Exenatide reduces cardiomyocyte apoptosis by stimulating adiponectin secretion and activating APPL1-AMPK-PPARα axis
Introduction
Diabetic cardiomyopathy (DC), which was first proposed by Robler in 1972, is a specific myocardial disease generally observed in diabetic patients and also one of the main causes for diabetic death. DC is primarily manifested as ventricular diastolic dysfunction that causes heart failure. Although the pathogenesis has not been fully clarified, the current studies have shown that cardiomyocyte apoptosis is the major chronic pathological process of DC (1,2), and thus the inhibition of cardiomyocyte apoptosis is a key for the prevention and treatment of DC.
Adiponectin, an adipokine with the highest level in the blood, has anti-inflammatory and anti-atherosclerotic effects. Studies have suggested that adiponectin plays an important role in heart failure and ventricular remodeling, and it is closely associated with cardiovascular complications in diabetes mellitus (3,4). In addition to adipocytes, adiponectin is also secreted by cardiomyocytes, but its physiological effects are unclear (5,6). In a previous study, glucagon-like peptide-1 (GLP-1) was found to promote adiponectin secretion in vivo (7). Exenatide is a GLP-1 receptor agonist mainly used to decrease blood glucose and reduce body weight. Our preliminary animal study demonstrated that exenatide could stimulate the secretion of adiponectin to activate APPL1, AMPK and PPARα signaling pathways in sequence, and thus up-regulate the expression of APPL1, AMPK and PPARα and inhibit the activity of NF-κB, eventually suppressing diabetic cardiomyocyte apoptosis and improving heart function. Such anti-cardiomyocyte apoptosis effects of exenatide are independent of its anti-diabetic effect. Therefore, we hypothesized that diabetic cardiomyocyte apoptosis was regulated by adiponectin and APPL1-AMPK-PPARα axis (Figure 1). To test this hypothesis, we cultured rat cardiomyocytes using high-dose glucose/palmitate followed by treatment with exenatide. Then, we measured the anti-apoptotic effect of exenatide and the changes of adiponectin expression in the cultured cardiomyocytes.
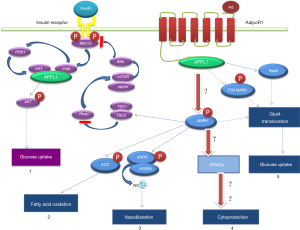
Methods
Cardiomyocyte culture
Neonatal SD rats (age: 2–4 days) were washed with running tap water, then fixed on a super clean bench after skin disinfection with 75% alcohol cotton balls, anesthetized, and sacrificed by cervical dislocation. Then, the heart was collected by aseptic technique, placed into a culture dish containing 4 °C precooled D-Hanks buffer, and then washed by clamping with notched forceps for 3–4 times until the blood in the heart was removed completely. Thereafter, the redundant tissues were removed, and the trimmed heart was sheared into 1 mm tissue blocks using ophthalmic scissors. The solution containing the tissue blocks was transferred into a centrifuge tube and centrifuged at 1,000 rpm/min for 5 min. The supernatant was discarded. The obtained tissues were added to 1.5 mL of digestive enzyme, homogenized by blowing, and digested in a 37 °C water bath kettle for 5 min. The supernatant was discarded and 2 mL of digestive enzyme was added. The centrifuge tube was tightly sealed, gently shaken, placed into a 37 °C water bath kettle and shaken once every 3 min. After 20 min digestion, the supernatant was collected, and the culture medium was added into the collecting tube to terminate the digestion. After the digestion was repeated for 3–4 times, the collected supernatant was centrifuged at 1,000 rpm/min for 5 min. The supernatant was discarded, and the obtained tissues were added to a proper amount of culture medium, re-suspended and preserved in a 37 °C, 5% CO2 environment for 30 min until the cells adhered. Finally, the supernatant was collected, inoculated in a new culture dish and cultured with low-glucose DMEM culture medium + 10% fetal bovine serum (FBS).
Construction of APPL1-overexpressing and APPL1 knock-down adenovirus
We searched the APPL1 sequence information and designed the knock-down sites: 5'- GCCGCAATTAATAGGTACAGC-3'. The sequencing and adenovirus plasmids were completed by Shanghai GenePharma Co., Ltd (China).
Cell grouping
The primary cells obtained were inoculated and cultured for 48 h, and then the culture medium was replaced and the cells were grouped and subjected to the following interventions: (I) control group (group C): low-glucose (5.5 mmol/L) DMEM culture medium + 10% FBS; (II) diabetic group (group D): high-glucose (25 mmol/L) DMEM culture medium + 0.5 M palmitate + 10% FBS; (III) diabetic + exenatide treatment group (group DE): high-glucose DMEM culture medium + 0.5 M palmitate + 10% FBS (48 h culture) + 3 µg exenatide (12 h culture); (IV) diabetic + exenatide treatment + APPL1 overexpression group (group OE): high-glucose DMEM culture medium + 0.5 M palmitate + 10% FBS + 2 µL APPL1-overexpressing adenovirus (48 h culture) + 3 µg exenatide (12 h culture); (V) diabetic + exenatide treatment + APPL1 knock-down group (group KD): high-glucose DMEM culture medium + 0.5 M palmitate + 10% FBS + 2 µL APPL1-interfering (knock-down) adenovirus (48 h culture) + 3 µg exenatide (12 h culture).
Western blotting
The cultured cardiomyocytes were washed with PBS and then scraped into centrifuge tubes. Fifty microliters of lysis buffer and 0.5 µL of proteinase inhibitor were added to each tube. The tubes were placed on ice for 30 min and then centrifuged. The supernatant was collected. After the protein concentration was measured, 20 µL of the supernatant was loaded for polyacrylamide gel electrophoresis 20 min at 80 V and then 2 h at 120 V. Subsequently, the protein on the separation gel was transferred onto methanol-treated PVDF membranes (200 mA, 90 min) using the semi-dry transfer method. The membranes were blocked with 5% defatted milk powder (Sigma) for 2 h. Primary antibodies were added (APPL1, 1:1,000; AMPK, 1:1,000; p-AMPK, 1:500; PPARα, 1:1,000; NF-κB p65, 1:500) along with rabbit anti-rat β-actin antibody (1:400, Santa Cruz, ZSGB-BIO), and then these membranes were incubated overnight in a 4 °C refrigerator. After washing with TBST, they were incubated with HRP-labeled goat anti-rabbit IgG antibody (1:5,000) at 37 °C on a 50 r/min shaking table for 90 min, and then washed with TBST again. Thereafter, the conjugated HRP-labeled secondary antibodies were detected with a chemiluminescence kit, followed by X-ray development and Quality One software analysis. The optimal density (OD) of the target band/β-actin was used as the quantitative indicator reflecting the intensity. Three samples were measured and then the measurement results were averaged in each group. The expression changes of APPL1, AMPK, p-AMPK, PPARα and NF-κB (p65) in cardiomyocytes were detected.
Detection of cardiomyocyte apoptosis
The cardiomyocyte slides were washed with PBS for 3 times, fixed with 4% paraformaldehyde for 2 h, blocked with 3% hydrogen peroxide-methanol, permeated with 0.2% Triton-X-100, coated with TUNEL buffer, incubated overnight at 4 °C, and washed again with PBS for 3 times. These slides were then reacted with POD at 37 °C for 30 min, developed with DAB at room temperature, washed with purified water, counterstained with hematoxylin for 2 min, washed with PBS and mounted. Seven positive fields were photographed under light microscope (×400) according to the distribution of apoptotic cells in each group; 100 apoptotic cardiomyocytes were counted in each field, and then the apoptosis rate (i.e., the percentage of the mean number of apoptotic cells) was calculated.
Detection of adiponectin in the supernatant of cardiomyocyte culture (ELISA)
The primary standard substance in the kit was diluted at a gradient. The ELISA plate containing the sample and standard substance was equilibrated at room temperature for 30 min, incubated at room temperature for 1 h, and washed with the liquid detergent for 3 times. Then, biotin-labeled antibody was added to the sample wells, but not to the standard wells, and the plate was covered with a film for blockage, incubated at room temperature for 1 h, and washed again with the liquid detergent for 3 times. Next, 50 µL of streptavidin-HRP was added, and the plate was incubated at room temperature for 30 min and washed with the liquid detergent for 3 times. Finally, the developer was added, and the plate was developed protecting from light at room temperature for 30 min. The Stop buffer was added to terminate the reaction. The zeroing was performed with the blank control. The OD in each well was measured at 450 nm, the standard curve was plotted and the sample concentration was calculated.
Statistical analysis
The experimental data with homogeneity of variance were presented as the mean ± standard deviation and those with heterogeneity of variance as an interquartile range. SPSS 20.0 statistical software was used for statistical analysis. Kruskal-Wallis non-parametric test and one-factor analysis of variance were employed. P<0.05 indicated a statistically significant difference.
Results
Adiponectin secretion level in cardiomyocytes
In group C, the adiponectin level in the cell culture supernatant was 7.40±0.29; it was significantly decreased in group D (6.72±0.19) (P<0.05). Compared to group D, the adiponectin level was markedly increased in all group DE (9.40±0.16), group OE (8.70±0.34) and group KD (9.30±0.30) (P<0.05); however, the difference between group KD and group DE was not statistically significant (P>0.05) (Figure 2). Our results verified that adiponectin could be secreted by primary cardiomyocytes, and exenatide promoted the adiponectin secretion of cardiomyocytes after high-glucose intervention.
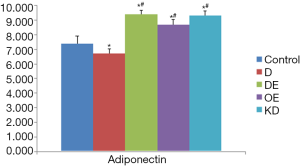
Detection of cardiomyocyte apoptosis
Cardiomyocyte apoptosis was detected by TUNEL staining in each group (Figure 3A). In group C, the apoptosis rate of cardiomyocytes was 3% to 5%. Compared to group C, it was significantly increased in group D (34% to 42%). Compared to group D, it was markedly decreased in both group DE (20% to 29%) and group OE (18% to 24%). Compared to group OE, it was increased in group KD (43% to 52%). All of the differences were statistically significant (P<0.05) (Figure 3B). As we know, a high concentration of glucose will increase the apoptosis rate of cardiomyocytes, but exenatide intervention can decrease it, even if the mechanism is not clarified.
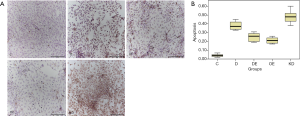
Cardiomyocyte culture and APPL1 interference with adenoviruses
Nearly all cardiomyocytes adhered to the wall at 24 h after culture, irregular pulsation was observed at 48 h, and massive pulsation was observed at 72 h. For interference of APPL1, we constructed APPL1 siRNA adenoviruses. After transfecting the cardiomyocytes with adenoviruses for 48 h, we detected the expression of APPL1 by Western blotting (Figure 4).
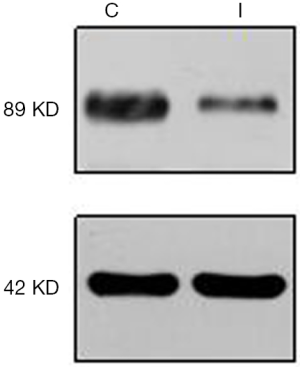
Protein expression level in cardiomyocytes
We detected the expression levels of APPL1, p-AMPK, T-AMPK, PPARα and NF-κB (p65) in cardiomyocytes. The results showed that the expression levels of APPL1, p-AMPK and PPARα in group D were lower than those in group C, while that of NF-κB (p65) was higher. The expression levels of APPL1, p-AMPK and PPARα in group DE and group OE were significantly higher than those in group D, while that of NF-κB (p65) was lower. The expression levels of p-AMPK and PPARα in group KD were lower than those in group OE, while that of NF-κB (p65) was higher (Figure 5). Our results showed that the expression levels of APPL1, p-AMPK and PPARα had the same change trend as the adiponectin level, but NF-κB (p65) demonstrated an opposite trend. Therefore, we believe that APPL1, p-AMPK and PPARα jointly participate in the adiponectin signaling pathway. The expression levels of p-AMPK and PPARα were significantly reduced after APPL1 knock-down, but obviously increased after APPL1 overexpression. These findings suggest that APPL1 has a key regulating effect on the “APPL1-AMPK-PPARα” anti-apoptosis signaling axis in cardiomyocytes.
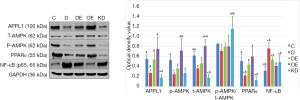
Discussion
Type 2 diabetes mellitus is a common disease in the contemporary society. Diabetic cardiovascular diseases are the most frequently reported cause of death in patients with type 2 diabetes mellitus. In addition to atherosclerosis, specific cardiomyopathy can induce energy dysmetabolism, structural abnormality and functional changes of cardiomyocytes, and it is also the major cause of diabetic cardiovascular diseases. The recent studies have revealed that DC is an important risk factor for heart dysfunction, heart failure and even death in diabetic patients. Cardiomyocyte apoptosis is the main cause of DC, but the mechanism is unclear, and there is a lack of effective therapies for DC.
Adiponectin is a protein secreted by adipocytes. A marked reduction of adiponectin level is found in patients with diabetes mellitus, coronary artery disease or hypertension (8,9). An elevated adiponectin level can suppress the oxidative stress of cardiomyocytes caused by hyperglycemic exposure and relieve the hypertrophy of cardiomyocytes (10). Pischon et al. found an association between a high adiponectin level and a low risk of myocardial infarction in the mankind (11). In ischemia-reperfusion animal models or in vitro cell studies, the involvement of adiponectin in the inhibition of cardiomyocyte apoptosis has been confirmed (12,13). These studies have proven the adiponectin-mediated heart protection, but the mechanism has not been fully clarified and there are no relevant diabetic animal studies reported. The expression of adiponectin gene in other types of cells, including hepatocytes, skeletal muscle cells and osteoblasts, has been revealed by some recent studies (14,15). Adiponectin synthesis and secretion are also found in isolated mouse and human cardiomyocytes (16-18). In this study, we detected adiponectin in the supernatant of neonatal rat cardiomyocyte culture, and adiponectin might transmit signals via adiponectin receptors (mainly AdipoR1) on cardiomyocytes, which indicates that cardiomyocytes may secrete adiponectin to promote self-protection; however, such a finding remains to be explored.
APPL1 can bind with AdipoR1 to mediate several adiponectin signaling pathways in cells (12,19). Numerous studies have shown that APPL1 overexpression or inhibition can enhance or suppress the adiponectin signaling pathways (20,21), indicating that APPL1 plays a key role in regulating adiponectin signaling pathways. Fang et al. observed that the binding of APPL1 to adiponectin receptors activated AMPK (a downstream protein) and inhibited NF-κB to achieve the anti-cardiomyocyte apoptosis effect of adiponectin, and APPL1 played an essential role in this process (12). Piñeiro et al. found that adiponectin could induce AMPK phosphorylation in cardiomyocytes treated with high-dose glucose/lipid (18). Other studies demonstrated that after binding to receptors, adiponectin could activate AMPK and PPARα to down-regulate oxidative stress and inhibit inflammatory reactions and apoptosis (22,23). PPARα is an important downstream molecule of AMPK and also a critical protein in adiponectin signaling pathways. In a preliminary study, we found that PPARα could inhibit NF-κB in the adiponectin signaling pathway and directly participate in preventing the apoptosis of diabetic cardiomyocytes (24). A hypothesis was proposed that the “APPL1-AMPK-PPARα” axis of adiponectin regulated cardiomyocyte apoptosis, which prompted the previous animal experimental study. This study demonstrated that compared with rats with a low serum level of adiponectin, the up-regulated expression of APPL1, AMPK and PPARα was observed and cardiomyocyte apoptosis was significantly reduced in diabetic rats with a high serum level of adiponectin (25). The results suggest that the “APPL1-AMPK-PPARα” axis is involved in the regulation of cardiomyocyte apoptosis.
GLP-1 is an insulin-secreting agonist which is secreted by L cells in the distal jejunum, ileum and colon. GLP-1/GLP-1R binding can stimulate insulin secretion, alleviate insulin resistance and reduce glucagon secretion. In addition to the classic hypoglycemic effect, the cell protective role of GLP-1 has attracted increasing attentions. Based on a large number of studies in ischemia-reperfusion rat heart models, GLP-1 therapy can markedly reduce cardiomyocyte apoptosis and decrease the infarction area (26). The cardiovascular protection observed with GLP-1 is independent to the glucose level in the peripheral blood (7). Many studies have suggested that GLP-1 or GLP-1 receptor agonists can promote adiponectin secretion (27,28), and some investigators found by injecting exendin-4 expressing adenovirus into mice that exendin-4 could directly promote adiponectin secretion (29). In a preliminary study, we observed in exenatide-treated diabetic SD rats that the serum adiponectin level was elevated, the expression of APPL1, AMPK and PPARα in heart tissues was up-regulated, the expression of NF-κB was suppressed, cardiomyocyte apoptosis was reduced, and heart function was improved (25). Considering that exenatide might improve heart function by lowering blood glucose and lipid levels, we used primary cardiomyocytes collected from a neonatal rat to test this hypothesis.
Using primary cardiomyocytes collected from a neonatal SD rat, we found the presence of adiponectin in the supernatant of cardiomyocyte culture, which further proves that cardiomyocytes can secrete adiponectin. We simulated the hyperglycemic and hyperlipidemic environment in diabetes mellitus using high-dose glucose/palmitate culture medium. The results showed that compared to normal cells, the apoptosis rate of cardiomyocytes was increased and the adiponectin level was decreased in the hyperglycemic and hyperlipidemic environment. After treating the cells with exenatide, the apoptosis rate was decreased and the adiponectin level was increased (P<0.05), the expression of APPL1, p-AMPK and PPARα was up-regulated and that of NF-κB was down-regulated (P<0.05), which is consistent with the results of the diabetic animal studies (25). In group OE, the above trend was more prominent in the cardiomyocytes transfected with APPL1 overexpression adenovirus. On the contrary, the expression of t-AMPK, p-AMPK, PPARα were decreased in the cardiomyocytes transfected with APPL1-interfering (knock-down) adenovirus (group KD), though the level of adiponectin was not significantly different from group DE. Cardiomyocytes apoptosis rate was also significantly increased compared with group DE (P<0.05). These findings suggest that APPL1 has a key regulating effect on the “APPL1-AMPK-PPARα” anti-apoptosis signaling axis. We noticed that the expression level of t-AMPK and p-AMPK were similarly changed among the groups compared with animal studies, but the ratio of p-AMPK/t-AMPK was not entirely consistent with those in animal studies (25). Our analysis results show that the changes in p-AMPK are simply attributed to the change in total AMPK expression.
Our experimental results have demonstrated that exenatide can activate the “APPL1-AMPK-PPARα” anti-apoptosis signaling axis by promoting adiponectin expression in cardiomyocytes and reducing the apoptosis of diabetic cardiomyocytes, thus protecting cardiomyocytes. However, NF-κB plays a complex role in the apoptosis signaling pathway, and the specific mechanism of “APPL1-AMPK-PPARα” axis regulating NF-κB is not yet clear. The subsequent study is needed to clarify this issue.
Acknowledgments
Funding: This work was supported by Chinese Society of Endocrinology (No. 13020120397) and National Natural Science Foundation of China (No. 81670711).
Footnote
Conflicts of Interest: The authors have no conflicts of interest to declare.
Ethical Statement: The authors are accountable for all aspects of the work in ensuring that questions related to the accuracy or integrity of any part of the work are appropriately investigated and resolved.
References
- Battiprolu PK, Hojayev B, Jiang N, et al. Metabolic stress-induced activation of FoxO1 triggers diabetic cardiomyopathy in mice. J Clin Invest 2012;122:1109-18. [Crossref] [PubMed]
- Rubin J, Matsushita K, Ballantyne CM, et al. Chronic hyperglycemia and subclinical myocardial injury. J Am Coll Cardiol 2012;59:484-9. [Crossref] [PubMed]
- Mossalam M, Jeong JH, Abel ED, et al. Reversal of oxidative stress in endothelial cells by controlled release of adiponectin. J Control Release 2008;130:234-7. [Crossref] [PubMed]
- Yokota T, Oritani K, Takahashi I, et al. Adiponectin, a new member of the family of soluble defense collagens, negatively regulates the growth of myelomonocytic progenitors and the functions of macrophages. Blood 2000;96:1723-32. [PubMed]
- Wang Y, Lau WB, Gao E, et al. Cardiomyocyte-derived adiponectin is biologically active in protecting against myocardial ischemia-reperfusion injury. Am J Physiol Endocrinol Metab 2010;298:E663-70. [Crossref] [PubMed]
- Guo Bingyan, Li Yongjun, Rui Han, et al. Angiotensin II upregulation of cardiomyocyte adiponectin production is nitric oxide/cyclic GMP dependent. Am J Med Sci 2011;341:350-5. [Crossref] [PubMed]
- Ravassa S, Zudaire A, Carr RD, et al. Antiapoptotic effects of GLP-1 in murine HL-1 cardiomyocytes. Am J Physiol Heart Circ Physiol 2011;300:H1361-72. [Crossref] [PubMed]
- Tao Ling, Gao Erhe, Jiao Xiangying, et al. Adiponectin cardioprotection after myocardial ischemia/reperfusion involves the reduction of oxidative/nitrative stress. Circulation 2007;115:1408-16. [Crossref] [PubMed]
- Jee SH, Ahn CW, Park JS, et al. Serum adiponectin and type 2 diabetes: a 6-year follow-up cohort study. Diabetes Metab J 2013;37:252-61. [Crossref] [PubMed]
- Li H, Yao W, Irwin M, et al. Adiponectin ameliorates hyperglycemia-induced cardiac hypertrophy and dysfunction by concomitantly activating Nrf2 and Brg1. Free Radic Biol Med 2015;84:311-21. [Crossref] [PubMed]
- Pischon T, Girman C, Hotamisligil GS, et al. Plasma adiponectin levels and risk of myocardial infarction in men. JAMA 2004;291:1730-7. [Crossref] [PubMed]
- Fang X, Palanivel R, Cresser J, et al. An APPL1-AMPK signaling axis mediates beneficial metabolic effects of adiponectin in the heart. Am J Physiol Endocrinol Metab 2010;299:E721-9. [Crossref] [PubMed]
- Shibata R, Izumiya Y, Sato K, et al. Adiponectin protects against the development of systolic dysfunction following myocardial infarction. J Mol Cell Cardiol 2007;42:1065-74. [Crossref] [PubMed]
- Liu Y, Chewchuk S, Lavigne C, et al. Functional significance of skeletal muscle adiponectin production, changes in animal models of obesity and diabetes, and regulation by rosiglitazone treatment. Am J Physiol Endocrinol Metab 2009;297:E657-64. [Crossref] [PubMed]
- Shinoda Y, Yamaguchi M, Ogata N, et al. Regulation of bone formation by adiponectin through autocrine/paracrine and endocrine pathways. J Cell Biochem 2006;99:196-208. [Crossref] [PubMed]
- Ding G, Qin Q, He N, et al. Adiponectin and its receptors are expressed in adult ventricular cardiomyocytes and upregulated by activation of peroxisome proliferator-activated receptor gamma. J Mol Cell Cardiol 2007;43:73-84. [Crossref] [PubMed]
- Guo Z, Xia Z, Yuen VG, et al. Cardiac expression of adiponectin and its receptors in streptozotocin-induced diabetic rats. Metabolism 2007;56:1363-71. [Crossref] [PubMed]
- Piñeiro R, Iglesias M, Gallego R, et al. Adiponectin is synthesized and secreted by human and murine cardiomyocytes. FEBS Lett 2005;579:5163-9. [Crossref] [PubMed]
- Chandrasekar B, Boylston WH, Venkatachalam K, et al. Adiponectin blocks interleukin-18-mediated endothelial cell death via APPL1-dependent AMP-activated protein kinase (AMPK) activation and IKK/NF-kappa B/PTEN suppression. J Biol Chem 2008;283:24889-98. [Crossref] [PubMed]
- Deepa SS, Dong LQ. APPL1: role in adiponectin signaling and beyond. Am J Physiol Endocrinol Metab 2009;296:E22-36. [Crossref] [PubMed]
- Mao X, Kikani CK, Riojas RA, et al. APPL1 binds to adiponectin receptors and mediates adiponectin signalling and function. Nat Cell Biol 2006;8:516-23. [Crossref] [PubMed]
- Bråkenhielm E, Veitonmäki N, Cao R, et al. Adiponectin-induced antiangiogenesis and antitumor activity involve caspase-mediated endothelial cell apoptosis. Proc Natl Acad Sci U S A 2004;101:2476-81. [Crossref] [PubMed]
- Yoon MJ, Lee GY, Chung JJ, et al. Adiponectin increases fatty acid oxidation in skeletal muscle cells by sequential activation of AMP-activated protein kinase, p38 mitogen-activated protein kinase, and peroxisome proliferator-activated receptor alpha. Diabetes 2006;55:2562-70. [Crossref] [PubMed]
- Nan WQ, Shan TQ, Qian X, et al. PPARalpha agonist prevented the apoptosis induced by glucose and fatty acid in neonatal cardiomyocytes. J Endocrinol Invest 2011;34:271-5. [Crossref] [PubMed]
- XiaoTian L, QiNan W, XiaGuang G, et al. Exenatide Activates the APPL1-AMPK-PPARα Axis to Prevent Diabetic Cardiomyocyte Apoptosis. J Diabetes Res 2016;2016:4219735. [PubMed]
- Bose AK, Mocanu MM, Carr RD, et al. Glucagon-like peptide 1 can directly protect the heart against ischemia/reperfusion injury. Diabetes 2005;54:146-51. [Crossref] [PubMed]
- Kim Chung le T, Hosaka T, Yoshida M, et al. Exendin-4, a GLP-1 receptor agonist, directly induces adiponectin expression through protein kinase A pathway and prevents inflammatory adipokine expression. Biochem Biophys Res Commun 2009;390:613-8. [Crossref] [PubMed]
- Lee YS, Shin S, Shigihara T, et al. Glucagon-like peptide-1 gene therapy in obese diabetic mice results in long-term cure of diabetes by improving insulin sensitivity and reducing hepatic gluconeogenesis. Diabetes 2007;56:1671-9. [Crossref] [PubMed]
- Samson SL, Gonzalez EV, Yechoor V, et al. Gene therapy for diabetes: metabolic effects of helper-dependent adenoviral exendin 4 expression in a diet-induced obesity mouse model. Mol Ther 2008;16:1805-12. [Crossref] [PubMed]