Effect of miRNA-19a antisense oligonucleotide and Ara-C on the proliferation and apoptosis of HL60 cells
Introduction
In China, leukemia has high morbidity and mortality, the incidence of myeloid leukemia is higher than that of lymphocytic leukemia, and both significantly threaten human life. MicRNAs are a group of endogenous non-coding small RNAs, and can regulate the expression of nearly 60% of genes responsible for the cell proliferation, differentiation, apoptosis and other physiological processes (1). In a wide variety of tumors, micRNAs have similar functions to oncogenes or tumor suppressor genes (2,3) and may affect the initiation and development of tumors. It has been reported that micRNAs are expressed in a wide variety of tumors and play important roles in the classification, diagnosis and prognosis assessment of leukemia. There is evidence showing that miRNAs are closely associated with acute myelocytic leukemia (AML) and expected to become the potential therapeutic targets of AML (4,5).
MiR-17-92 cluster is a representative of proto-oncogene miRNAs and its expression increases in lymphoma, leukemia, multiple myeloma (MM) and other hematopoietic system tumors (6). MicroRNA-19 is a member of miR-17-92 cluster and can promote the proliferation of tumor cells and inhibit their apoptosis (7). In recent years, studies have shown that inhibition of carcinoid tumor gene expression may become a new and effective anti-tumor therapy (3,8). In 2015, Feng et al. down-regulated the expression of micRNA-19a in MM RPMI-8266 cells, which effectively inhibited cell vitality, invasion and cloning, providing a potential target for the treatment of MM (9). Inhibition of micRNA-19 expression in B lymphocytes is able to inhibit the proliferation of B lymphocytes (10). MiRNA-19, as a member of miR-17-92 cluster that can promote tumor formation (11,12), is closely related to the growth, metastasis and prognosis of MM, leukemia, lymphoma and other hematological malignancies, but little is known about the relationship between microRNA19a (miR-19a) and AML. In addition, micRNA-19 is also involved in the regulation of sensitivity of tumor cells to chemotherapy (13). In this study, the effects of miR-19a alone or in combination with Ara-C on the proliferation and apoptosis of HL60 cells were investigated. Our findings may provide evidence on the role of miR-19a in the pathogenesis of AML and present a potential targeted treatment for it.
Methods
Materials
HL60 cells were purchased from Shanghai Cell Bank Research Institute. IMDM was purchased from HyClone (USA). Lipofectamine 2000 Reagent was purchased from Invitrogen (USA). The CCK-8 Kit was purchased from Tongren Pharmaceutical Co. Ltd (Japan). The fluorescence quantitative PCR kit was purchased from RiboBio Co. Ltd (China). Antibodies and Hoechst33258 were purchased from Abcam (USA). The antisense oligonucleotide (ASODN) and scrambled oligonucleotide (SODN) were synthesized in Shanghai GeneChem Co. Ltd. (China). MiRNA-19a ASODN: 5'-UCAGUUUUGCAUAGAUUUGCACA-3'; scrambled sequence: 5'-CAGUACUUUUGUGUAGUACAA-3'.
Cell culture and grouping
HL60 cells were maintained in IMDM containing 15% fetal bovine serum (FBS), 100 U/mL penicillin, and 100 U/mL streptomycin in a humidified environment with 5% CO2 at 37 °C. The medium was refreshed once every 2–3 days, and cells in logarithmic growth phase were used in following experiments. In experiment 1, cells were divided into four groups: blank control group, blank transfection group, SODN group, and ASODN group. In experiment 2, cells were divided into Ara-C group, SODN + Ara-C group and ASODN + Ara-C group.
Cell transfection
Transfection was performed in accordance with the manufacturer’s instructions of Lipofectamine 2000 reagent. In the blank control group, cells were not treated; in the blank transfection group, cells were treated by Lipofectamine 2000 reagent alone; in the SODN group, cells were transfected with SODN; in the ASODN group, cells were transfected with ASODN.
Fluorescence real-time quantitative PCR
In experiment 1, cells were collected after transfection for 48 and 72 h, and RNA was extracted using the TRIzol reagent. The first strand cDNA was synthesized by reverse transcription using random primers and a reverse transcription kit. MiR-19a expression was detected using the SYBR Green I dye with U6 as the internal control. The total volume of reaction mixture was 20 µL. The reaction was performed at 95 °C for 10 min for pre-denaturation followed by 40 cycles of 95 °C for 2 s, 60 °C for 30 s and 70 °C for 8 s. The plate was read once at 70 °C. Then, the fluorescence intensity was recorded once every 5 s from 70 to 95 °C at a rate of 0.5 °C/s to obtain the melting curve. The relative expression was calculated using the relative quantitative formula: 2–∆Ct×100%, ∆Ct = Ct(miR-20a) - Ct(U6).
CCK8 assay
Cells in the logarithmic growth phase were seeded onto 96-well plates and then transfected. In experiment 1, cells were harvested after transfection for 48 h and 72 h; in experiment 2, cells were collected at 48 and 72 h. For CCK8 assay, 10 µL of CCK8 solution was added to each well, followed by incubation for 4 h. The absorbance at 450 nm was measured in a microplate reader. The A450 indirectly reflects cell survival. In the miR-19a ASODN + Ara-C group, cells were transfected with miR-19a ASODN and then incubated with 0.9 µmol/L for 48 and 72 h. The A450 was measured as above. The proliferation inhibition rate calculated as follow: cell proliferation inhibition rate (%) = (1-A450treatment/A450control) ×100%.
Hoechst staining
In experiment 1, cells were collected after 48-h transfection; in experiment 2, cells were harvested at 72 h. After washing in PBS twice, cells were fixed in 0.5 mL of 4% formaldehyde for 8 min, transferred onto slides, and allowed to dry in air. The Hoechst 33258 solution was dropped onto each slide, followed by staining for 5 min in dark. The slides were washed with PBS for 1 min and then allowed to dry. The stained cells were observed under a fluorescence microscope. In the miR-19a ASODN + Ara-C group, cells were transfected with miR-19a ASODN and then treated with 0.9 µmol/L Ara-C for 72 h. The cells were observed after Hoechst 33258 staining.
Annexin V/PI double staining and flow cytometry
In experiment 1, cells were collected after 48 and 72 h transfection; in experiment 2, cells were harvested at 72 h. After centrifugation for 5 min, cells washed in PBS twice. These cells were re-suspended in 400 µL of 1× binding buffer and incubated with 5 µL of Annexin V-FITC for 10 min in dark. Cells were treated with 5 µL of PI staining solution. Then, the apoptotic cells were detected by flow cytometry. In the miR-19a ASODN + Ara-C group, cells were transfected with miR-19a ASODN and then treated with 0.9 µmol/L Ara-C for 72 h. The apoptotic cells were detected by flow cytometry.
Western blotting
In experiment 1, cells in each group were collected after 48-h transfection. Total protein was extracted using a protein extraction kit (Sigma, USA) and quantified with BCA method. The protein lysate (100 µL) was mixed with 5× loading buffer (25 µL), followed by denaturation at 100 °C for 5 min. Proteins were separated by 10% SDS-PAGE and electrotransferred onto a nitrocellulose membrane. The membrane was incubated with primary antibodies (E2F1 at 1:200 and Bim at 1:500) at 4 °C overnight, and GADPH served as the internal control. Then, the membrane was incubated with secondary antibodies (mouse anti-rabbit GAPDH antibody at 1:6,000; E2F1 and Bim secondary antibodies at 1:2,000) at room temperature for approximately 1.5 h with a constant shaking (75 rpm). The membrane was then washed in TBST and visualization was done with electrochemiluminescence method (Multi Sciences, China). The membrane was placed in an UV gel image analyzer and photographed.
Statistical analysis
SPSS version 13.0 (SPSS Direct, Chicago) was used for statistical analysis. Data are expressed as mean ± standard deviation (SD). Two-sample t test was employed for the comparisons between two groups. The one way analysis of variance (ANOVA) was used for the comparisons among multiple groups, followed by the S-N-K test between groups. A value of P<0.05 was considered statistically significant.
Results
MiR-19a expression
The miR-19a expression in HL60 cells after 48 and 72 h transfection was calculated (Figure 1). Compared to the blank control group, the blank transfection group and the SODN group, the miR-19a expression in the ASODN group was significantly down-regulated (P<0.05). There was no significant difference in miR-19a expression among the blank control group, the blank transfection group and the SODN group (P>0.05).
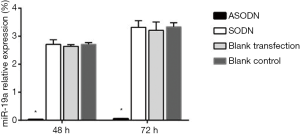
HL60 cell proliferation after transfection with miR-19a ASODN
The cell proliferation was detected by CCK8 assay (Figure 2). After transfection for 48 and 72 h, cell proliferation in the ASODN group was significantly inhibited compared to that in the blank control group, the blank transfection group, and the SODN group (P<0.05). Moreover, the cell proliferation inhibition was the most evident at 48 h. There was no significant difference in the proliferation inhibition rate among the blank control group, the blank transfection group and the SODN group (P>0.05).
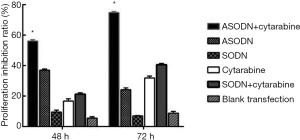
In addition, Ara-C significantly inhibited the proliferation of HL60 cells at 72 h and Ara-C could synergistic effects on the proliferation inhibition of HL60 cells, and the proliferation inhibition rate in the Ara-C + ASODN group was higher than the sum of that in the Ara-C group and the ASODN group (P<0.05).
HL60 cell apoptosis after transfection
After transfection for 48 h, Hoechst staining showed that HL60 cells exhibited apoptotic morphology: decreased cell volume, nuclear condensation and nuclear fragmentation in the ASODN group. HL60 cells in the blank control group, the blank transfection group and the SODN group did not display apoptotic morphology (Figure 3). In experiment 2, Hoechst staining showed more HL60 cells exhibited apoptotic morphology in the ASODN + Ara-C group as compared to the ASODN group, the SODN + Ara-C group and the Ara-C group at 72 h (Figure 4). In addition, Ara-C treatment significantly increased the apoptotic cells, and Ara-C could further increase the apoptotic cells after ASODN transfection.
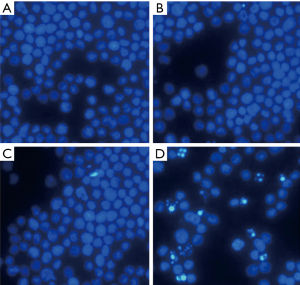
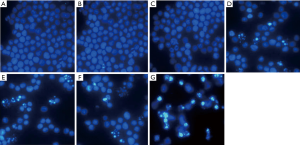
After transfection for 48 and 72 h, the apoptosis rate was detected by flow cytometry after Annexin V/PI double staining. The cell apoptosis rate at 48 h in the ASODN group, the SODN group, the blank transfection group and the blank control group was 18.30%±0.45%, 2.76%±0.11%, 2.20%±0.12% and 1.45%±0.56%, respectively. The cell apoptosis rate at 72 h was 7.80%±0.27% in the miR-19a ASODN group, 3.58%±0.48% in the SODN group, 1.76%±0.21% in the blank control group and 3.93%±0.07% in the blank transfection group. The cell apoptosis rate at 72 h in the miR-19a ASODN group was lower than that at 48 h and also markedly higher than in other groups (P<0.05). However, there was no marked difference in the apoptosis rate among other three groups (P>0.05) (Figure 5). In experiment 2, the cell apoptosis rate in the ASODN + Ara-C group, the SODN + Ara-C group, the Ara-C group and the ASODN group was 37.42%±0.53%, 14.77%±0.44%, 13.40%±0.41%, and 10.83%±0.27%, respectively, and the apoptosis rate in the ASODN + Ara-C group was the highest and higher than the sum of that in both Ara-C group and ASODN group (P<0.05) (Figure 6).
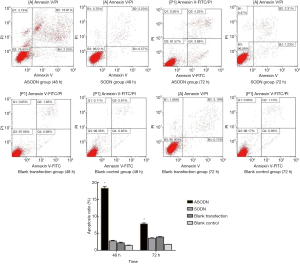
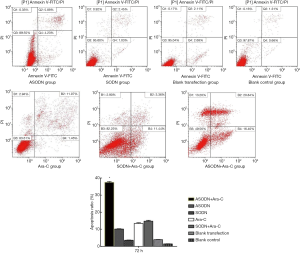
Protein expression of E2F1 and Bim
After transfection for 48 h, the protein expression of E2F1 and Bim was detected by Western blotting (Figure 7). Results showed that the expression of E2F1 and Bim in the ASODN group was significantly higher than in the blank control group, the blank transfection group and the SODN group.
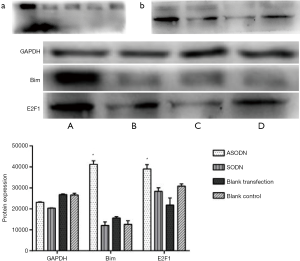
Discussion
MiR-19a plays important roles in the tumorigenesis and tumor progression, and miR-19a over-expression has been found to be closely related to the proliferation, metastasis and prognosis of hematological diseases such as MM, leukemia, and lymphoma (14-17). Feng et al. (9) found that the ASODNs against miR-19a and miR-92a could inhibit the growth, colony formation and invasiveness of MM cells. It has been reported that down-regulation of miR-21 and miR-19b with ASODNs reduced the colony forming ability of leukemia cells in vitro and inhibited their proliferation. Our results showed miR-19 had a high expression in the acute myeloid leukemia cells, and miR-19a ASODN was used to reduce the expression of miR-19a in HL60 cells. Then, the proliferation and apoptosis of HL60 cells were detected.
After transfection of miR-19a ASODN into HL60 cells, the miR-19a expression significantly decreased, and the proliferation of the HL60 cells was markedly suppressed. This suggests that miR-19a ASODN can inhibit the proliferation of the HL60 cells and miR-19a is therefore helpful for the proliferation of the HL60 cells. Hoechst staining showed the HL60 cells after transfection with miR-19a ASODN exhibited apoptotic morphology including decreased cell volume, nuclear condensation, and nuclear fragmentation, and further flow cytometry showed the cell apoptosis rate increased significantly after miR-19a ASODN transfection. This shows that miR-19a ASODN can promote the apoptosis of the HL60 cells.
To investigate molecular mechanism underlying the inhibitory effect of miR-19a ASODN on the HL60 cell proliferation, Western blotting was performed to detect the expression of Bim (6) and E2F1 (18), two target genes of the miR-17-92. E2F1 is a transcription factor closely related to cancer. E2F1 can accelerate cell proliferation through activating the expression of genes in S phase to increase DNA synthesis and also promote apoptosis through the ARF-P53 pathway. In 2009, it was reported that the adenovirus-mediated over-expression of E2F1 induced the apoptosis of human gastric cancer cells and colon cancer cells (19). In 2015, Jin et al. (20) employed lentivirus vector to over-express miRNA-34a in HT29 colon cancer cells and found miRNA-34a over-expression promoted cell apoptosis which was related to E2F1. Our results showed the E2F1 expression increased significantly in the HL60 cells after transfection with miR-19a ASODN. It suggests that the inhibition of HL60 cell proliferation after miR-19a ASODN transfection is related to the inhibition of miR-19a expression and thereafter the increase in E2F1 expression.
Bim is an important regulatory protein. There is evidence showing that Bim mRNA expression significantly reduces in bone marrow cells in case of acute myeloid leukemia (21). Pichiorri et al. (15) found that miR-17-92 clusters in MM cells could inhibit the apoptosis of MM cells and prolong their survival time through directly inhibiting Bim protein expression. In addition, the increased Bim expression may promote cell apoptosis, exerting anti-tumor effect (22,23). In the study of Wang et al. (24), glucocorticoid-induced high expression of Bim was able to induce ALL cell apoptosis in the absence of other apoptotic signals. In the family of miR-17-92, miR-19 is a main component that can facilitate tumor formation, inhibit Bim expression (25) and promote proliferation of tumor cells. In the present study, after down-regulation of miR-19a expression by transfection with ASODN, the Bim protein expression increased in the HL60 cells. This suggests that ASODN-induced down-regulation of miR-19a may increase Bim expression, prompting the apoptosis of the HL60 cells.
MiR-19a expression was inhibited by transfection with miR-19a ASODN in the HeLa cells, which increased the sensitivity of these cells to cisplatin (26). In addition, miR-19a ASODN significantly enhanced the killing activity of doxorubicin on CD133 + HT29 cells in a PTEN/PI3K/AKT/Bad pathway dependent manner (27). In the present study, the synergistic anti-tumor effect of miR-19a ASODN and Ara-C was further investigated in the HL60 cells. CCK8 assay showed that miR-19a ASODN combined with Ara-C significantly inhibited HL60 cell growth in a time dependent manner, and the inhibition rate in the ASODN + Ara-C group was the highest at 72 h and higher than the sum of that in both Ara-C group and ASODN group. Hoechchst staining also indicated more apoptotic cells in the ASODN + Ara-C group than in the Ara-C group and the ASODN group. Flow cytometry showed the cell apoptosis rate in the ASODN + Ara-C group significantly increased and was higher than the sum of that in both Ara-C group and ASODN group. These findings suggest that Ara-C may further elevate the anti-tumor effects of miR-19a ASODN in the HL60 cells.
However, there were some limitations in the present study. In experiment 2, the cell apoptosis was not assessed by Hoechst 33258 staining and flow cytometry at 48 h, and the mechanism by which miR-19a regulates the growth and apoptosis of the HL60 cells was not further investigated. Taken together, our results indicate miR-19a ASODN can inhibit HL60 cell proliferation and increase HL60 cell apoptosis, which are further facilitated with the addition of Ara-C. In addition, miR-19a ASODN-induced inhibition of HL60 cell proliferation may be associated with the up-regulation of target genes of miR-19a (E2F1 and Bim). In the future, we will further study the related mechanisms and genes based on these results.
Acknowledgments
Funding: This work was supported by grants from the Science and Technology Planning Project of Guangdong Province, China (No. 2012B031800474, No. 2013B021800151).
Footnote
Conflicts of Interest: The authors have no conflicts of interest to declare.
References
- Cheng AM, Byrom MW, Shelton J, et al. Antisense inhibition of human miRNAs and indications for an involvement of miRNA in cell growth and apoptosis. Nucleic Acids Res 2005;33:1290-7. [Crossref] [PubMed]
- Chen CZ. MicroRNAs as oncogenes and tumor suppressors. N Engl J Med 2005;353:1768-71. [Crossref] [PubMed]
- Esquela-Kerscher A, Slack FJ. Oncomirs - microRNAs with a role in cancer. Nat Rev Cancer 2006;6:259-69. [Crossref] [PubMed]
- Bousquet M, Harris MH, Zhou B, et al. MicroRNA miR-125b causes leukemia. Proc Natl Acad Sci U S A 2010;107:21558-63. [Crossref] [PubMed]
- Creighton CJ, Reid JG, Gunaratne PH. Expression profiling of microRNAs by deep sequencing. Brief Bioinform 2009;10:490-7. [Crossref] [PubMed]
- Hayashita Y, Osada H, Tatematsu Y, et al. A polycistronic microRNA cluster, miR-17-92, is overexpressed in human lung cancers and enhances cell proliferation. Cancer Res 2005;65:9628-32. [Crossref] [PubMed]
- Wang J, Song S, Xie C, et al. MicroRNA profiling in the left atrium in patients with non-valvular paroxysmal atrial fibrillation. BMC Cardiovasc Disord 2015;15:97. [Crossref] [PubMed]
- Cho WC. OncomiRs: the discovery and progress of microRNAs in cancers. Mol Cancer 2007;6:60. [Crossref] [PubMed]
- Feng MX, Chun-Ming GU, Yin Z, et al. Function of miR-19a and miR-92a determined by seed-targeting inhibition in multiple myeloma cells and their signal pathway analysis. Chin J Pathophysiol 2015;31:1505-9.
- Long C, Tang XY, Zheng FY, et al. The mechanism in of miRNA-19b on the pathogenesis of acute lymphoblastic leukemia. Chin Gerontol J 2016;36:4724-6.
- Murphy BL, Obad S, Bihannic L, et al. Silencing of the miR-17~92 cluster family inhibits medulloblastoma progression. Cancer Res 2013;73:7068-78. [Crossref] [PubMed]
- Zhou M, Cai J, Tang Y, et al. MiR-17-92 cluster is a novel regulatory gene of cardiac ischemic/reperfusion injury. Med Hypotheses 2013;81:108-10. [Crossref] [PubMed]
- Cui XY, Guo YJ, Yao HR. Analysis of microRNA in drug-resistant breast cancer cell line MCF-7/ADR. Nan Fang Yi Ke Da Xue Xue Bao 2008;28:1813-5. [PubMed]
- Mavrakis KJ, Wolfe AL, Oricchio E, et al. Genome-wide RNA-mediated interference screen identifies miR-19 targets in Notch-induced T-cell acute lymphoblastic leukaemia. Nat Cell Biol 2010;12:372-9. [Crossref] [PubMed]
- Pichiorri F, Suh SS, Ladetto M, et al. MicroRNAs regulate critical genes associated with multiple myeloma pathogenesis. Proc Natl Acad Sci U S A 2008;105:12885-90. [Crossref] [PubMed]
- Todoerti K, Barbui V, Pedrini O, et al. Pleiotropic anti-myeloma activity of ITF2357: inhibition of interleukin-6 receptor signaling and repression of miR-19a and miR-19b. Haematologica 2010;95:260-9. [Crossref] [PubMed]
- Zhang X, Yu H, Lou JR, et al. MicroRNA-19 (miR-19) regulates tissue factor expression in breast cancer cells. J Biol Chem 2011;286:1429-35. [Crossref] [PubMed]
- Mundle SD, Saberwal G. Evolving intricacies and implications of E2F1 regulation. Faseb j 2003;17:569-74. [Crossref] [PubMed]
- Wu Z, Yu Q. E2F1-mediated apoptosis as a target of cancer therapy. Curr Mol Pharmacol 2009;2:149-60. [Crossref] [PubMed]
- Jin FL, Cai ZF, Ge YL, et al. miRNA-34a increases the radiotherapy sensitivity of colon cancer by targeting Rb/E2F1 pathway. Chin Med Guide 2015;12:13-6.
- Long SFA, Chen SJB. Relationship between Expressions of Proapototic Protein Bim and Ferritin in Leukemia. J Practic Clini Med 2008;9:7-9.
- Puthalakath H, Strasser A. Keeping killers on a tight leash: transcriptional and post-translational control of the pro-apoptotic activity of BH3-only proteins. Cell Death Differ 2002;9:505-12. [Crossref] [PubMed]
- Yip KW, Li A, Li JH, et al. Potential utility of BimS as a novel apoptotic therapeutic molecule. Mol Ther 2004;10:533-44. [Crossref] [PubMed]
- Wang Z, Malone MH, He H, et al. Microarray analysis uncovers the induction of the proapoptotic BH3-only protein Bim in multiple models of glucocorticoid-induced apoptosis. J Biol Chem 2003;278:23861-7. [Crossref] [PubMed]
- Wang F, Li T, Zhang B, et al. MicroRNA-19a/b regulates multidrug resistance in human gastric cancer cells by targeting PTEN. Biochem Biophys Res Commun 2013;434:688-94. [Crossref] [PubMed]
- Cui L, Du J, Geng H, et al. Targeted suppression of miR- 19a enhanced chemosensitivity of cervical cancer HeLa cells to cisplatin. J Modern Oncol 2015;23:605-7.
- Pan HQ, Shen F, Cui JH, et al. Study the Relationship Between MiR-19 Antisense Oligonucleotides and the Sensitivity of CD133+HT29 Cell Subsets to Doxorubicin. Chin J Mod Appl Pharm 2017;34:215-20.