures and apoptosis was likely to be involved in these patients (19-21).
Previous studies demonstrated that pyroptosis was widely involved in the initiation and development of a host of infectious diseases, particularly those caused by gram positive bacteria (22,23). The murine osteomyelitis model in our study presented robust inflammatory response and osteolysis. Simultaneously, pyroptosis was also activated in the S. aureus-infection cell model, along with decreased levels of bone formation markers, including collagen I and Runx2. The results validated the adverse effects of pyroptosis in bone formation in osteomyelitis. Furthermore, our results suggested that inhibition of pyroptosis by caspase1 inhibitor, AC-YVAD-CMK, could not only effectively attenuate the bacterial infection-induced pyroptosis as described before, but also helped to restore the expression of bone formation marker like collagen I and Runx2 in vitro and relieved the bacteria-induced bone destruction in vivo. Meanwhile, as the upstream of caspase1, NLRP3 was viewed as another key mediator in pyroptosis for its pivotal role as an inflammatory sensor in canonical pyroptosis (24). There exist studies illustrating that inhibition of the NLRP3 inflammasome reduced the severity of experimentally-induced acute pancreatitis in obese mice (25). Thus, in our study, Glyburide, a widely used inhibitor of NLRP3, was applied to detecting the role of NLRP3 in septic inflammation-induced pyroptosis in osteomyelitis. Intriguingly, we found that bone destruction and excessive inflammatory response was relieved after Glyburide administration. On the other hand, osteoclast was reported to drive bone resorption at early stage of inflammation. Although we did not detect bone formation and resorption markers in vivo, our experiment still illustrated that the administration of AC-YVAD-CMK and Glyburide significantly reversed the aberrantly upregulated activity of osteoclast after pretreatment with S. aureus. This observation indirectly confirmed that the inhibition of osteoblast and activation of osteoclast by pyroptosis played an important role in osteomyelitis. Given the fact that Glyburide was a hypoglycemic drug prevalently used extensively, our findings also provided the potential to enlarge the indication of this traditional anti-diabetic drugs, especially for patients who suffer from diabetes mellitus and osteomyelitis simultaneously.
Briefly, as described in Figure 5, upon stimulated by S. aureus, cellular inflammatory sensor NLRP3 activated caspase1, which cleaves GSDMD and leads to the formation of the pores on the membrane. These pores contribute to cell swelling and eventual lysis, along with the release of inflammatory factors including IL-1β and IL-18 in osteoblast. Besides, sRANK-L (soluble receptor activator of nuclear factor kappa-B ligand) is released from the pores and subsequently leads to excessive formation and activation of osteoclasts, resulting in severe bone destruction.
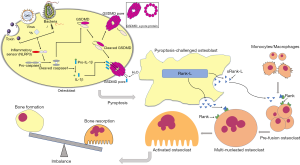
Clearly, the finding in the present study must be interpreted with the recognition of its limitation. As the specific terminal pyroptosis executioner, GSDMD was reported to release its gasdermin-N domain that perforates the plasma membrane to induce cell swelling and osmotic lysis (26). However, we did not find the suitable inhibitor or inhibited methods to block GSDMD’s function in pyroptosis during osteomyelitis. Also, we should use more methods to detect the expression of proteins in our experiment. Additionally, to fulfill the function of anti-pyroptosis, the concentration of Glyburide was 10 times higher than that of anti-hyperglycemia, which indicates increased probability of side effects. Further studies are needed to discover a more suitable drugs like MCC950 to inhibit pyroptosis and alleviate the progression of osteomyelitis (27).
In summary, our results suggested that pyroptosis aggravated the bone injury and inflammatory response in septic osteomyelitis. Inhibition of pyroptosis by caspase1 and NLRP3 inhibitors could alleviate inflammation and bone injury, providing a potential target towards osteomyelitis.
Acknowledgements
Funding: This study was supported by the International Cooperation and Exchanges of National Natural Science Foundation [NSFC 81420108021], Key Program of NSFC [81730067], Excellent Young Scholars NSFC (81622033), Jiangsu Provincial Key Medical Center Foundation and Jiangsu Provincial Medical Outstanding Talent Foundation, Jiangsu Provincial Medical Youth Talent Foundation and Jiangsu Provincial Key Medical Talent Foundation.
Footnote
Conflicts of Interest: The authors have no conflicts of interest to declare.
Ethical Statement: This study was approved by the hospital ethics review committee (2009022).
References
- Kavanagh N, Ryan EJ, Widaa A, et al. Staphylococcal osteomyelitis: Disease progression, treatment challenges, and future directions. Clin Microbiol Rev 2018;31:1-25. [Crossref] [PubMed]
- Sanders J, Mauffrey C. Long Bone Osteomyelitis in Adults: Fundamental Concepts and Current Techniques. Orthopedics 2013;36:368-75. [Crossref] [PubMed]
- Kankilic B, Bilgic E, Korkusuz P, et al. Vancomycin containing PLLA/β-TCP controls experimental osteomyelitis in vivo. J Orthop Surg Res 2014;9:114. [Crossref] [PubMed]
- Schmidt-Rohlfing B, Lemmen SW, et al. Osteomyelitis in adults. Diagnostic principles and therapeutic strategies. Unfallchirurg 2012;115:55-66. [Crossref] [PubMed]
- Mödinger Y, Löffler B, Huber-Lang M, et al. Complement involvement in bone homeostasis and bone disorders. Semin Immunol 2018;37:53-65. [Crossref] [PubMed]
- Chen X, Jiao J, He XQ, et al. CHI3L1 regulation of inflammation and the effects on osteogenesis in a Staphylococcus aureus-induced murine model of osteomyelitis. FEBS J 2017;284:1738-47. [Crossref] [PubMed]
- Zhan R, Zhao M, Zhou T, et al. Dapsone protects brain microvascular integrity from high-fat diet induced LDL oxidation. Cell Death Dis 2018;9:683. [Crossref] [PubMed]
- Sun Z, Zhao T, Lv S, et al. Dexmedetomidine attenuates spinal cord ischemia–reperfusion injury through both anti-inflammation and anti-apoptosis mechanisms in rabbits. J Transl Med 2018;16:209. [Crossref] [PubMed]
- Kim HK, Falugi F, Missiakas DM, et al. Peptidoglycan-linked protein A promotes T cell-dependent antibody expansion during Staphylococcus aureus infection. Proc Natl Acad Sci 2016;113:5718-23. [Crossref] [PubMed]
- Young AB, Cooley ID, Chauhan VS, et al. Causative agents of osteomyelitis induce death domain-containing TNF-related apoptosis-inducing ligand receptor expression on osteoblasts. Bone 2011;48:857-63. [Crossref] [PubMed]
- Shi J, Gao W, Shao F. Pyroptosis: Gasdermin-Mediated Programmed Necrotic Cell Death. Trends Biochem Sci 2017;42:245-54. [Crossref] [PubMed]
- Liu X, Zhang Z, Ruan J, et al. Inflammasome-activated gasdermin D causes pyroptosis by forming membrane pores. Nature 2016;535:153-8. [Crossref] [PubMed]
- Shi J, Zhao Y, Wang Y, et al. Inflammatory caspases are innate immune receptors for intracellular LPS. Nature 2014;514:187-92. [Crossref] [PubMed]
- Chen YL, Xu G, Liang X, et al. Inhibition of hepatic cells pyroptosis attenuates CLP-induced acute liver injury. Am J Transl Res 2016;8:5685-95. [PubMed]
- Funao H, Ishii K, Nagai S, et al. Establishment of a real-time, quantitative, and reproducible mouse model of staphylococcus osteomyelitis using bioluminescence imaging. Infect Immun 2012;80:733-41. [Crossref] [PubMed]
- Shi T, Lu K, Shen S, et al. Fenofibrate decreases the bone quality by down regulating Runx2 in high-fat-diet induced Type 2 diabetes mellitus mouse model. Lipids Health Dis 2017;16:201-9. [Crossref] [PubMed]
- Ford CA, Cassat JE. Advances in the local and targeted delivery of anti-infective agents for management of osteomyelitis. Expert Rev Anti Infect Ther 2017;15:851-60. [Crossref] [PubMed]
- Chen Q, Hou T, Luo F, et al. Involvement of toll-like receptor 2 and pro-apoptotic signaling pathways in bone remodeling in osteomyelitis. Cell Physiol Biochem 2014;34:1890-900. [Crossref] [PubMed]
- Aghajanian P, Mohan S. The art of building bone: Emerging role of chondrocyte-to-osteoblast transdifferentiation in endochondral ossification. Bone Res 2018;6:19. [Crossref] [PubMed]
- Power J, Poole KE, Van Bezooijen R, et al. Sclerostin and the regulation of bone formation: Effects in hip osteoarthritis and femoral neck fracture. J Bone Miner Res 2010;25:1867-76. [Crossref] [PubMed]
- Sutherland MK, Geoghegan JC, Yu C, et al. Sclerostin promotes the apoptosis of human osteoblastic cells: A novel regulation of bone formation. Bone 2004;35:828-35. [Crossref] [PubMed]
- Xi H, Zhang Y, Xu Y, et al. Caspase-1 Inflammasome Activation Mediates Homocysteine-Induced Pyrop-Apoptosis in Endothelial Cells. Circ Res 2016;118:1525-39. [Crossref] [PubMed]
- Yuan J, Najafov A, Py BF. Roles of Caspases in Necrotic Cell Death. Cell 2016;167:1693-704. [Crossref] [PubMed]
- Wree A, Eguchi A, McGeough MD, et al. NLRP3 inflammasome activation results in hepatocyte pyroptosis, liver inflammation, and fibrosis in mice. Hepatology 2014;59:898-910. [Crossref] [PubMed]
- Li Y, Pan Y, Gao L, et al. Dexmedetomidine attenuates pancreatic injury and inflammatory response in mice with pancreatitis by possible reduction of NLRP3 activation and up-regulation of NET expression. Biochem Biophys Res Commun 2018;495:2439-47. [Crossref] [PubMed]
- Man SM, Karki R, Kanneganti TD. Molecular mechanisms and functions of pyroptosis, inflammatory caspases and inflammasomes in infectious diseases. Immunol Rev 2017;277:61-75. [Crossref] [PubMed]
- Coll RC, Robertson AA, Chae JJ, et al. A small-molecule inhibitor of the NLRP3 inflammasome for the treatment of inflammatory diseases. Nat Med 2015;21:248-55. [Crossref] [PubMed]