FK506 prevented bone loss in streptozotocin-induced diabetic rats via enhancing osteogenesis and inhibiting adipogenesis
Introduction
Type 1 diabetes mellitus (T1DM) is a common systemic disease worldwide. It has detrimental effects on the skeleton, resulting several bone diseases, including osteoporosis, osteopenia, bone fractures, and impaired bone healing and regeneration (1). An epidemiologic study demonstrated that patients with T1DM are at higher fracture risk: hip fracture risk is elevated (RR =6.94, 95% confidence interval, 3.25–14.78) compared to the risk in persons without diabetes (2). Nevertheless, the link between diabetes mellitus (DM) and skeletal health receives only cursory attention in osteoporosis, and an effective treatment is lacking.
Osteoblasts and adipocytes differentiate from a common precursor cell in bone marrow, mesenchymal stem cells (MSCs) (3,4). The pluripotency of MSCs is well known, and their capacity to differentiate into osteoblasts, adipocytes, and chondrocytes has been described extensively (5,6). There is an inverse relationship between the differentiation of MSCs to osteoblasts and adipocytes, as the osteogenic differentiation of MSCs requires a coordinated inhibition of adipogenic differentiation (6,7). Consistent with this reciprocal relationship, multiple studies have also confirmed the correlations of high marrow fat content and low bone mass with increased fracture risk, particularly in the context of T1DM (8,9). Increased marrow adipose tissue accumulation occurs at the expense of bone formation and in turn impairs osteogenic regeneration (10). Thus, if a therapy can influence MSC differentiation by promoting osteoblast formation and inhibiting adipocyte production, it will likely be a good candidate for the treatment of bone loss in patients with T1DM.
FK506, also called tacrolimus or fujimycin, is a macrolide antibiotic produced by Streptomyces tsukubaensis and is known as a strong immunosuppressant. FK506 was first described in 1987 (11) and was introduced into clinical trials in 1989. The drug has been applied in organ transplantation (12,13), atopic dermatitis (14), rheumatoid arthritis (15,16), and diabetes (17,18). FK506 has an immunosuppressive effect by interfering with T cell activation through the inhibition of calcineurin. Studies have elaborated that transplant patients treated with FK506 often develop osteoporosis symptoms or bone fracture (13,19). The reason might be that FK506 could cause increased parathyroid hormone secretion, which increased osteoclast formation. Besides, FK506 could also increase bone resorption directly. Kang et al. (20) demonstrated that FK506 inhibits bone erosion by increasing bone formation in rheumatoid arthritis by targeting both osteoblasts and T cells via the inhibition of the calcineurin/nuclear factor of activated T cells (NFAT) pathway. Folwarczna et al. (21) suggested that low dose of FK506 (0.3 mg/kg/day) did not affect skeletal system in healthy male rats. However, Rubert et al. (22) showed that high dose of FK506 (3 mg/kg/day) produced osteopenia in healthy male rats. Above all, the skeletal effects of FK506 on several non-diabetic diseases have been elaborated, and the skeletal effects of FK506 vary in different diseases. However, the effects of FK506 on the skeleton in T1DM have not been clarified. This study aimed to investigate the influences of FK506 on the balance of osteogenesis and adipogenesis in streptozocin (STZ)-induced diabetic rats, particularly to assess changes in skeletal parameters.
Methods
Animals
The institutional animal care and use committee of Southeast University (Nanjing, China) authorized our study (approval No. 20170926007). Male Sprague-Dawley rats (Animal Laboratory of Shanghai Sippr-BK, China) at the age of 8 weeks were randomly divided to 3 groups: a control (CTL) group, a DM group and a DM+FK506 group (n=10, respectively). FK506 (Astellas Ireland Co., Ltd., Dublin, Ireland) was orally administered once daily (0.15 mg/kg/d). DM was induced by a single intraperitoneal injection of STZ (s0130, Sigma) at the dose of 58 mg/kg. The rats were sacrificed at 32 weeks after diabetes induction. Blood, urine, tibias, femurs and lumbar vertebrae were collected for our study.
Serum biochemistry
Blood urea nitrogen (BUN), serum creatinine (Scr), and phosphate and total calcium concentrations were detected by a semiautomatic biochemical analyzer (ECA-2000A) and a UV-5100 spectrophotometer. Blood glucose was determined using an automatic glucometer (Bayer Medical, Germany). Urinary protein levels were determined by a protein assay kit through the modified Bradford method (KeyGen).
H&E stain
Bone masses were fixed in 10% neutral buffered formalin for 24 h. They were then decalcified in 10% (w/v) ethylenediaminetetraacetic acid (EDTA) (G1105, Servicebio) for 3 weeks and embedded in paraffin. Sections were stained with H&E (G1005, Servicebio) after being deparaffinized and rehydrated according to routine protocol (23). Briefly, after the specimens were deparaffinized and rehydrated, 4 µm sections were mounted on slides and stained with hematoxylin solution for 5 minutes and then washed in the distilled water for 1 minute. Next, the sections were stained with eosin solution for 2 minutes and were washed in the distilled water for 1 minute. The sections were then dehydrated with graded alcohol and cleared in xylene. Finally, the mounted slides were examined, and images were taken through a light microscope (Olympus, Tokyo).
Oil red O stain
The femurs were fixed in 4% paraformaldehyde, decalcified in 10% (w/v) EDTA (G1105, Servicebio) for 3 weeks, and then embedded. Sections were mounted on slides and stained with oil red O (G1016, Servicebio) for 15 minutes and then washed in distilled water for 1 minute. Next the sections were stained with eosin solution for 2 minutes and then washed in distilled water for 1 minute. Finally, the mounted slides were examined, and images were taken through a light microscope (Olympus, Tokyo). Adipogenesis was assessed in the medullary cavity.
Dual energy X-ray absorptiometry (DEXA)
The bone mineral density (BMD) was detected by DEXA through a bone mineral analyzer (HOLOGIC QDR series, America) in Southeast University-affiliated Zhongda Hospital. All animals were anesthetized with 10% chloral hydrate (302-17-0, Crystal Chemical) before the in vivo scan was performed. The BMD of the femurs and lumbar vertebrae were measured based on the small animal pattern.
Microcomputed tomography (micro-CT)
Micro-CT (SkyScan 1176, Bruker) was used to determine trabecular bone volume (bone volume/tissue volume, BV/TV), architecture (thickness, number and spacing) and cortical bone geometry (thickness and area). Femora and lumbar vertebrae were scanned at a resolution of 18 µm. The X-ray tube potential of 65 kV and current of 0.38 mA were established for all the animals. The volume of interest (VOI) was set as previously described (24). CtAnalyser (SkyScan software) was used for data analysis. Three-dimensional images were acquired through CtVox (SkyScan software). The reported micro-CT parameters were based on the international guidelines (25).
Bone mechanics
Structural mechanical properties and apparent material properties of the fifth lumbar (L5) vertebra were obtained by uniaxial compression testing (Instron 5943, America). Before mechanical testing, the vertebral arch and endplates were removed. Samples were loaded at a rate of 0.5 mm/minute to generate a force-displacement curve, and structural mechanical properties were determined through these curves as previously described (26).
Western blot analysis
Proteins obtained from each lysate were electrophoresed on SDS-polyacrylamide gels and transferred onto a PVDF membrane (60183-208, Thermo Fisher Scientific). Blots were incubated with corresponding primary antibodies against C/EBP-α (sc-365318, Santa Cruz), PPAR-γ (ab-209350, Abcam), Osterix (sc-393325, Santa Cruz), Runx2 (sc-101145, Santa Cruz), and β-catenin (ab-32572, Abcam). Signals were determined by an advanced ECL system (GE Healthcare). Finally, the immunoreactive bands were determined via densitometry through Image J software (NIH, Bethesda, USA).
Statistical analysis
Chi-square test was used for counting data. The measurement data are described as mean ± standard deviation (SD). If the data conformed to the parametric test, then the differences among groups were compared by Bonferroni test for post-hoc analysis after one-way ANOVA test. Otherwise, a non-parametric test (such as rank-sum test) was used for analysis. SPSS 21.0 software was used for all statistical analysis. A difference was considered significant if P<0.05.
Results
Animal biochemical measurements
As shown in Table 1, no significant difference in glucose levels was observed between the DM group and the DM + FK506 group, the levels of which were significantly higher than those in the CTL group. The levels of Scr and BUN were increased in the DM group and DM + FK506 group compared with those in the CTL group. Serum phosphorus and calcium levels were comparable among the 3 groups. The expression level of 24 h urinary protein was remarkably higher (+200%) in the DM group than that in the CTL group, and tended to decrease (−31%) after FK506 treatment.
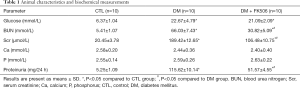
Full table
FK506 treatment increases BMD
The BMD measurements are shown in Table 2. The BMD in the DM group was significantly decreased (femur: −17.3%; lumbar vertebra: −23.1%) compared with that in the CTL group and tended to improve (femur: +28.3%; lumbar vertebra: +36.7%) after FK506 treatment.

Full table
FK506 treatment improves bone trabeculae by micro-CT assessment
Micro-CT assessments of bone parameters are shown in Table 3. In the distal femur, BV/TV (−45%), trabecular thickness (Tb.Th, −14%), and trabecular number (Tb.N, −48%) were lower, and trabecular separation (Tb.Sp, +163%) was higher in the DM group than their normal controls. FK506 administration tended to increase BV/TV (+99%) and Tb.Th (+23%), Tb.N (+121%) but decrease Tb.Sp (−69%) in the DM rats.
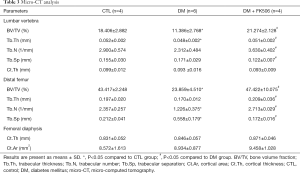
Full table
In the femoral diaphysis, cortical area (Ct.Ar) and cortical thickness (Ct.Th) were comparable among the study groups.
In the lumbar vertebra, BV/TV (−38%) and Tb.Th (–8%) were decreased in the DM group compared with those in the CTL group. FK506 administration tended to increase BV/TV (+87%), Tb.Th (+6%) and Tb.N (+57%) but decrease Tb.Sp (−29%) in the DM rats.
Three-dimensional images are shown in Figure 1.
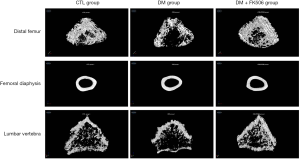
FK506 treatment improves bone mechanical properties
As shown in Table 4, vertebra compression tests showed that DM animals had lower ultimate force (−62%) and work to failure (−48%) than normal animals did, which tended to be alleviated after FK506 treatment (+171% for ultimate force; +87% for work to failure).
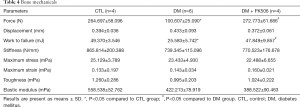
Full table
Effects of FK506 on bone histopathology
H&E staining of the rat tibia sections revealed the following (Figure 2A). Rats in the CTL group exhibited well-formed and connected bone with normal thickness. Femur bone tissue from the DM group exhibited widely separated, uniform thinning of the trabeculae, suggesting osteoporosis. The FK506 treatment group demonstrated a well-formed, benign-looking, joined bone trabeculae.
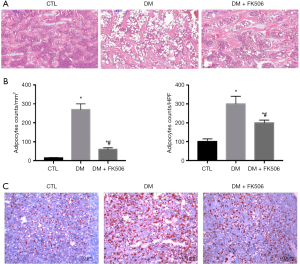
In addition, the infiltration of bone marrow fat was determined by H&E staining and oil red O staining (Figure 2A,B,C,D) of the bone sections. The data suggest that rats in the DM group had increased bone marrow fat, which was decreased after FK506 treatment. These data indicated that FK506 improved bone histopathology in the DM rats.
FK506 upregulates the expression levels of osteogenesis markers and downregulates the expression levels of adipogenesis markers
A seen from Figure 3, the expression levels of osteogenesis markers (Runx2, Osterix) were significantly decreased in the DM group compared with their normal controls. In contrast, the expression levels of adipogenesis markers (PPAR-γ, C/EBP-α) were remarkably enhanced in the DM group compared with those in the CTL group. However, these changes were alleviated after FK506 therapy.
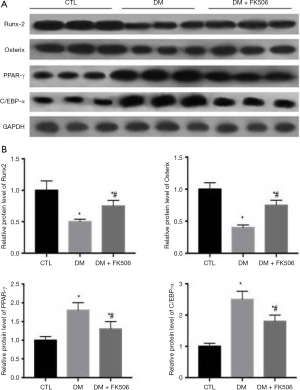
FK506 increases the expression of nuclear translocation of β-catenin
To acquire further understanding about the mechanisms involved in the balance between osteoblastogenesis and adipogenesis, we examined the nuclear expression of β-catenin in the study groups (Figure 4). As shown by western blotting, the nuclear translocation of β-catenin was decreased in the DM group compared with their normal animals but was increased after FK506 treatment.

Discussion
Bone loss is a known negative consequence in T1DM. The imbalance between osteogenesis and adipogenesis is an important mechanism of osteoporosis in T1DM (6,7). Here, we used a STZ-induced diabetic rat model to examine the skeletal effects of FK506 treatment in T1DM. We found the following findings: (I) FK506 attenuates diabetes-induced bone loss; (II) FK506 increases osteogenesis and decreases adipogenesis; and (III) FK506 might regulate the bone-fat balance via the Wnt/β-catenin signaling pathway.
The diabetic population is a high-risk group for osteoporosis, but even in developed countries, there have been few systematic studies of diabetes-induced osteoporosis. In this study, we thoroughly examined the skeletal system of study animals via multiple methods such as DEXA, micro-CT, bone mechanics and bone histology. Data from our laboratory showed that DM rats have decreased BMD, trabecular bone loss, reduced ultimate force and work to failure, devastating bone histomorphology and increased bone marrow fat compared with these data in the CTL rats. These observations are similar to the bone loss reported in other STZ-induced diabetic rats (8,27,28). FK506, an immunosuppressant, significantly increased BMD, reduced trabecular bone loss, improved ultimate force and work to failure, improved bone histomorphology and decreased bone marrow fat in diabetic rats in our study. Interestingly, our data confirmed that FK506 attenuated bone loss in regions of trabecular bone but was not statistically significant in cortical bone, possibly because trabecular bone is more metabolically active than cortical bone is (9). In addition, the expression levels of bone-related markers (Runx2, Osterix) were decreased in the DM group compared with their normal controls, while the expression of adipocyte-related markers (PPAR-γ, C/EBP-α) were increased. FK506 attenuated these changes. Runx2 is important for the differentiation of MSCs into osteoblasts and inhibits differentiation into adipocytes and chondrocytes (29,30). Osterix functions downstream of Runx2 for inducing osteoblastic differentiation (31,32). PPAR-γ and C/EBP-α family proteins are major transcription factors that directly affect preadipocyte proliferation and differentiation (33,34). These data suggest that FK506 attenuates bone loss by increasing osteogenesis and decreasing adipogenesis in diabetic rats.
The beneficial effects of FK506 on the skeleton in diabetic rats present an interesting finding. The role of FK506 in osteoblasts is ambiguous. Krocker et al. (35) suggested that FK506 (10 ng/mL) had no effects on cell proliferation but upregulated the osteogenic markers of human osteoblasts. Koga et al. (36) elaborated that FK506 decreased bone formation in osteoblasts. The role of FK506 in osteoclasts is also complex. Igarashi et al. (37) suggested that FK506 induced osteoclast apoptosis. Miyazaki et al. (38) showed that FK506 inhibited human osteoclast formation in rheumatoid arthritis via targeting the calcineurin-depengd NFAT pathway and an activation pathway for c-Jun or microphthalmia transcription factor. Besides these bone cells, bone marrow derived MSCs and bone marrow adipocytes have been increasingly popular in diabetic osteoporosis. Isomoto et al. (39) revealed that FK506 (10−8 mol/L) exhibited an osteogenic effect on MSCs. The effects of FK506 on bone marrow adipocytes remained unclear. Further than that, we reviewed the clinical and animal studies: posttransplantation patients treated with immunosuppressants often developed osteopenic conditions or bone fractures (13,19). Osteopenia related to intramuscular injection of FK506 (1 mg/kg for 28 consecutive days) has also been observed in rats (40). Thus, we hypothesized that various factors, such as concentration and duration of the drug treatment, the target tissues or cells, the model species and disease conditions, can all influence the effects of FK506 on skeletal metabolism, which still need further research. Above all, the skeletal effects of FK506 is complex, and the in vitro could not keep consistent with the in vivo studies. Our vivo study showed that FK506 has a beneficial effect on diabetic osteoporosis by suppressing adipogenesis and increasing osteogenesis, which further expands the applications for FK506 treatment. However, greater sample sizes and more clinical studies are required to fully determine the safety and efficacy of FK506 for diabetic osteoporosis.
The mechanism of action of FK506 on the skeleton in diabetic rats is still largely unclear. Previous studies demonstrated that the Wnt/β-catenin pathway plays a vital role in the pathogenesis of diabetes-induced bone loss (41-44). Wnts signal through frizzled and LRP5/6 coreceptors, leading to inactivation of the axin-GSK3-β complex, which phosphorylates and directs the degradation of β-catenin. Stabilized β-catenin translocates into the nucleus to activate Wnt target genes (45,46). The Wnt pathway mediates certain transcription factors expression, such as Runx2 and Osterix, which strongly enhances osteoblastogenesis (46,47). On one hand, Wnt/β-catenin pathway could mediate the MSC fate by increasing osteogenesis and inhibiting adipogenesis. Enhanced Wnt signaling either by Wnt overexpression (48) or deficiency (49) in Wnt antagonists is associated with increased bone formation in animals and humans. On the other hand, WNT1 regulates osteoblast function during bone homeostasis (50). And deletion of Wnt1 in osteocytes leads to reduced bone mass with increased fractures (50). In our study, FK506 dramatically promotes nuclear translocation of β-catenin in DM rats, suggesting that FK506 therapy might promote osteoblastogenesis through activation of the Wnt/β-catenin pathway, which needed to be further studied.
Interestingly, several reports have demonstrated a significant increase in blood glucose after FK506 treatment (51,52). The mechanism for these diabetogenic effects may be that FK506 inhibits the function of pancreatic β-cells (53). However, our experiment did not detect elevated blood glucose levels. It is possible that the dose of FK506 used in the current study is low, and the β-cells had already been destroyed by STZ in diabetic rats.
In conclusion, this study demonstrated that FK506 has a beneficial effect on the skeleton in diabetic rats, making it a promising candidate for treating and preventing diabetic osteoporosis, which may be regulated by the canonical Wnt/β-catenin pathway.
Acknowledgments
Funding: This study was funded by grants from the National Natural Science Foundation of China (31571186, 81770735, 81370919, 81470997), The Medical Talents of Jiangsu Province (ZDRCA2016079), the Natural Science Foundation of Jiangsu Province (BK20161437), Clinical Medicine Science and Technology Project-Clinic Research Center of Jiangsu Province (BL2014080), and Jiangsu Commission of Health “Six One Project” (LGY2018097).
Footnote
Conflicts of Interest: The authors have no conflicts of interest to declare.
Ethical Statement: The institutional animal care and use committee of Southeast University (Nanjing, China) authorized our study (approval No. 20170926007).
References
- Starup-Linde J, Vestergaard P. Management of endocrine disease: Diabetes and osteoporosis: cause for concern? Eur J Endocrinol 2015;173:R93-9. [Crossref] [PubMed]
- Vestergaard P. Discrepancies in bone mineral density and fracture risk in patients with type 1 and type 2 diabetes--a meta-analysis. Osteoporos Int 2007;18:427-44. [Crossref] [PubMed]
- Minguell JJ, Erices A, Conget P. Mesenchymal stem cells. Exp Biol Med (Maywood) 2001;226:507-20. [Crossref] [PubMed]
- Muruganandan S, Roman AA, Sinal CJ. Adipocyte differentiation of bone marrow-derived mesenchymal stem cells: cross talk with the osteoblastogenic program. Cell Mol Life Sci 2009;66:236-53. [Crossref] [PubMed]
- Gharibi B, Abraham AA, Ham J, et al. Adenosine receptor subtype expression and activation influence the differentiation of mesenchymal stem cells to osteoblasts and adipocytes. J Bone Miner Res 2011;26:2112-24. [Crossref] [PubMed]
- Kawai M, Rosen CJ. PPARgamma: a circadian transcription factor in adipogenesis and osteogenesis. Nat Rev Endocrinol 2010;6:629-36. [Crossref] [PubMed]
- McCauley LK. c-Maf and you won't see fat. J Clin Invest 2010;120:3440-2. [Crossref] [PubMed]
- Botolin S, McCabe LR. Bone loss and increased bone adiposity in spontaneous and pharmacologically induced diabetic mice. Endocrinology 2007;148:198-205. [Crossref] [PubMed]
- Botolin S, Faugere MC, Malluche H, et al. Increased bone adiposity and peroxisomal proliferator-activated receptor-gamma2 expression in type I diabetic mice. Endocrinology 2005;146:3622-31. [Crossref] [PubMed]
- Ambrosi TH, Scialdone A, Graja A, et al. Adipocyte Accumulation in the Bone Marrow during Obesity and Aging Impairs Stem Cell-Based Hematopoietic and Bone Regeneration. Cell Stem Cell 2017;20:771-84.e6. [Crossref] [PubMed]
- Kino T, Hatanaka H, Miyata S, et al. FK-506, a novel immunosuppressant isolated from a Streptomyces. II. Immunosuppressive effect of FK-506 in vitro. J Antibiot (Tokyo) 1987;40:1256-65. [Crossref] [PubMed]
- Rodino MA, Shane E. Osteoporosis after organ transplantation. Am J Med 1998;104:459-69. [Crossref] [PubMed]
- Stein E, Ebeling P, Shane E. Post-transplantation osteoporosis. Endocrinol Metab Clin North Am 2007;36:937-63. [Crossref] [PubMed]
- Ohtsuki M, Morimoto H, Nakagawa H. Tacrolimus ointment for the treatment of adult and pediatric atopic dermatitis: Review on safety and benefits. J Dermatol 2018;45:936-42. [Crossref] [PubMed]
- Takeuchi T, Ishida K, Shiraki K, et al. Safety and effectiveness of tacrolimus add-on therapy for rheumatoid arthritis patients without an adequate response to biological disease-modifying anti-rheumatic drugs (DMARDs): Post-marketing surveillance in Japan. Mod Rheumatol 2018;28:48-57. [Crossref] [PubMed]
- Ito A, Okada Y, Hashita T. Sex Differences in the Blood Concentration of Tacrolimus in Systemic Lupus Erythematosus and Rheumatoid Arthritis Patients with CYP3A5*3/*3. Biochem Genet 2017;55:268-77. [Crossref] [PubMed]
- Qi XM, Wang J, Xu XX, et al. FK506 reduces albuminuria through improving podocyte nephrin and podocin expression in diabetic rats. Inflamm Res 2016;65:103-14. [Crossref] [PubMed]
- Su L, Ji J, Bian J, et al. Tacrolimus (FK506) prevents early retinal neovascularization in streptozotocin-induced diabetic mice. Int Immunopharmacol 2012;14:606-12. [Crossref] [PubMed]
- Kulak CA, Borba VZ, Kulak J Junior, et al. Post-transplantation osteoporosis. Arq Bras Endocrinol Metabol 2010;54:143-9. [Crossref] [PubMed]
- Kang KY, Ju JH, Song YW, et al. Tacrolimus treatment increases bone formation in patients with rheumatoid arthritis. Rheumatol Int 2013;33:2159-63. [Crossref] [PubMed]
- Folwarczna J, Kaczmarczyk-Sedlak I, Pytlik M, et al. Effect of low-dose tacrolimus coadministered with raloxifene on the skeletal system in male rats. Acta Pol Pharm 2009;66:207-12. [PubMed]
- Rubert M, Montero M, Guede D, et al. Sirolimus and tacrolimus rather than cyclosporine A cause bone loss in healthy adult male rats. Bone Rep 2015;2:74-81. [Crossref] [PubMed]
- Liu H, Zhu R, Liu C, et al. Evaluation of Decalcification Techniques for Rat Femurs Using HE and Immunohistochemical Staining. Biomed Res Int 2017;2017:9050754. [PubMed]
- Ni LH, Tang RN, Lv LL, et al. A rat model of SHPT with bone abnormalities in CKD induced by adenine and a high phosphorus diet. Biochem Biophys Res Commun 2018;498:654-9. [Crossref] [PubMed]
- Bouxsein ML, Boyd SK, Christiansen BA, et al. Guidelines for assessment of bone microstructure in rodents using micro-computed tomography. J Bone Miner Res 2010;25:1468-86. [Crossref] [PubMed]
- Campbell GM, Tiwari S, Hofbauer C, et al. Effects of parathyroid hormone on cortical porosity, non-enzymatic glycation and bone tissue mechanics in rats with type 2 diabetes mellitus. Bone 2016;82:116-21. [Crossref] [PubMed]
- Shyng YC, Devlin H, Sloan P. The effect of streptozotocin-induced experimental diabetes mellitus on calvarial defect healing and bone turnover in the rat. Int J Oral Maxillofac Surg 2001;30:70-4. [Crossref] [PubMed]
- Motyl K, McCabe LR. Streptozotocin, type I diabetes severity and bone. Biol Proced Online 2009;11:296-315. [Crossref] [PubMed]
- Liu TM, Lee EH. Transcriptional regulatory cascades in Runx2-dependent bone development. Tissue Eng Part B Rev 2013;19:254-63. [Crossref] [PubMed]
- Ducy P. Cbfa1: a molecular switch in osteoblast biology. Dev Dyn 2000;219:461-71. [Crossref] [PubMed]
- Nakashima K, Zhou X, Kunkel G, et al. The novel zinc finger-containing transcription factor osterix is required for osteoblast differentiation and bone formation. Cell 2002;108:17-29. [Crossref] [PubMed]
- Zhang C. Transcriptional regulation of bone formation by the osteoblast-specific transcription factor Osx. J Orthop Surg Res 2010;5:37. [Crossref] [PubMed]
- Zhuang H, Zhang X, Zhu C, et al. Molecular Mechanisms of PPAR-gamma Governing MSC Osteogenic and Adipogenic Differentiation. Curr Stem Cell Res Ther 2016;11:255-64. [Crossref] [PubMed]
- James AW. Review of Signaling Pathways Governing MSC Osteogenic and Adipogenic Differentiation. Scientifica (Cairo) 2013;2013:684736. [Crossref] [PubMed]
- Krocker D, Perka C, Tuischer J, et al. Effects of tacrolimus, cyclosporin A and sirolimus on MG63 cells. Transpl Int 2006;19:563-9. [Crossref] [PubMed]
- Koga T, Matsui Y, Asagiri M, et al. NFAT and Osterix cooperatively regulate bone formation. Nat Med 2005;11:880-5. [Crossref] [PubMed]
- Igarashi K, Hirotani H, Woo JT, et al. Cyclosporine A and FK506 induce osteoclast apoptosis in mouse bone marrow cell cultures. Bone 2004;35:47-56. [Crossref] [PubMed]
- Miyazaki M, Fujikawa Y, Takita C, et al. Tacrolimus and cyclosporine A inhibit human osteoclast formation via targeting the calcineurin-dependent NFAT pathway and an activation pathway for c-Jun or MITF in rheumatoid arthritis. Clin Rheumatol 2007;26:231-9. [Crossref] [PubMed]
- Isomoto S, Hattori K, Ohgushi H, et al. Rapamycin as an inhibitor of osteogenic differentiation in bone marrow-derived mesenchymal stem cells. J Orthop Sci 2007;12:83-8. [Crossref] [PubMed]
- Fukunaga J, Yamaai T, Yamachika E, et al. Expression of osteoclast differentiation factor and osteoclastogenesis inhibitory factor in rat osteoporosis induced by immunosuppressant FK506. Bone 2004;34:425-31. [Crossref] [PubMed]
- Antonopoulou M, Bahtiyar G, Banerji MA, et al. Diabetes and bone health. Maturitas 2013;76:253-9. [Crossref] [PubMed]
- Bennett CN, Longo KA, Wright WS, et al. Regulation of osteoblastogenesis and bone mass by Wnt10b. Proc Natl Acad Sci U S A 2005;102:3324-9. [Crossref] [PubMed]
- Gaur T, Lengner CJ, Hovhannisyan H, et al. Canonical WNT signaling promotes osteogenesis by directly stimulating Runx2 gene expression. J Biol Chem 2005;280:33132-40. [Crossref] [PubMed]
- Gong Y, Slee RB, Fukai N, et al. LDL receptor-related protein 5 (LRP5) affects bone accrual and eye development. Cell 2001;107:513-23. [Crossref] [PubMed]
- Reya T, Clevers H. Wnt signalling in stem cells and cancer. Nature 2005;434:843-50. [Crossref] [PubMed]
- Liu G, Vijayakumar S, Grumolato L, et al. Canonical Wnts function as potent regulators of osteogenesis by human mesenchymal stem cells. J Cell Biol 2009;185:67-75. [Crossref] [PubMed]
- Meng J, Ma X, Wang N, et al. Activation of GLP-1 Receptor Promotes Bone Marrow Stromal Cell Osteogenic Differentiation through β-Catenin. Stem Cell Reports 2016;6:579-91. [Crossref] [PubMed]
- Bennett CN, Ouyang H, Ma YL, et al. Wnt10b increases postnatal bone formation by enhancing osteoblast differentiation. J Bone Miner Res 2007;22:1924-32. [Crossref] [PubMed]
- ten Dijke P, Krause C, de Gorter DJ, et al. Osteocyte-derived sclerostin inhibits bone formation: its role in bone morphogenetic protein and Wnt signaling. J Bone Joint Surg Am 2008;90 Suppl 1:31-5. [Crossref] [PubMed]
- Joeng KS, Lee YC, Lim J, et al. Osteocyte-specific WNT1 regulates osteoblast function during bone homeostasis. J Clin Invest 2017;127:2678-88. [Crossref] [PubMed]
- Hoitsma AJ, Hilbrands LB. Relative risk of new-onset diabetes during the first year after renal transplantation in patients receiving tacrolimus or cyclosporine immunosuppression. Clin Transplant 2006;20:659-64. [Crossref] [PubMed]
- Furth S, Neu A, Colombani P, et al. Diabetes as a complication of tacrolimus (FK506) in pediatric renal transplant patients. Pediatr Nephrol 1996;10:64-6. [Crossref] [PubMed]
- Heit JJ, Apelqvist AA, Gu X, et al. Calcineurin/NFAT signalling regulates pancreatic beta-cell growth and function. Nature 2006;443:345-9. [Crossref] [PubMed]