Mild gait impairment in long-term treated patients with neurological Wilson’s disease
Introduction
Wilson’s disease (WD) is an autosomal recessively inherited disorder of copper metabolism caused by a defect of the gene ATP7B localized on chromosome 13 [13q14.3-q21.1; (1-3)]. Copper transport in the human body is regulated by an ATP7B encoded enzyme (1). In WD-patients reduced synthesis of ceruloplasmin and reduced biliary excretion of copper cause elevated serum levels of unbound copper and tissue accumulation of toxic copper ions (4). Multiple organs become affected during the course of untreated WD (5).
In the central nervous system (CNS), copper is predominantly stored in the grey matter of the basal ganglia and the cortex (6,7) resulting in typical neuropsychiatric manifestations (5-10). But MRI- and PET-scanning also indicate the additional involvement of the brainstem und cerebellar nuclei (6,7). Little is known about the clinical implications of the involvement of these structures in the disease process. Impaired eye movements (5,11) and impaired breathing (12) have been described, but not studied in detail. Walking is another motor task which is influenced by basal ganglia, cerebellar and brainstem input. Clinically dystonic, ataxic and parkinsonian gait patterns are distinguished and scored in WD (9,10,13), but have not been analyzed quantitatively so far.
Walking is a basic motor skill and the first locomotion pattern to appear in children in an upright position (14). By about 3 years of age, lower limb joint motion during walking develops adult-like consistency (15). Since WD does usually not become manifest before the age of 5 (5), it can be assumed that WD-patients have learnt to walk normally. The prevalence of gait abnormalities among initial symptoms of WD ranges from not being present or not being observed over 10% (16), 17% (17) and 40% (18) to 45% (9) up to 59% (13) and even 75% (8).
Using clinical scores to semi-quantify gait disturbance in WD, Burke et al. (9) were able to demonstrate that gait responded excellently to therapy in WD in comparison to other neurological symptoms and in contrast to dystonia in 85 patients who had been recruited in 3 different clinical trials to test safety and efficacy of tetramolybdate [TM®; (9)]. This implies that in long-term treated WD-patients in contrast to de novo patients, gait disturbances will become rare and mild.
Therefore, the present study was performed, using the easy to handle Infotronic® gait analysis system (19,20), which allows to measure easily the spatiotemporal relationship between both legs as well as the pressure of the feet to the ground. Evidence is presented that the adherence to therapy in WD has an influence on gait speed and cadence, but that the central pattern generator of walking (organizing the spatiotemporal time structure of bipedal interaction) appears to remain intact in WD.
Methods
Patients and controls
In the outpatient department of the University hospital in Düsseldorf (Germany), more than 120 patients with WD are seen on a regular basis every 3, 6, 9 or 12 months depending on the clinical outcome and duration of treatment. Out of these patients, a cohort of 30 patients was consecutively recruited after they had been informed on the purpose of this study and had given informed consent. Patients with a history of walking deficits before the age of 5 were excluded from the study. Demographical data (age, distribution of sex, age at diagnosis, body weight, body size) of the patients and an age- and sex-matched control group are presented in Table 1.
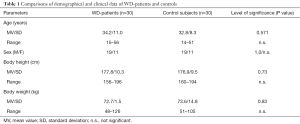
Full table
Clinical examination and scoring of neurological symptoms
Before gait analysis, patients and controls underwent a detailed clinical neurological investigation. Seven motor symptoms (dystonia, dysarthria, bradykinesia, tremor, gait disturbance, oculomotor deficits, ataxia of extremities) as well as three non-motor symptoms (reflex abnormalities, sensory symptoms, neuropsychological and psychiatric symptoms) were scored on whether these symptoms were absent [0] or only mildly [1], moderately [2] or severely [3] present. The 7 motor subscores were summed up to yield a motor score (MotS; 0–21), the three non-motor items were summed up to a non-motor score (N-MotS; 0–9), and the sum of MotS and N-MotS yielded the total score (TS; 0–30). These scores have already been used in previous studies (10,18). A similar score is used by the Italian Monotematica AISF liver transplantation group (21). Patients with TS <3 were classified as mildly, those with TS between 3 and 6 as moderately and those with TS >6 as severely affected. Control subjects with a TS >0 were excluded from the study.
Laboratory findings
From the detailed laboratory testing being performed as routine therapy monitoring the following parameters were selected for correlation with clinical score and walking parameters: (I) parameters of copper metabolism (ceruloplasmin, serum copper, concentration of copper in the 24 h urine collected under medication); (II) liver enzymes (GOT, GPT, GGT),; (III) coagulation [thromboplastin time (PTT), international normalized ratio (INR)]; (IV) kidney function [serum level of creatinine (Crea)].
Gait measurement
Before the gait measurements the recording device was demonstrated to the patients. Measurements were only initiated after patients had given their consent a second time. All patients were able to walk without aid. None of the patients wore orthopaedic shoes or an ankle foot orthosis. Patients and controls had to walk a distance of 40 m at the preferred (natural) walking speed. Patients had to use their usual street shoes.
For gait measurement, the Ultraflex Infotronic® system (NL-7650 AB Tubbergen, The Netherlands) was used [for photos and further details see http://www.infotronic.nl and (19,20)]. It consists of light tissue shoes which can easily be strapped over the street shoes. In each of the two solid, but flexible plates of the two Infotronic® shoes 8 force transducers are integrated which record the vertical component of the ground reaction forces (= GFz-curve). This system automatically yields a variety of parameters. For determination of the onset and end of the stance phase those timing points were chosen by the system where the GFz-curve crossed the force level of 5% of peak amplitude.
For further analysis, the following parameters were selected: DUR = duration to walk the distance of 40 meters in secs; GSP = gait speed in m/s = 40m/DUR; CAD = steps/sec and number of steps (NUM) needed to walk 40m, stance time from heel-on to toe-off (STANR, STANL), single swing right and left (SSWR, SSWL), double support right and left (DSR, DSL), and step time (= double support plus single stance=double support plus single swing of the contralateral side; STEPR, STEPL) (see Figure 1).
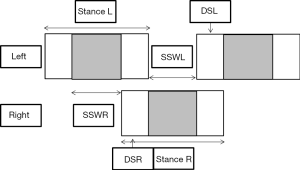
For time normalization, STAN, STEP, SSW, and DS were divided by cycle time (STEPR + STEPL) yielding the parameters TNSTN, TNSTEP, TNSSW, and TNDS.
Statistics
Group comparisons were performed non-parametrically using Wilcoxon’s test. For the correlation analysis the non-parametric Spearman’s rho (rank correlation) was used. All tests were part of the commercially available SSPS statistics package (version 25; IBM Analytics, Armonk, USA).
Results
Comparison of demographical data of WD-patients and control subjects
In Table 1 demographical data of the patient cohort and the control group are compared. Patients were perfectly sex-matched by the controls, there was no significant difference in age, body size and body weight. Mean age at diagnosis ranged from 11 to 36 years (mean: 22.0; SD: 6.8 years) and duration of treatment from 31 to 376 months (mean: 144; SD: 106 months).
Clinical findings in the WD-patients
The most frequently observed motor abnormality was bradykinesia (90%) followed by dysarthria (66%), tremor 41%, dystonia (38%) and cerebellar abnormalities (38%). During clinical investigation only 6 patients (20%) presented with a gait disorder: two patients suffered from a typical parkinsonian gait (patient 1: TS =12; patient 2: TS =7), three patients from a mild dystonic gait (patient 3: TS =7; patient 4: TS =7; patient 5: TS =4) and one patient from an ataxic gait (patient 6: TS =16). Five of these patients presented with additional cerebellar symptoms and were unable to hop in place [for details see (10)].
Walking speed and cadence in WD-patients and control subjects
To walk a distance of 40 meters, WD-patients needed significantly (P<0.001) more time (DUR), had a significantly (P<0.001) lower cadence (CAD) and therewith a significantly (P<0.001) lower gait speed (GSP) than the control subjects. But the number of steps (NUM) and therewith step length needed to walk 40 meters did not differ significantly between patients and controls (see Table 2).
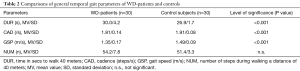
Full table
Recording of GFz-curves in differently affected patients
In Figure 2 the sequences of ground contacts of the left (L) and right foot (R) of a normal subject (left side), a moderately affected patient (TS =5; middle) and a severely affected patient (TS =12; right part) are presented. The upper part of Figure 2 shows the corresponding GFz-curves (Figure 2A), the lower part shows the temporal sequence of the steps (Figure 2B). The more affected the patient is, the slower he walks, the higher the variability of the individual steps and the less clear is the heel-on-midstance-toe off-modulation of the CFz-curves.
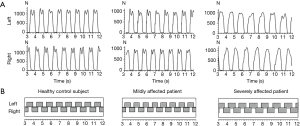
Temporal parameters of walking in WD-patients and control subjects
The duration of the swing phase of the left (SSWL) and the right leg (SSWR) were significantly (R: P<0.02; L: P<0.01) longer in the patients compared to those of the controls (Table 3), and there was a tendency for a longer double support (DSR, DSL) in the patients (Table 3). As a consequence derived measures as step time (STEP = SSW + DS of the contralateral leg) or stance time (STAN = SSW of the contralateral leg + DSR + DSL) were significantly (P<0.01) longer in the patients.
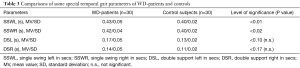
Full table
However, when all temporal parameters were time normalized (calculated in % of a gait cycle = STEPR + STEPL), no differences between patients and controls could be detected for temporal parameters: normalized stance time (TNSTAN), step time (TNSTEP), single swing (TNSSW), and double support (TNDS) were normal.
Correlation of temporal parameters with clinical scores in WD-patients
None of the temporal parameters showed a correlation with MotS. The total score TS showed a significant negative correlation (r=−0.461, P<0.012) with gait speed (Figure 3). There was a significant negative correlation (r=−0.547; P<0.001) between all three items of N-MotS as well as N-MotS itself and gait speed: the higher the non-motor score, the slower the patients walked. Furthermore, the more sensory deficits (item: sensory symptoms) the patients had, the longer the double support time was (DSR and DSL: r=0.593; P<0.001).
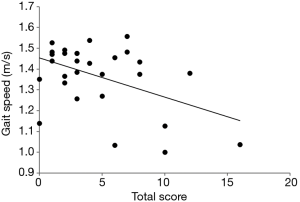
Correlation of temporal parameters with laboratory findings in WD-patients
Liver enzymes correlated with duration of swing time (SSWR, SSWL), stance time (STANR, STANL), and step time (STEPR, STEPL). The highest correlations were found for GPT [SSW (R + L): r=0.463, P<0.01; STAN (R + L): r=0.474, P<0.01; STEP (R + L): r=0.547, P<0.001]. But GPT, GOT and GGT were also inversely correlated with cadence (r=−0.515, P<0.001). This is demonstrated for GOT in Figure 4. The serum level of ceruloplasmin and the copper content in the 24 h urine were positively correlated with gait speed (r=0.394, P<0.01; r=0.414, P<0.003 respectively). The relationship between urinary copper content and gait speed was obviously non-linear. A fit of a logarithmic curve yielded a higher correlation (r=0.504; P<0.002) than a linear fit or Spearman’s rho. These correlations were calculated with the obviously exceptional case included (see Figure 5).
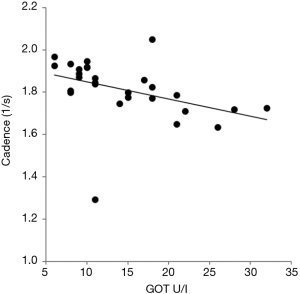
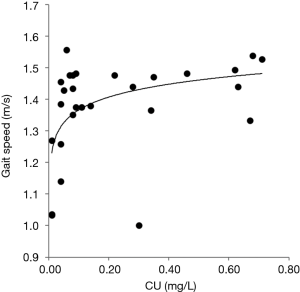
Discussion
Severity of symptoms in long-term treated WD-patients
For the interpretation of the results it is important to emphasize that patients were recruited from out-patients, were treated for 12 years in the mean and responded well to therapy. In full agreement with Burke et al. (9) who had observed a good response of initial gait abnormalities to therapy in WD-patients a low frequency of gait abnormalities [20%; (10)] was found in the present study. Compared to patients in a study on initial gait disturbances and falls in WD (13), patients in the present study were diagnosed about 14 years earlier in the mean, and therefore were less affected (9,18). Early diagnosis of WD and early onset of therapy appear to be crucial for the long-term outcome, especially the persistence of gait abnormalities.
Preserved temporal patterns of walking in WD
All relevant temporal parameters (STAN, STEP, SSW) in our cohort of long-term treated WD-patients were significantly longer than those of an age- and sex matched control group. However, in contrast to patients with hemispheric lesions after stroke with a highly asymmetric gait (22,23), the temporal pattern of walking appeared to be fairly normal in WD. After time normalization (compensating the influence of gait speed) the relative relationships between temporal gait parameters turned out to be completely normal.
The temporal pattern of locomotion in mammalians is controlled by general pattern generators (CPGs) in the spinal cord or the brain stem (24-26). The preservation of normal temporal relationships suggests that the CPGs involved in walking had not been affected in the present cohort of WD-patients.
Reduced compliance as possible cause for slowness of walking in WD
Gait speed showed a positive, significant relation to the copper concentration in the 24 h-urine under medication. This confirms that the compliance of the patients may play a major role for improvement of gait. In untreated patients, copper concentration in the 24 h-urine initially is high and continuously declines under treatment. It reaches a stable plateau reflecting a steady state between copper up-take and copper elimination. When the dose of copper eliminating drugs is reduced at that time, urinary copper concentration will decline, and the patient may develop symptoms again. Therefore, the positive correlation between gait speed and urinary copper concentration fits to the clinical observation that gait is sensitive to copper elimination therapy (9).
Reduction of copper elimination goes along with a significant increase of the total score and serum levels of liver enzymes (10). Therefore the significant inverse relationship between cadence and liver enzymes fits to the assumption of a reduced compliance during long-term treatment in WD leading to a secondary intoxication of liver and CNS (10). Cadence of natural walking appears to be highly sensitive to changes of liver enzymes (Figure 4). This is well-known from impaired walking in patients with hepatic encephalopathy (27,28), but liver dysfunction in our patients was so mild that none of the patients suffered from hepatic encephalopathy.
Clinical implications and conclusions
The results of the present study have immediate consequences for clinical practise. The significant positive (rank) correlation between gait speed and urinary copper concentration and the significant negative correlation between liver enzymes and cadence indicate that reduced compliance has relevant negative implications on functional outcome, especially on gait in WD. Therefore patients with WD should undergo careful neurological investigation and laboratory findings on a regular basis to detect neurological deterioration under therapy and to control patient’s compliance. This becomes increasingly important with duration of treatment since deterioration in spite of therapy may not only reflect reduced compliance but also the development of a hepatocellular carcinoma or another malignant process as we have observed in 5 out of 120 long-term treated WD-patients.
Limitations of the study
The Infotronic® Ultraflex gait analysis system does not allow the measurement of movements of parts of the body in space. Therefore, no angle measurements could be performed. On the other hand, measurements of toe and forefoot movements during walking are difficult to perform even with more complex gait analysis systems, especially with systems using active markers. Therefore the application of the Ultraflex system is a compromise since it can be handled easily.
Conclusions
The temporal pattern of gait is normal in WD. A compliance dependent reduction of gait speed and cadence underline the necessity of careful long-term therapy monitoring in WD.
Acknowledgements
None.
Footnote
Conflicts of Interest: O Tezayak and D Rosenthal have no conflicts of interest to declare. H Hefter has received a grant from the German Wilson disease patient group to support his studies on the compliance behaviour of WD-patients in detail.
Ethical Statement: The study was performed according to the 1964 Declaration of Helsinki and its later amendments and the guidelines for good clinical practise. All persons (patients and normal subjects) had been informed in detail on the purpose of the present study and had given informed consent prior to data recording and/or clinical examinations. Publication of anonymized clinical data after informed consent of patients with WD was approved by the local ethics committee of the University of Düsseldorf.
References
- Bull PC, Thomas GR, Rommens JM, et al. The Wilson disease gene is a putative copper transporting P-type ATPase similar to the Menkes gene. Nat Genet 1993;5:327-37. [Crossref] [PubMed]
- Petrukhin K, Fischer SG, Pirastu M, et al. Mapping, cloning and genetic characterization of the region containing the Wilson disease gene. Nat Genet 1993;5:338-43. [Crossref] [PubMed]
- Tanzi RE, Petrukhin K, Chernov I, et al. The Wilson disease gene is a copper transporting ATPase with homology to the Menkes disease gene. Nature Genet 1993;5:344-50. [Crossref] [PubMed]
- Cumings JN. The copper and iron content of brain and liver in the normal and in hepato-lenticular degeneration. Brain 1948;71:410-5. [Crossref] [PubMed]
- Ghika J, Vingerhoets F, Maeder P, et al. Maladie de Wilson. EMC-Neurologie 2004;1:481-511. [Crossref]
- Kuwert T, Hefter H, Scholz D, et al. Regional cerebral glucose consumption measured by positron emission tomography in patients with Wilson’s disease. Eur J Nucl Med 1992;19:96-101. [Crossref] [PubMed]
- Magalhaes ACA, Caramelli P, Menezes JR, et al. Wilson’s disease: MRI with clinical correlation. Neuroradiology 1994;36:97-100. [Crossref] [PubMed]
- Machado A, Chien HF, Deguti MM, et al. Neurological manifestations in Wilson’s disease: report of 119 cases. Mov Disord 2006;21:2192-6. [Crossref] [PubMed]
- Burke JF, Dayalu P, Nan B, et al. Prognostic significance of neurologic examination findings in Wilson disease. Parkinsonism Relat Disord 2011;17:551-6. [Crossref] [PubMed]
- Hefter H, Tezayak O, Rosenthal D. Long-term outcome of neurological Wilson’s disease. Parkinsonism Relat Disord 2018;49:48-53. [Crossref] [PubMed]
- Kirkham TH, Kamin DF. Slow saccadic eye movements in Wilson’s disease. J Neurol Neurosurg Psychiatry 1974;37:191-4. [Crossref] [PubMed]
- Ebert D, Georgas E, Rosenthal D, et al. Phase relations between rhythmical arm movements and breathing in Wilson’s disease. Adv Exp Med Biol 2010;669:231-4. [Crossref] [PubMed]
- Dzieżyc K, Litwin T, Chabik G, et al. Frequencies of initial gait disturbances and falls in 100 Wilson’s disease patients. Gait Posture 2015;42:601-3. [Crossref] [PubMed]
- Clark JE, Whitall J. Development of posture and gait across life span. Univ South Carol Press, 1989.
- Whitall J, Getchell N. Development of posture and gait across the life span. Child Dev 1995;66:1541-53. [Crossref] [PubMed]
- Giagheddu A, Demelia L, Puggioni G, et al. Epidemiologic study of hepatolenticular degeneration (Wilson’s disease) in Sardinia (1902-1983) Acta Neurol Scand 1985;72:43-55. [Crossref] [PubMed]
- Oder W, Grimm G, Kollegger H, et al. Neurological and neuropsychiatric spectrum of Wilson’s disease: a prospective study of 45 cases. J Neurol 1991;238:281-7. [PubMed]
- Hefter H, Arendt G, Stremmel W, et al. Motor impairment in Wilson’s disease I: slowness of voluntary limb movements. Acta Neurol Scand 1993;87:133-47. [Crossref] [PubMed]
- Hennerici M, Bäzner H. Gangstörungen. Berlin Heidelberg New York: Springer, 2001.
- Jeleń P, Wit A, Dudzinski K, et al. Expressing gait-line symmetry in able-bodied gait. Dyn Med 2008;7:17. [Crossref] [PubMed]
- Medici V, Mirante VG, Fassati LR, et al. Liver transplantation for Wilson’s disease: The burden of neurological and psychiatric disorders. Liver Transpl 2005;11:1056-63. [Crossref] [PubMed]
- Patterson KK, Parafianowicz I, Danells CJ, et al. Gait asymmetry in community-ambulating stroke survivors. Arch Phys Med Rehabil 2008;89:304-10. [Crossref] [PubMed]
- Patterson KK, Gage WH, Brooks D, et al. Evaluation of gait symmetry after stroke: A comparison of current methods and recommendations for standardization. Gait Posture 2010;31:241-6. [Crossref] [PubMed]
- Grillner S. Control of locomotion in bipeds, tetrapods, and fish: In: Brookhart JM, Mountcastle VB. editors. Handbook of physiology – the nervous system II. American Physiological Society, Bethesda, 1981:1179-236.
- Duysens J, Van de Crommert HW. Neural control of locomotion, part 1: The central pattern generator from cats to humans. Gait Posture 1998;7:131-41. [Crossref] [PubMed]
- Capaday C. (The special nature of human walking and its neural control. Trends Neurosci 2002;25:370-6. [Crossref] [PubMed]
- Sherlock S, Summerskill WH, White LP, et al. Portal-systemic encephalopathy. Neurological complications of liver disease. Lancet 1954;267:454-7. [PubMed]
- Weissenborn K, Ennen JC, Schomerus H, et al. neuropsychological characterization of hepatic encephalopathy. J Hepatol 2001;34:768-73. [Crossref] [PubMed]