Nutrition and exercise in Pompe disease
Introduction
Pompe disease (PD) is due to acid alpha-glucosidase (GAA) deficiency, which causes accumulation of lysosomal glycogen and autophagic buildup, leading to progressive skeletal muscle (SM) deterioration, functional impairment, and disability (1). Lifestyle and dietary factors, such as physical inactivity and inadequate food intake, may also accelerate disease progression in patients with muscle disease. For example, a low energy, protein and/or micronutrient intake may limit the ability to withstand infections and other metabolic stressors (2). Another common complication of muscle weakness is avoidance of physical activity (PA), which lowers energy expenditure and predisposes for weight-gain. Consequently, in order to maintain bodyweight, patients with muscle disease often consume too few calories, including key nutrients for maintaining SM mass, such as protein (3). The invariant oro-pharyngeal dysphagia that accompanies infantile-onset Pompe disease (IOPD) (4), and occasionally the late-onset form (LOPD) (5,6), further complicates the ability to maintain safe and adequate oral intake. From a weight-management perspective, it is therefore often not possible to reduce energy intake further, which predisposes to obesity, as previously described in some Pompe patients (7). A reduction in muscle mass and increase in body fat are interactively deleterious to health, as seen in older adults with sarcopenic obesity (8). The role of low SM mass (cachexia) combined with excessive body fat is likely underappreciated in patients with muscle disease. Therefore, ensuring an adequate energy intake that provides sufficient protein to maintain SM mass and function, whilst preventing body fat gain, is a delicate balancing act in PD patients, and more research is warranted in this area.
One strategy to enhance SM mass, strength and function is resistance exercise training (RET) and this has been used successfully in a wide variety of muscle diseases (9-13). Endurance exercise training (AET) is classically associated with improvements in aerobic capacity, and several studies have found improvements in fitness and/or functional capacity in patients with primary muscle disorders (14-17) and metabolic myopathies (18-20). More recently, high intensity interval training (HIIT), by itself or when combined with regular AET and RET, has been shown to improve fitness in fascio-scapulo-humeral muscular dystrophy (MD) patients (21,22). Combined endurance and resistance exercise programs have also been shown to improve muscle strength in Pompe patients (10,23-25). A common issue with both IOPD and LOPD is progressive restrictive ventilatory failure (1,26). Several forms of respiratory muscle exercise training have been successfully described in MD (27-29). More recently, several studies have shown that respiratory exercise training is helpful in PD (30-32).
The purpose of this review is to describe the major clinical challenges associated with providing nutritional and exercise guidelines to children and adults with PD. The nutritional section will cover our current knowledge on body composition and habitual nutrient intake in PD patients, and delineate specific supplementation strategies to mitigate obesity and SM deterioration in this population. The exercise section will provide a general overview of the benefits of mixed exercise training (AET and RET), how it mitigates PD progression, and why it should be used as an adjunct to enzyme replacement therapy (ERT) in LOPD. We will then discuss the rationale for combining both exercise and dietary intervention to mitigate PD progression.
Body composition
The physical manifestation of low energy and protein intake is cachexia, and this is reflected in low muscle mass, often estimated in large population studies using body mass index (BMI) [wt (kg)/ht (m)2]. A BMI of <18.5 is considered underweight and is suggestive of cachexia whilst values ≥30 suggest obesity. Given that it is the actual amount of muscle and fat that are most important in determining nutritional adequacy and individual recommendations, a more accurate determination of these metrics is required in research and clinical care. Dual energy absorptiometry (DEXA) has become a gold standard tool for the determination of body composition (body fat and lean mass) and bone density in neuromuscular disease and aging research (33).
DEXA scanning was used to evaluate body composition in nine LOPD patients and found that whilst the BMI indicated obesity in only one patient (38.7), eight of the nine were obese based upon body fat (>30% body fat) and three patients were over 50% body fat (7). Further indicating the inadequacy of BMI in the evaluation of individual patients was their finding that the patient with the lowest BMI (19.1, close to underweight) had a body fat percentage of 38.7% (e.g., obesity) (7). Another study found that the average percent body fat in five LOPD patients was in the obese range at ~45% (23). Consequently, it appears that obesity is a significant issue in LOPD patients and is concerning for it will increase the risk of obesity-associated morbidities (34), especially when combined with low PA (35-37). Furthermore, the extra weight is a major issue in a patient with SM weakness and will further impair activities of daily living (ADL). Unfortunately, the results of a 20-week exercise intervention study showed that the percent body fat did not change in spite of significant functional improvements (23). The latter findings are similar to results in able-bodied middle-aged men and women (38,39), suggesting that exercise must be combined with significant dietary restrictions in order to lower the percent body fat once a patient is already obese.
DEXA scanning has also found a low bone mineral density (BMD) in a high proportion of Pompe patients (40-42), and that low BMD correlated with muscle weakness (41). The latter effect is likely related to the known stimulatory effect of muscle contraction on osteoblasts (synthesis) and the failure of osteoblast activation with reduced mechanical stimuli (43,44). As expected, the low bone mass in Pompe patients further declines over time (40). Muscle weakness and low BMD are a dangerous combination for they lead to an increased fall risk and subsequent fracture risk (45,46). A femur or hip fracture in a patient who is very weak can be the seminal event resulting in a permanent loss of ambulation and can even lead to higher mortality risk (47). To what extent the low BMD seen in some Pompe patients is due to hypodynamia, low vitamin D status, and/or low protein intake is unclear, but all are likely contributors to some extent in specific patients and all are modifiable.
The above data suggests that many Pompe patients have low lean body mass (muscle) and high body fat, suggesting sarcopenic obesity. Furthermore, BMI is an inadequate tool for the individual assessment of Pompe patients. The data also suggests that DEXA scanning of all patients will help to assess lean muscle mass, body fat and bone density and allow for the ability to follow and quantify changes over time in response to various interventions. Furthermore, it will also allow for a more accurate assessment of body composition to identify issues at an early stage where intervention may be preventative (i.e., lower energy intake if obese, calcium/vitamin D if osteopenic, and bisphosphonates if osteoporotic). Finally, the DEXA scan analysis can be used as another marker for the efficacy of an intervention such as ERT or exercise.
Protein turnover and dietary concerns for Pompe patients
At the cellular level, the net protein balance of a tissue or whole body is a function of protein synthesis—protein breakdown/oxidation. Exercise and nutrition are important independent and interactive determinants of protein balance (48-50). For example, it has been well documented that acute bouts of resistance exercise lead to a more positive net muscle protein synthesis in healthy younger and older adults (48,51,52). It has also been shown that the provision of high-quality proteins [e.g., milk-based (whey and casein) and eggs (53)] synergistically enhance the protein stimulatory effects of resistance exercise. Importantly, these stimulatory effects are more potent for milk- vs. plant-based proteins (e.g., soy) (54,55). The superiority of milk and egg proteins over plant-based proteins is due to the higher biological value of the former, which contain a more balanced complement of the essential amino acids. Of the essential amino acids, leucine (a branched chain) appears to be the most potent in stimulating protein synthesis through mTOR activation (56).
There has only been one study that has looked at protein turnover is LOPD patients (N=5) and they reported a higher leucine rate of appearance (crude measure of protein breakdown) and a mild increase in leucine oxidation with a higher alanine rate of appearance all when expressed per lean body mass (57). Metabolic studies have also shown that altered nutrient sensing contributes to a greater protein breakdown in PD (58). Collectively, the above data suggests a net loss of protein through protein breakdown/oxidation that eventually leads to the canonical observation of lower muscle mass in patients. Interestingly, Raben’s group recently demonstrated that GAA KO mice exhibit an impairment in the mTOR signalling pathway with sequestration of mTORC1 to the lysosome due to a lysosomal acidification defect and constitutive excess of TSC2, which ultimately inhibits mTORC1 activity (despite being localized to the lysosomal membrane) and impairs protein synthesis (59-61). They also demonstrated that the addition of L-arginine to GAA-/- animals leads to an increase in mTORC1 activation by TSC2 inhibition, which may remove autophagic buildup by inhibiting transcription factor EB (TFEB) and autophagosome formation (59). From a therapeutic perspective, L-leucine supplementation also shows promise for activating mTORC1 and augmenting protein synthesis in PD patients. For example, it was recently demonstrated that the addition of cell-permeable leucine to cell culture from an IOPD patient and L-leucine to the GAA-/- murine PD model, led to substantial mTORC1 activation and improvements in running, muscle mass and spontaneous activity (62). As such, it is plausible that leucine-induced mTORC1 activation will alleviate the buildup of new autophagosomes by inhibiting TFEB, albeit by a different mechanism than arginine (in this case TSC2 independent) (62). However, more research is needed to discern which amino acid is the most safe and effective adjunctive therapy to ERT in PD. To date, the only amino acid that has been evaluated in LOPD patients is the non-essential amino acid alanine that was added to a high protein diet plus exercise by Slonim et al. (63,64); however, given the open design and co-intervention it was not possible to determine whether alanine was independently and/or interactively beneficial.
Most of the nutritional recommendations for Pompe patients have emphasized the importance of a high protein diet (63,65-67). There is some evidence that a high protein diet can alter the impairments in protein turnover seen in PD (57). From the functional perspective, one case report (68), and a case series (64), reported improvements in body composition and/or muscle function in Pompe patients after a high protein diet intervention. In contrast, a small study (N=5) found improvements in protein turnover but no clinically relevant benefits from a high protein diet (57). Two small scale studies have found improvements in respiratory function in Pompe patients with a high protein diet (66,69). A longer-term (2–10 y) study in 34 patients with PD found that those who complied with a high protein diet (25–30% energy from PRO +1.5 g alanine 4 times a day) had a slower rate of physical decline over time (63); however, the latter study had no control group, and the intervention was both a nutritional and exercise intervention; consequently, it was unclear what proportion of the effect was attributed to each of the interventions or other factors.
To date, there have been no systematic evaluations of plasma vitamin and mineral levels in patients with PD; however, our data in MD patients (3), would predict that a substantial number of Pompe patients will also have a hypoenergetic dietary intake and a low intake of many micronutrients (vitamins and minerals). For example, our group found that 12/12 of our LOPD patients had plasma vitamin D levels that were insufficient (<75 nmol/L) and 4/12 were below the deficiency (<30 nmol/L) range (Tarnopolsky et al., 2019, unpublished observations). Further supporting the importance of measuring and treating low vitamin D levels comes from the observation that a substantial proportion of patients have low BMD (41,70). From a practical perspective, it would be reasonable to consider suggesting a balanced multi-vitamin that meets the minimal daily requirement for the given country and provide 2,000 IU/d vitamin D supplementation to all LOPD and 1,000 IU/d to all IOPD patients outside of equatorial regions, unless contraindications would preclude this.
There have been very few studies looking at specific supplements in LOPD patients in contrast to the many evaluating creatine monohydrate in MD (71-74). A Cochrane review of the literature found that creatine monohydrate supplementation was associated with an 8.5% increase in muscle strength in MD (75); however, myotonic MD type 1 patients appeared to show no effect (73,74). Given the similarities between MD and PD, it is likely that at least some of the benefits reported in MD would be seen in PD; however, this remains a study that requires further evaluation. Another intervention that has been informally discussed but not formally studied in the PD population is the ketogenic diet (http://www.pompewarriorfoundation.com/research/). A ketogenic diet is usually >60% fat with very low carbohydrate (<50 g/d). The possible theories suggested for improving PD are that ketones (B-OH-butyrate and acetoacetate) can enhance muscle mass in MD (76), lower inflammation (77), activate chaperone-mediated autophagy (78), and that a very low carbohydrate intake is a form of substrate reduction therapy (SRT). The potential benefit of SRT was shown in the murine Pompe model with genetic knock-down of glycogen synthase leading to improved function (79). Practical issues with the ketogenic diet are issues of long-term compliance and care to ensure adequate micronutrient intake. Another concern is the potential to increase neutral lipids in the liver and lead to non-alcoholic fatty liver disease (NAFLD) and hepatic insulin resistance (80,81). Clearly, with the anecdotal evidence of “benefit” it is important that this concept be first evaluated in the murine model and if successful, in a randomized human clinical trial.
Exercise, health-span, and longevity
While the effects of habitual PA on ‘health-span’, mortality risks, and longevity are unknown for many rare diseases, findings in the general population largely extend to other human conditions. Cumulative evidence amassed over the last 50 years unequivocally show that sedentary living predisposes to chronic disease, disability, and premature death (82,83). Conversely, regular exercise, such as RET and AET, provides multi-systemic benefits that protect against morbidity, all-cause mortality, and extend average life expectancy (84-87). The reduction in all-cause mortality is primarily attributed to enhanced maximal aerobic capacity (VO2max), but also to protection against obesity, type 2 diabetes, cancer, osteoporosis, hip fracture, depression, anxiety, and cognitive decline (88-93). Although vigorous exercise is particularly effective in reducing mortality risks (84,94), low-to-moderate intensity PA also confers substantial pro-longevity benefits and is more suitable for patients (95).
Exercise adaptations
The canonical adaptations to endurance and strength training are listed in Table 1, and these generally apply to special populations as well, including LOPD patients. As discussed, VO2max is a strong predictor of all-cause mortality and may be improved by AET, which augments cardiac output (e.g., stroke volume) and systemic oxygen extraction (e.g., vasodilatory function, capillary density, and mitochondrial volume) (96,97). Other well-known adaptations to AET include a reduction in fat mass, improved insulin sensitivity/glucose regulation, and an enhanced blood lipid profile. Similarly, strength training reduces mortality risk, but by augmenting indices closely related to SM and bone mass, including muscle function (strength, endurance, and power), ADL, fall risk and institutionalization (98,99). For these reasons, AET is considered the cornerstone of cardiometabolic disease prevention, while RET is mainly used as a tool to combat age- and disease-related muscle loss (e.g., sarcopenia and cachexia, respectively). For increased efficacy, RET may be combined with oral supplementation of high-quality protein (e.g., whey and casein) (100). From a clinical perspective, it is important to recognize that there is significant overlap between the two exercise modes. For example, weight-bearing AET (e.g., walking, running, and cycling) provides a small but significant anabolic stimulus to muscles and bones (101), and RET may also improve cardiovascular function, albeit to a lesser extent than endurance training (102,103).
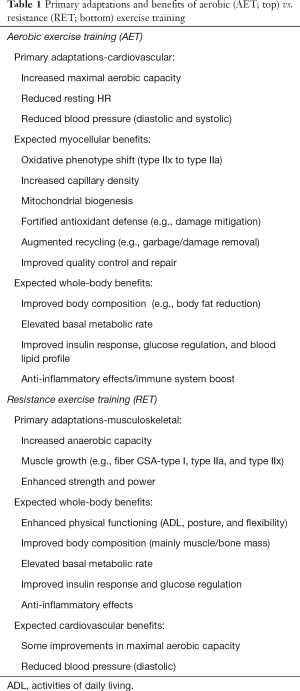
Full table
Exercise hormesis—the molecular basis
Concomitant accumulation of danger-associated molecular patterns (DAMPs), including oxidative damage, protein aggregates, and lipofuscin, is a common feature of biological aging, neurodegenerative disease, and lysosomal storage disorders (104-107). Intervention strategies that rejuvenate the organelles/systems that regulate ATP and ROS production (mitochondria), waste recycling (autophagosome, lysosome, and 26 proteasome), and protein quality control (unfolded protein response, UPRER and UPRMT) may mitigate garbage buildup (e.g., ‘autophagic blockage’) and thereby preserve critical organ function (108). Exercise is an acute stress signal (ROS, Ca2+, pH, and hypoxia) that activates evolutionary conserved cell danger response programs (CDR and DDR, respectively), lowers the energy state of the cell (NAD+/NADH and AMP/ATP ratios), and promotes the release of neurotransmitters, hormones, and circulatory factors (e.g., exerkines), which coordinately stimulate mitochondrial biogenesis (CaMK II, PGC-1α, SIRT1, and AMPK), antioxidant defense (Nrf2-Keap1, NFκB, and MAPK), waste processing (ULK1-Beclin1, TFEB, and FOXO3a), and the immune response (NLRP3, IL-1β, IL-6, IL-10, IL-1ra, sTNF-R, and IL-18) (107-118). Repeated exposure to acute, non-lethal stress, such as AET, results in cellular adaptation into a more stress-resistant level, signified by rejuvenated mitochondria, fortified antioxidant defense, reduced oxidative damage/garbage buildup, and improved immunity (e.g., concept of exercise hormesis) (107,108,119,120). Collectively, there is a strong molecular basis for using exercise therapy in PD management, but a combined approach consisting of mixed AET/RET and intermittent supplementation of specific micronutrients, high-quality proteins, and amino acids (see text for details), may be necessary to maintain critical organ reserves, functional capacity, and independence in LOPD patients (Figure 1).
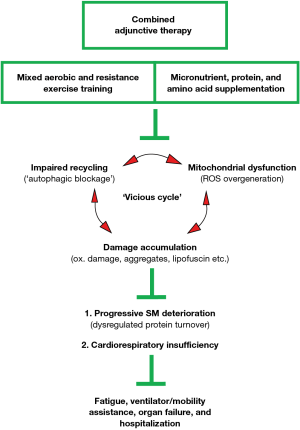
Exercise guidelines
Numerous position stands, authoritative reviews, and meta-analyses conclude that AET and RET should be prescribed together in concurrent/mixed training programs to maximize health benefits (91-93). For example, a traditional, mixed training protocol consisting of 16–20 weeks of AET (3–5 d/week at 50–75% VO2max) and RET (2–3 d/wk at 65–85% 1-RM) is sufficient to bestow the vast majority of the proven benefits of AET and RET in untrained individuals (Table 1). Considering the low risk-benefit ratio, LOPD patients are encouraged to engage in low-to-moderate intensity exercise under supervision on most days of the week (95,121-123). Clinicians and physical therapists should adhere to the standard exercise principles for untrained individuals [e.g., FITT formula-frequency, intensity, time, and type (124)], keeping in mind that excessively strenuous exercise, particularly with an eccentric component, may exacerbate muscle deterioration (123). Gentle strengthening exercises and stretching with a focus on functionality are therefore recommended, while overwork weakness, excessive fatigue, and muscle pain should be avoided (121,123). Notably, the safety and efficacy of mixed RET, AET, and core stability training were recently demonstrated in LOPD patients (discussed in the next section) (10,23,25), but in view of the substantial therapeutic benefits of AET alone, it may be the preferred single modality, with exercise intensities as high as 60–70% VO2max (e.g., ~70–80% HRmax) well-tolerated (122).
Exercise therapy in LOPD patients
In general, exercise trials are lacking in LOPD and in the majority of those that have been completed, the number of patients have been too low to draw definite conclusions about safety and efficacy. Perhaps the first suggestion of the potential benefit of exercise in PD came from the “nutrition and exercise therapy (NET)” reported by Dr. Slonim’s group (64). This case series reported on 34 Pompe patients who followed a nutrition (low carbohydrate + high protein + alanine 1.5 g four times a day) and exercise (45–50 min of aerobic treadmill walking at ~65% VO2peak + 10–15 min of arm ergometry) program for two–ten years (63). They found that the Walton scores (a measure of function) plateaued or improved in the 26 compliant patients whilst, declining in the eight non-compliant patients (63). It is difficult to know what proportion of the benefits could be attributed to the exercise and what could be attributed to the nutritional intervention in the latter study given that there was a co-intervention.
Another study indirectly evaluated exercise interventions using 6 minute walk distance in 44 Pompe patients before and after 12 months of ERT where they completed a sub-group analysis in five patients who did cycle ergometry every 2 weeks during the ERT infusion, with three subjects also doing “regular physiotherapy” (125). Each of the five patients showed improvements in the 6-MWT and ranked # 3,4,5,6,12 of the 21 patients (6 of the non-exercisers showed no improvement or a loss in performance) who completed the 6-MWT before and after the 12 month ERT trial (125). Again, there were confounders for only 3 of 5 participants did regular physiotherapy exercise (endurance-based), and with the small sample size it was impossible to determine of the benefit was from enhanced ERT uptake and/or the regular exercise.
In addition to the obvious benefits of increased aerobic capacity/fitness to an adult with PD (35,126), endurance exercise is likely to be of benefit to Pompe patients for other specific reasons including; a reduction in the risk of type 2 diabetes (127), and a reduction in body fat [obesity is more common in Pompe patients (7)]. Fatigue is also a common feature in adults with PD with severe fatigue reported in ~66% of cases (128). Even if only a portion of the fatigue in a Pompe patient has a central generator, a potential benefit from AET is likely to be lower peripheral and central fatigue. Finally, it is possible that AET and/or performing endurance exercise during ERT may enhance ERT uptake by the working muscle [greater capillarization, increased blood flow, increased number of mannose-6-phosphate receptors (125)]. Unfortunately, the potential for a synergistic effect of exercising whilst receiving ERT did not appear to improve function in a small study with five late onset Pompe patients (24); however, the initial evaluation of 6-MWT and strength in this study occurred after several months of both ERT and exercise and this would make it difficult to determine the effect of exercise during ERT for the largest effects (ERT + exercise) had already occurred (24).
A protean manifestation of PD is muscle weakness (1). Several studies have found that a RET program increased muscle strength in patients with MD (9,12). It is conceptually attractive to hypothesize that resistance exercise would also increase strength and ultimately function in patients with PD, yet only a few studies have been completed to date (10,23,25). Terzis and colleagues used a progressive resistance exercise program (following an aerobic cycling warm-up) for 20 weeks in 5 Pompe patients who had received ERT for one year (23). They reported improvements in muscle strength in four exercise tasks ranging from + 18.7–50.8% (3 of 4 statistically significant), and a significant increase in arm FFM (23). Importantly, they also found an increase in 6-minute walk test distance (6-MWT) with a very strong statistical relationship between hip extension strength and the 6-MWT (23). Although there was an endurance exercise component to the training, it is likely that most of the strength outcomes were a direct result of the resistance training component of the program (23). In a separate study, van der Ploeg’s group examined the effects of mixed AET (60% VO2max, 2×15 min), RET (70% 4-RM, 3 sets ×15 reps, 6 exercises), and core stability (4×30 sec, 4 exercises) training on aerobic capacity, body composition, muscle strength, and function (10,25). Significant improvements in VO2max, 6-MWT, hip flexor strength, shoulder abductor strength, core stability, 4-step stair-climbing, and time-to-stand from supine position were reported, while body composition and pulmonary function (FVC) did not change. The latter finding stresses the importance of a dedicated program to improve FVC in LOPD. Another important finding of this study was that moderate-intensity mixed exercise is safe and well-tolerated since 23 out of 25 patients completed the training program. In the future, it will be important to determine the independent and interactive benefits of RET and AET in a larger cohort of patients and in children.
Exercise therapy in Pompe mice
To further evaluate the biochemical and molecular mechanisms behind a potential benefit of exercise in Pompe patients, we evaluated several outcome metrics in the GAA-/- murine model of PD (105,129). Wild-type controls and GAA-/- mice were randomly allocated to a progressive 14-week AET program (45 min, 5 d/wk) or a sedentary lifestyle with 50% also receiving bi-weekly ERT (40 mg/kg) nested within the exercise and sedentary allocations (129). To address the issue whether acute increases in blood flow enhance ERT uptake, we also added another cohort of GAA-/- mice that exercised for 30 min (warm-up), followed by an infusion of ERT (40 mg/kg) and a 60 min post-injection run at 15 m/min. The uptake of ERT did not appear to be enhanced in either muscle or heart with acute endurance exercise (as reflected by GAA enzyme activity). In addition, fourteen weeks of ERT was associated with an approximate doubling of GAA activity in both heart and quadriceps muscle in the GAA-/- mice with no further enhancement from AET. Although ERT alone enhanced grip strength in treated animals, it did not improve aerobic fitness (maximal running test) or motor function, dynamic balance, and dexterity (rotarod test) to the same extent as the mice receiving polytherapy (e.g., ERT and AET). Finally, glycogen clearance was nearly 100% in heart and soleus (slow-twitch muscle) vs. ~40–80% complete in fast-twitch muscles (quadriceps, plantaris, and diaphragm) in both ERT-treated groups (with and without EX), but was not independently affected by exercise alone or synergistically enhanced in mice receiving polytherapy (ERT + EX) (129).
The above data strongly suggest that the clinical benefits of AET reported in LOPD patients (24), are due to mechanism(s) independent of enhanced removal of glycogen. Our follow-up analyses indicated that AET rejuvenated the mitochondrial-lysosomal axis, and that the fast-twitch muscles of the polytherapy-treated mice (ERT + EX) exhibited less oxidative damage and autophagic buildup compared to unexercised controls (105). Considering that contractile activity stimulates antioxidant (Nrf2-Keap1, NFκB, and MAPK) and recycling (TFEB, FOXO3, and ULK1-Beclin1) pathways, AET may have mitigated ‘autophagic blockage’ in the polytherapy group. Although the clearance of autophagic debris by AET has yet to be shown in humans, and it is difficult to extrapolate our findings, it is interesting that the initial clinical sign reported by most LOPD patients is impaired exercise capacity (1). Since a lack of endurance and balance/coordination issues lead to functional impairment and increased fall-risk, respectively, the functional metrics reported in our mouse study may have clinical relevance.
Respiratory training in LOPD patients
Given that respiratory weakness is a major cause of morbidity and mortality in both IOPD and LOPD patients (1,6,26), strategies that could improve respiratory muscle strength/function could have very significant long-term benefits. In fact, respiratory function was the co-primary outcome in the seminal study evaluating the effect of rGAA ERT in LOPD (130). The first study to evaluate respiratory muscle strength training used a device that provided 60% of the maximal inspiratory and expiratory strength with 25 repetitions twice a day six days a week (31). The duration in the two patients varied from 12 to 32 weeks, yet the increases were ~73% for inspiratory strength and 31–48% for expiratory strength (31). This same group used a 12-wk respiratory muscle strength protocol in eight LOPD patients followed by 12 weeks of detraining (30). They reported a 19.6% increase in maximal inspiratory pressure and a 16.1% increase in maximal expiratory pressure in the eight patients (30). Importantly, these gains were maintained during the 12 weeks of detraining (30). A similar increase in maximal inspiratory pressure (strength) of 15.7% at 6 weeks and 26.4% at 52 weeks was reported with progressive inspiratory muscle strength training in 11 LOPD patients (131). There is only one report of respiratory muscle training in IOPD with five patients on full time ventilation requirements and four on part-time requirements (32). They trained for 90 days using 3–4 sets of 6–10 inspiratory efforts with a modified threshold inspiratory training device, and then received adenovirus GAA gene therapy directly to the diaphragm and continued training for ~ one year (32). The exercise training alone (90 day before gene therapy) leads to a significant increase in peak inspiratory flow and a trend for an increase in tidal volume (32). Collectively, the above data show that inspiratory and combined inspiratory/expiratory muscle strength training improves important metrics of respiratory function ~15–45% in LOPD patients, and there appears to be some improvement even in ventilator dependent IOPD patients. Importantly, none of the studies showed any evidence of serious side effects attributable to the therapy such as a pneumothorax.
Conclusions
Many patients with PD show evidence of sarcopenic obesity with a low muscle mass and high body fat percentage. Furthermore, a high proportion show low BMD that can be a precursor to osteoporosis and fracture risk. The best way to determine the body composition in an individual patient is a DEXA scan. The cause of the aforementioned body composition alterations is likely sub-optimal protein intake combined with excessive energy intake that is compounded by muscle weakness and lower amounts of PA. Patients should consume protein intake levels (~1.2–1.4 g/kg/d) above those recommended for the general population (0.8–1.0 g/kg/d) but it is unclear if very high levels (>1.6 g/kg/d) would confer benefit or may lead to excessive energy intake. To optimize the muscle protein synthesis effects of protein it is suggested that proteins of higher biological value be consumed (milk, whey, eggs, lean meat and fish). It is likely that the low energy intakes required by relatively inactive patients would also lead to sub-optimal vitamin intake, as we have shown in MD patients (3), with vitamin D being most likely to be deficient in non-equatorial regions. A balanced multi-vitamin supplement would likely be a reasonable suggestion for most patients given the likely low total energy intake.
At this point, it is not clear whether specific amino acid supplementation would confer functional benefits to Pompe patient. It is theoretically attractive to consider an interactive exercise-nutrition program, for example, consumption of high quality proteins enriched with leucine in conjunction with RET for augmenting mTOR activity and protein synthesis or L-arginine supplementation combined with AET (or intermittent fasting) for TFEB activation and increased autophagic flux, although such strategies remain to be elucidated. The data in MD patients would suggest that creatine monohydrate would improve muscle strength in Pompe patients (75); however, this should be evaluated specifically in Pompe patients in the future. Similarly, although there are theoretical reasons to speculate that a ketogenic diet could confer benefits in PD, this has not yet been studied in murine models or patients.
Exercise confers many health benefits to able-bodied men and women and these will very likely extend to those with neuromuscular disorders including PD. Animal studies suggest that the benefits come from an enhanced autophagy and mitochondrial biogenesis and not an increase in the delivery or uptake of ERT. Similarly, the hypothesis that exercise during ERT will improve uptake has not been supported by animal studies, nor proven in human trials. The combination of both endurance and RET programs for Pompe patients has been shown to improve strength and function substantially and this combined approach also fits with the theoretical optimal program for patients and older adults. Given the involvement of the respiratory system in LOPD and IOPD, it is encouraging that specific respiratory exercise training improves respiratory capacity. The effects of exercise reported to date are actually above the current ERT benefits (130). For example, 18 months of ERT was associated with a 28.1 m improvement in the 6-MWT (130), whilst 5 months of ERT combined with endurance and resistance exercise led to a 44.4 m improvement in the 6-MWT (23). Furthermore 18 months of ERT was associated with a 3.4% improvement in respiratory capacity (130), with several months of respiratory exercise being associated with a ~18–50% increase in respiratory capacity (30,31). Overall, exercise training appears to be a safe, effective and inexpensive intervention to improve functional metrics of importance to the health of Pompe patients.
Acknowledgments
Dr. Tarnopolsky has grant support from the Canadian Institute for Health Research (CIHR) to investigate therapies for muscle disorders, including Pompe disease. Dan Wright and family, Warren Lammert and family and Giant Tiger Stores have supported Dr. Tarnopolsky’s research into therapies for glycogen storage disease and mitochondrial disease.
Footnote
Conflicts of Interest: Drs. Tarnopolsky and Nilsson have shares in Exerkine Corporation and the company has filed a patent on the use of a ketogenic compound for the treatment of Pompe disease. Dr. Tarnopolsky has received speaker honoraria from Sanofi-Genzyme.
References
- Hagemans ML, Winkel LP, Van Doorn PA, et al. Clinical manifestation and natural course of late-onset Pompe's disease in 54 Dutch patients. Brain 2005;128:671-7. [Crossref] [PubMed]
- Wintergerst ES, Maggini S, Hornig DH. Contribution of selected vitamins and trace elements to immune function. Ann Nutr Metab 2007;51:301-23. [Crossref] [PubMed]
- Motlagh B, MacDonald JR, Tarnopolsky MA. Nutritional inadequacy in adults with muscular dystrophy. Muscle Nerve 2005;31:713-8. [Crossref] [PubMed]
- Jones HN, Muller CW, Lin M, et al. Oropharyngeal dysphagia in infants and children with infantile Pompe disease. Dysphagia 2010;25:277-83. [Crossref] [PubMed]
- Hobson-Webb LD, Jones HN, Kishnani PS. Oropharyngeal dysphagia may occur in late-onset Pompe disease, implicating bulbar muscle involvement. Neuromuscul Disord 2013;23:319-23. [Crossref] [PubMed]
- van der Beek NA, de Vries JM, Hagemans ML, et al. Clinical features and predictors for disease natural progression in adults with Pompe disease: a nationwide prospective observational study. Orphanet J Rare Dis 2012;7:88. [Crossref] [PubMed]
- Papadimas GK, Terzis G, Methenitis S, et al. Body composition analysis in late-onset Pompe disease. Mol Genet Metab 2011;102:41-3. [Crossref] [PubMed]
- Batsis JA, Villareal DT. Sarcopenic obesity in older adults: aetiology, epidemiology and treatment strategies. Nat Rev Endocrinol 2018;14:513-37. [Crossref] [PubMed]
- Milner-Brown HS, Miller RG. Muscle strengthening through high-resistance weight training in patients with neuromuscular disorders. Arch Phys Med Rehabil 1988;69:14-9. [PubMed]
- Favejee MM, van den Berg LE, Kruijshaar ME, et al. Exercise training in adults with Pompe disease: the effects on pain, fatigue, and functioning. Arch Phys Med Rehabil 2015;96:817-22. [Crossref] [PubMed]
- Siciliano G, Simoncini C, Giannotti S, et al. Muscle exercise in limb girdle muscular dystrophies: pitfall and advantages. Acta Myol 2015;34:3-8. [PubMed]
- Sveen ML, Andersen SP, Ingelsrud LH, et al. Resistance training in patients with limb-girdle and becker muscular dystrophies. Muscle Nerve 2013;47:163-9. [Crossref] [PubMed]
- Murphy JL, Blakely EL, Schaefer AM, et al. Resistance training in patients with single, large-scale deletions of mitochondrial DNA. Brain 2008;131:2832-40. [Crossref] [PubMed]
- Andersen G, Prahm KP, Dahlqvist JR, et al. Aerobic training and postexercise protein in facioscapulohumeral muscular dystrophy: RCT study. Neurology 2015;85:396-403. [Crossref] [PubMed]
- Olsen DB, Orngreen MC, Vissing J. Aerobic training improves exercise performance in facioscapulohumeral muscular dystrophy. Neurology 2005;64:1064-6. [Crossref] [PubMed]
- Sveen ML, Jeppesen TD, Hauerslev S, et al. Endurance training: an effective and safe treatment for patients with LGMD2I. Neurology 2007;68:59-61. [Crossref] [PubMed]
- Vissing CR, Preisler N, Husu E, et al. Aerobic training in patients with anoctamin 5 myopathy and hyperckemia. Muscle Nerve 2014;50:119-23. [Crossref] [PubMed]
- Haller RG, Wyrick P, Taivassalo T, et al. Aerobic conditioning: an effective therapy in McArdle's disease. Ann Neurol 2006;59:922-8. [Crossref] [PubMed]
- Taivassalo T, Gardner JL, Taylor RW, et al. Endurance training and detraining in mitochondrial myopathies due to single large-scale mtDNA deletions. Brain 2006;129:3391-401. [Crossref] [PubMed]
- Jeppesen TD, Schwartz M, Olsen DB, et al. Aerobic training is safe and improves exercise capacity in patients with mitochondrial myopathy. Brain 2006;129:3402-12. [Crossref] [PubMed]
- Andersen G, Heje K, Buch AE, et al. High-intensity interval training in facioscapulohumeral muscular dystrophy type 1: a randomized clinical trial. J Neurol 2017;264:1099-106. [Crossref] [PubMed]
- Bankolé LC, Millet GY, Temesi J, et al. Safety and efficacy of a 6-month home-based exercise program in patients with facioscapulohumeral muscular dystrophy: A randomized controlled trial. Medicine (Baltimore) 2016;95:e4497. [Crossref] [PubMed]
- Terzis G, Dimopoulos F, Papadimas GK, et al. Effect of aerobic and resistance exercise training on late-onset Pompe disease patients receiving enzyme replacement therapy. Mol Genet Metab 2011;104:279-83. [Crossref] [PubMed]
- Terzis G, Krase A, Papadimas G, et al. Effects of exercise training during infusion on late-onset Pompe disease patients receiving enzyme replacement therapy. Mol Genet Metab 2012;107:669-73. [Crossref] [PubMed]
- van den Berg LE, Favejee MM, Wens SC, et al. Safety and efficacy of exercise training in adults with Pompe disease: evalution of endurance, muscle strength and core stability before and after a 12 week training program. Orphanet J Rare Dis 2015;10:87. [Crossref] [PubMed]
- Kishnani PS, Hwu WL, Mandel H, et al. A retrospective, multinational, multicenter study on the natural history of infantile-onset Pompe disease. J Pediatr 2006;148:671-6. [Crossref] [PubMed]
- Martin AJ, Stern L, Yeates J, et al. Respiratory muscle training in Duchenne muscular dystrophy. Dev Med Child Neurol 1986;28:314-8. [Crossref] [PubMed]
- Wanke T, Toifl K, Merkle M, et al. Inspiratory muscle training in patients with Duchenne muscular dystrophy. Chest 1994;105:475-82. [Crossref] [PubMed]
- Yeldan I, Gurses HN, Yuksel H. Comparison study of chest physiotherapy home training programmes on respiratory functions in patients with muscular dystrophy. Clin Rehabil 2008;22:741-8. [Crossref] [PubMed]
- Jones HN, Crisp KD, Robey RR, et al. Respiratory muscle training (RMT) in late-onset Pompe disease (LOPD): Effects of training and detraining. Mol Genet Metab 2016;117:120-8. [Crossref] [PubMed]
- Jones HN, Moss T, Edwards L, et al. Increased inspiratory and expiratory muscle strength following respiratory muscle strength training (RMST) in two patients with late-onset Pompe disease. Mol Genet Metab 2011;104:417-20. [Crossref] [PubMed]
- Smith BK, Martin AD, Lawson LA, et al. Inspiratory muscle conditioning exercise and diaphragm gene therapy in Pompe disease: Clinical evidence of respiratory plasticity. Exp Neurol 2017;287:216-24. [Crossref] [PubMed]
- Tarnopolsky M, Zimmer A, Paikin J, et al. Creatine monohydrate and conjugated linoleic acid improve strength and body composition following resistance exercise in older adults. PLoS One 2007;2:e991. [Crossref] [PubMed]
- Kuk JL, Rotondi M, Sui X, et al. Individuals with obesity but no other metabolic risk factors are not at significantly elevated all-cause mortality risk in men and women. Clin Obes 2018;8:305-12. [Crossref] [PubMed]
- Haapanen-Niemi N, Miilunpalo S, Pasanen M, et al. Body mass index, physical inactivity and low level of physical fitness as determinants of all-cause and cardiovascular disease mortality--16 y follow-up of middle-aged and elderly men and women. Int J Obes Relat Metab Disord 2000;24:1465-74. [Crossref] [PubMed]
- Lee DC, Park I, Jun TW, et al. Physical activity and body mass index and their associations with the development of type 2 diabetes in korean men. Am J Epidemiol 2012;176:43-51. [Crossref] [PubMed]
- Ricketts TA, Sui X, Lavie CJ, et al. Addition of Cardiorespiratory Fitness Within an Obesity Risk Classification Model Identifies Men at Increased Risk of All-Cause Mortality. Am J Med 2016;129:536.e13-20. [Crossref] [PubMed]
- Samjoo IA, Safdar A, Hamadeh MJ, et al. The effect of endurance exercise on both skeletal muscle and systemic oxidative stress in previously sedentary obese men. Nutr Diabetes 2013;3:e88. [Crossref] [PubMed]
- Devries MC, Samjoo IA, Hamadeh MJ, et al. Endurance training modulates intramyocellular lipid compartmentalization and morphology in skeletal muscle of lean and obese women. J Clin Endocrinol Metab 2013;98:4852-62. [Crossref] [PubMed]
- Khan A, Weinstein Z, Hanley DA, et al. In vivo bone architecture in pompe disease using high-resolution peripheral computed tomography. JIMD Rep 2013;7:81-8. [Crossref] [PubMed]
- van den Berg LE, Zandbergen AA, van Capelle CI, et al. Low bone mass in Pompe disease: muscular strength as a predictor of bone mineral density. Bone 2010;47:643-9. [Crossref] [PubMed]
- Papadimas G, Terzis G, Papadopoulos C, et al. Bone density in patients with late onset Pompe disease. Int J Endocrinol Metab 2012;10:599-603. [Crossref] [PubMed]
- Willems HME, van den Heuvel E, Schoemaker RJW, et al. Diet and Exercise: a Match Made in Bone. Curr Osteoporos Rep 2017;15:555-63. [Crossref] [PubMed]
- Vico L, Lafage-Proust MH, Alexandre C. Effects of gravitational changes on the bone system in vitro and in vivo. Bone 1998;22:95S-100S. [Crossref] [PubMed]
- Pham HM, Nguyen ND, Center JR, et al. Contribution of Quadriceps Weakness to Fragility Fracture: A Prospective Study. J Bone Miner Res 2016;31:208-14. [Crossref] [PubMed]
- Francis GJ, Cook AE, Morrish DW, et al. What is the Fracture Risk in Patients at a Multidisciplinary Neuromuscular Clinic? J Neuromuscul Dis 2018;5:93-8. [Crossref] [PubMed]
- Pham HM, Nguyen SC, Ho-Le TP, et al. Association of Muscle Weakness With Post-Fracture Mortality in Older Men and Women: A 25-Year Prospective Study. J Bone Miner Res 2017;32:698-707. [Crossref] [PubMed]
- Areta JL, Burke LM, Camera DM, et al. Reduced resting skeletal muscle protein synthesis is rescued by resistance exercise and protein ingestion following short-term energy deficit. Am J Physiol Endocrinol Metab 2014;306:E989-97. [Crossref] [PubMed]
- Bell KE, Snijders T, Zulyniak M, et al. A whey protein-based multi-ingredient nutritional supplement stimulates gains in lean body mass and strength in healthy older men: A randomized controlled trial. PLoS One 2017;12:e0181387. [Crossref] [PubMed]
- Hartman JW, Tang JE, Wilkinson SB, et al. Consumption of fat-free fluid milk after resistance exercise promotes greater lean mass accretion than does consumption of soy or carbohydrate in young, novice, male weightlifters. Am J Clin Nutr 2007;86:373-81. [Crossref] [PubMed]
- Moore DR, Atherton PJ, Rennie MJ, et al. Resistance exercise enhances mTOR and MAPK signalling in human muscle over that seen at rest after bolus protein ingestion. Acta Physiol (Oxf) 2011;201:365-72. [Crossref] [PubMed]
- Welle S, Bhatt K, Thornton CA. Stimulation of myofibrillar synthesis by exercise is mediated by more efficient translation of mRNA. J Appl Physiol (1985) 1999;86:1220-5. [Crossref] [PubMed]
- van Vliet S, Shy EL, Abou Sawan S, et al. Consumption of whole eggs promotes greater stimulation of postexercise muscle protein synthesis than consumption of isonitrogenous amounts of egg whites in young men. Am J Clin Nutr 2017;106:1401-12. [Crossref] [PubMed]
- Tang JE, Moore DR, Kujbida GW, et al. Ingestion of whey hydrolysate, casein, or soy protein isolate: effects on mixed muscle protein synthesis at rest and following resistance exercise in young men. J Appl Physiol (1985) 2009;107:987-92. [Crossref] [PubMed]
- Wilkinson SB, Tarnopolsky MA, Macdonald MJ, et al. Consumption of fluid skim milk promotes greater muscle protein accretion after resistance exercise than does consumption of an isonitrogenous and isoenergetic soy-protein beverage. Am J Clin Nutr 2007;85:1031-40. [Crossref] [PubMed]
- Churchward-Venne TA, Burd NA, Mitchell CJ, et al. Supplementation of a suboptimal protein dose with leucine or essential amino acids: effects on myofibrillar protein synthesis at rest and following resistance exercise in men. J Physiol 2012;590:2751-65. [Crossref] [PubMed]
- Umpleby AM, Trend PS, Chubb D, et al. The effect of a high protein diet on leucine and alanine turnover in acid maltase deficiency. J Neurol Neurosurg Psychiatry 1989;52:954-61. [Crossref] [PubMed]
- Pascual JM, Roe CR. Systemic metabolic abnormalities in adult-onset acid maltase deficiency: beyond muscle glycogen accumulation. JAMA Neurol 2013;70:756-63. [Crossref] [PubMed]
- Lim JA, Li L, Shirihai OS, et al. Modulation of mTOR signaling as a strategy for the treatment of Pompe disease. EMBO Mol Med 2017;9:353-70. [Crossref] [PubMed]
- Martina JA, Diab HI, Lishu L, et al. The nutrient-responsive transcription factor TFE3 promotes autophagy, lysosomal biogenesis, and clearance of cellular debris. Sci Signal 2014;7:ra9. [Crossref] [PubMed]
- Spampanato C, Feeney E, Li L, et al. Transcription factor EB (TFEB) is a new therapeutic target for Pompe disease. EMBO Mol Med 2013;5:691-706. [Crossref] [PubMed]
- Shemesh A, Wang Y, Yang Y, et al. Suppression of mTORC1 activation in acid-alpha-glucosidase-deficient cells and mice is ameliorated by leucine supplementation. Am J Physiol Regul Integr Comp Physiol 2014;307:R1251-9. [Crossref] [PubMed]
- Slonim AE, Bulone L, Goldberg T, et al. Modification of the natural history of adult-onset acid maltase deficiency by nutrition and exercise therapy. Muscle Nerve 2007;35:70-7. [Crossref] [PubMed]
- Slonim AE, Coleman RA, McElligot MA, et al. Improvement of muscle function in acid maltase deficiency by high-protein therapy. Neurology 1983;33:34-8. [Crossref] [PubMed]
- Isaacs H, Savage N, Badenhorst M, et al. Acid maltase deficiency: a case study and review of the pathophysiological changes and proposed therapeutic measures. J Neurol Neurosurg Psychiatry 1986;49:1011-8. [Crossref] [PubMed]
- Demey HE, Van Meerbeeck JP, Vandewoude MF, et al. Respiratory insufficiency in acid maltase deficiency: the effect of high protein diet. JPEN J Parenter Enteral Nutr 1989;13:321-3. [Crossref] [PubMed]
- Margolis ML, Hill AR. Acid maltase deficiency in an adult. Evidence for improvement in respiratory function with high-protein dietary therapy. Am Rev Respir Dis 1986;134:328-31. [PubMed]
- Umpleby AM, Wiles CM, Trend PS, et al. Protein turnover in acid maltase deficiency before and after treatment with a high protein diet. J Neurol Neurosurg Psychiatry 1987;50:587-92. [Crossref] [PubMed]
- Mitchell WK, Phillips BE, Hill I, et al. Human skeletal muscle is refractory to the anabolic effects of leucine during the postprandial muscle-full period in older men. Clin Sci (Lond) 2017;131:2643-53. [Crossref] [PubMed]
- Papadimas GK, Terzis G, Spengos K, et al. Bone mineral density in adult patients with Pompe disease. Bone 2011;48:417-author reply 418-9. [Crossref] [PubMed]
- Walter MC, Lochmuller H, Reilich P, et al. Creatine monohydrate in muscular dystrophies: A double-blind, placebo-controlled clinical study. Neurology 2000;54:1848-50. [Crossref] [PubMed]
- Tarnopolsky MA, Mahoney DJ, Vajsar J, et al. Creatine monohydrate enhances strength and body composition in Duchenne muscular dystrophy. Neurology 2004;62:1771-7. [Crossref] [PubMed]
- Tarnopolsky M, Mahoney D, Thompson T, et al. Creatine monohydrate supplementation does not increase muscle strength, lean body mass, or muscle phosphocreatine in patients with myotonic dystrophy type 1. Muscle Nerve 2004;29:51-8. [Crossref] [PubMed]
- Walter MC, Reilich P, Lochmuller H, et al. Creatine monohydrate in myotonic dystrophy: a double-blind, placebo-controlled clinical study. J Neurol 2002;249:1717-22. [Crossref] [PubMed]
- Kley RA, Tarnopolsky MA, Vorgerd M. Creatine for treating muscle disorders. Cochrane Database Syst Rev 2013.CD004760. [PubMed]
- Zou X, Meng J, Li L, et al. Acetoacetate Accelerates Muscle Regeneration and Ameliorates Muscular Dystrophy in Mice. J Biol Chem 2016;291:2181-95. [Crossref] [PubMed]
- Youm YH, Nguyen KY, Grant RW, et al. The ketone metabolite beta-hydroxybutyrate blocks NLRP3 inflammasome-mediated inflammatory disease. Nat Med 2015;21:263-9. [Crossref] [PubMed]
- Finn PF, Dice JF. Ketone bodies stimulate chaperone-mediated autophagy. J Biol Chem 2005;280:25864-70. [Crossref] [PubMed]
- Douillard-Guilloux G, Raben N, Takikita S, et al. Restoration of muscle functionality by genetic suppression of glycogen synthesis in a murine model of Pompe disease. Hum Mol Genet 2010;19:684-96. [Crossref] [PubMed]
- Garbow JR, Doherty JM, Schugar RC, et al. Hepatic steatosis, inflammation, and ER stress in mice maintained long term on a very low-carbohydrate ketogenic diet. Am J Physiol Gastrointest Liver Physiol 2011;300:G956-67. [Crossref] [PubMed]
- Jornayvaz FR, Jurczak MJ, Lee HY, et al. A high-fat, ketogenic diet causes hepatic insulin resistance in mice, despite increasing energy expenditure and preventing weight gain. Am J Physiol Endocrinol Metab 2010;299:E808-15. [Crossref] [PubMed]
- Ding D, Lawson KD, Kolbe-Alexander TL, et al. The economic burden of physical inactivity: a global analysis of major non-communicable diseases. Lancet 2016;388:1311-24. [Crossref] [PubMed]
- Lee IM, Shiroma EJ, Lobelo F, et al. Effect of physical inactivity on major non-communicable diseases worldwide: an analysis of burden of disease and life expectancy. Lancet 2012;380:219-29. [Crossref] [PubMed]
- Arem H, Moore SC, Patel A, et al. Leisure time physical activity and mortality: a detailed pooled analysis of the dose-response relationship. JAMA Intern Med 2015;175:959-67. [Crossref] [PubMed]
- Lee DC, Brellenthin AG, Thompson PD, et al. Running as a Key Lifestyle Medicine for Longevity. Prog Cardiovasc Dis 2017;60:45-55. [Crossref] [PubMed]
- Reimers CD, Knapp G, Reimers AK. Does physical activity increase life expectancy? A review of the literature. J Aging Res 2012;2012:243958. [Crossref] [PubMed]
- Stessman J, Hammerman-Rozenberg R, Cohen A, et al. Physical Activity, Function, and Longevity Among the Very OldPhysical Activity Among the Very Old. Arch Int Med 2009;169:1476-83. [Crossref]
- Chodzko-Zajko WJ, Proctor DN, Fiatarone Singh MA, et al. Exercise and Physical Activity for Older Adults. Medicine & Science in Sports & Exercise 2009;41:1510-30. [Crossref] [PubMed]
- Powell KE, Paluch AE, Blair SN. Physical Activity for Health: What Kind? How Much? How Intense? On Top of What? Annu Rev Public Health 2011;32:349-65. [Crossref] [PubMed]
- Moore SC, Lee IM, Weiderpass E, et al. Association of Leisure-Time Physical Activity With Risk of 26 Types of Cancer in 1.44 Million AdultsLeisure-Time Physical Activity and Risk of 26 Types of Cancer Leisure-Time Physical Activity and Risk of 26 Types of Cancer. JAMA Int Med 2016;176:816-25. [Crossref]
- Nelson ME, Rejeski WJ, Blair SN, et al. Physical Activity and Public Health in Older Adults: Recommendation from the American College of Sports Medicine and the American Heart Association. Medicine & Science in Sports & Exercise 2007;39:1435-45. [Crossref] [PubMed]
- Piercy KL, Troiano RP, Ballard RM, et al. The Physical Activity Guidelines for Americans. JAMA 2018;320:2020-8. [Crossref] [PubMed]
- Warburton DE, Charlesworth S, Ivey A, et al. A systematic review of the evidence for Canada's Physical Activity Guidelines for Adults. Int J Behav Nutr Phys Act 2010;7:39. [Crossref] [PubMed]
- Swain DP, Franklin BA. Comparison of Cardioprotective Benefits of Vigorous Versus Moderate Intensity Aerobic Exercise. Am J Cardiol 2006;97:141-7. [Crossref] [PubMed]
- Wen CP, Wai JPM, Tsai MK, et al. Minimum amount of physical activity for reduced mortality and extended life expectancy: a prospective cohort study. Lancet 2011;378:1244-53. [Crossref] [PubMed]
- Kodama S, Saito K, Tanaka S, et al. Cardiorespiratory Fitness as a Quantitative Predictor of All-Cause Mortality and Cardiovascular Events in Healthy Men and Women: A Meta-analysis. JAMA 2009;301:2024-35. [Crossref] [PubMed]
- Bassett DR. Limiting factors for maximum oxygen uptake and determinants of endurance performance. Medicine & Science in Sports & Exercise 2000;32:70. [Crossref] [PubMed]
- Fitzgerald SJ, Barlow CE, Kampert JB, et al. Muscular Fitness and All-Cause Mortality: Prospective Observations. J Phys Act Health 2004;1:7-18. [Crossref]
- Katzmarzyk PT, Craig CL. Musculoskeletal fitness and risk of mortality. Med Sci Sports Exerc 2002;34:740-4. [Crossref] [PubMed]
- Cermak NM, Cermak NM, Res PT, et al. Protein supplementation augments the adaptive response of skeletal muscle to resistance-type exercise training: a meta-analysis. Am J Clin Nutr 2012;96:1454-64. [Crossref] [PubMed]
- Konopka AR, Harber MP. Skeletal muscle hypertrophy after aerobic exercise training. Exerc Sport Sci Rev 2014;42:53-61. [Crossref] [PubMed]
- Hagerman FC, Walsh S, Staron R, et al. Effects of high-intensity resistance training on untrained older men. I. Strength, cardiovascular, and metabolic responses. J Gerontol A Biol Sci Med Sci 2000;55:B336-46. [Crossref] [PubMed]
- Liu CJ, Latham NK. Progressive resistance strength training for improving physical function in older adults. Cochrane Database Syst Rev 2009.CD002759. [PubMed]
- Hyttinen JMT, Amadio M, Viiri J, et al. Clearance of misfolded and aggregated proteins by aggrephagy and implications for aggregation diseases. Ageing Res Rev 2014;18:16-28. [Crossref] [PubMed]
- Nilsson MI, MacNeil LG, Kitaoka Y, et al. Combined aerobic exercise and enzyme replacement therapy rejuvenates the mitochondrial-lysosomal axis and alleviates autophagic blockage in Pompe disease. Free Radic Biol Med 2015;87:98-112. [Crossref] [PubMed]
- Terman A, Kurz T, Navratil M, et al. Mitochondrial turnover and aging of long-lived postmitotic cells: the mitochondrial-lysosomal axis theory of aging. Antioxid Redox Signal 2010;12:503-35. [Crossref] [PubMed]
- Nilsson MI, Tarnopolsky MA. Mitochondria and Aging: The Role of Exercise as a Countermeasure. Biology 2019;8:40. [Crossref] [PubMed]
- Nilsson MI, Bourgeois JM, Nederveen JP, et al. Lifelong aerobic exercise protects against inflammaging and cancer. PLoS One 2019;14:e0210863. [Crossref] [PubMed]
- Garatachea N, Pareja-Galeano H, Sanchis-Gomar F, et al. Exercise attenuates the major hallmarks of aging. Rejuvenation Res 2015;18:57-89. [Crossref] [PubMed]
- Gleeson M, Bishop NC, Stensel DJ, et al. The anti-inflammatory effects of exercise: mechanisms and implications for the prevention and treatment of disease. Nat Rev Immunol 2011;11:607. [Crossref] [PubMed]
- Gounder SS, Kannan S, Devadoss D, et al. Impaired transcriptional activity of Nrf2 in age-related myocardial oxidative stress is reversible by moderate exercise training. PLoS One 2012;7:e45697. [Crossref] [PubMed]
- He C, Bassik MC, Moresi V, et al. Exercise-induced BCL2-regulated autophagy is required for muscle glucose homeostasis. Nature 2012;481:511-5. [Crossref] [PubMed]
- Ji LL, Gomez-Cabrera MC, Vina J. Role of nuclear factor kB and mitogen-activated protein kinase signaling in exercise-induced antioxidant enzyme adaptation. Appl Physiol Nutr Metab 2007;32:930-5. [Crossref] [PubMed]
- MacNeil L, Safdar A, Baker S, et al. Eccentric Exercise Affects NRF2-mediated Oxidative Stress Response in Skeletal Muscle by Increasing Nuclear NRF2 Content. Med Sci Sports Exerc 2011;43:383. [Crossref]
- Pagano AF, Py G, Bernardi H, et al. Autophagy and Protein Turnover Signaling in Slow-Twitch Muscle during Exercise. Medicine & Science in Sports & Exercise 2014;46:1314-25. [Crossref] [PubMed]
- Piantadosi CA, Suliman HB. Redox regulation of mitochondrial biogenesis. Free Radic Biol Med 2012;53:2043-53. [Crossref] [PubMed]
- Powers SK, Jackson MJ. Exercise-induced oxidative stress: cellular mechanisms and impact on muscle force production. Physiol Rev 2008;88:1243-76. [Crossref] [PubMed]
- Powers SK, Nelson WB, Hudson MB. Exercise-induced oxidative stress in humans: Cause and consequences. Free Radic Biol Med 2011;51:942-50. [Crossref] [PubMed]
- Ristow M, Zarse K. How increased oxidative stress promotes longevity and metabolic health: The concept of mitochondrial hormesis (mitohormesis). Exp Gerontol 2010;45:410-8. [Crossref] [PubMed]
- Tapia PC. Sublethal mitochondrial stress with an attendant stoichiometric augmentation of reactive oxygen species may precipitate many of the beneficial alterations in cellular physiology produced by caloric restriction, intermittent fasting, exercise and dietary phytonutrients: "Mitohormesis" for health and vitality. Med Hypotheses 2006;66:832-43. [Crossref] [PubMed]
- Case LE, Beckemeyer AA, Kishnani PS. Infantile Pompe disease on ERT-Update on clinical presentation, musculoskeletal management, and exercise considerations. Am J Med Genet C Semin Med Genet 2012;160C:69-79. [Crossref] [PubMed]
- Cupler EJ, Berger KI, Leshner RT, et al. Consensus treatment recommendations for late-onset Pompe disease. Muscle Nerve 2012;45:319-33. [Crossref] [PubMed]
- Kishnani PS, Steiner RD, Bali D, et al. Genet Med 2006;8:267-88. [Crossref] [PubMed]
- ACSM. Guidelines for exercise testing and prescription. Philadelphia: Lippincott Williams & Wilkins, 2010.
- Strothotte S, Strigl-Pill N, Grunert B, et al. Enzyme replacement therapy with alglucosidase alfa in 44 patients with late-onset glycogen storage disease type 2: 12-month results of an observational clinical trial. J Neurol 2010;257:91-7. [Crossref] [PubMed]
- Williams PT. Dose-response relationship of physical activity to premature and total all-cause and cardiovascular disease mortality in walkers. PLoS One 2013;8:e78777. [Crossref] [PubMed]
- Morrato EH, Hill JO, Wyatt HR, et al. Physical activity in U.S. adults with diabetes and at risk for developing diabetes, 2003. Diabetes Care 2007;30:203-9. [Crossref] [PubMed]
- de Vries JM, Hagemans ML, Bussmann JB, et al. Fatigue in neuromuscular disorders: focus on Guillain-Barre syndrome and Pompe disease. Cell Mol Life Sci 2010;67:701-13. [Crossref] [PubMed]
- Nilsson MI, Samjoo IA, Hettinga BP, et al. Aerobic training as an adjunctive therapy to enzyme replacement in Pompe disease. Mol Genet Metab 2012;107:469-79. [Crossref] [PubMed]
- van der Ploeg AT, Clemens PR, Corzo D, et al. A randomized study of alglucosidase alfa in late-onset Pompe's disease. N Engl J Med 2010;362:1396-406. [Crossref] [PubMed]
- Wenninger S, Greckl E, Babacic H, et al. Safety and efficacy of short- and long-term inspiratory muscle training in late-onset Pompe disease (LOPD): a pilot study. J Neurol 2019;266:133-47. [Crossref] [PubMed]