End-expiratory occlusion test predicts fluid responsiveness in cardiac surgical patients in the operating theatre
Introduction
Predicting fluid responsiveness is important in perioperative management and shock resuscitation (1,2). Functional hemodynamic assessment depending on heart-lung interactions has made great progress in recent years (3). Respiratory variation in stroke volume (SV) or other proxy indicators can predict fluid responsiveness in patients receiving mechanical ventilation (4,5). However, all the dynamic indicators pertinent to heart-lung interactions have limitations and can be used only under strict conditions.
The test of end-respiratory occlusion (EEO) was first reported by Monnet et al. in 2009 (6). By temporally stopping the inspiratory increase in intrathoracic pressure, EEO may allow for an increase in venous return, which further increases the left ventricular SV (6,7). Interestingly, the hemodynamic response to an EEO maneuver can predict fluid responsiveness in mechanically ventilated patients.
In published studies, the effects of EEO on SV have been monitored using either pulse contour analysis devices or transthoracic echocardiography (7-9). Most of these studies were conducted in an intensive care unit (ICU) (9-11). The accuracy of EEO in predicting preload responsiveness in the operation theater has been evaluated by only two studies, which have yielded conflicting conclusions (7,12). Using esophageal Doppler, Guinot et al. found that changes in SV induced by the EEO maneuver, did not accurately predict fluid responsiveness during surgery (12). On the contrary, Biais et al. demonstrated that an increase in pulse contour analysis SV induced by EEO accurately predicted fluid responsiveness in patients receiving low tidal volume mechanical ventilation in the operation room (7).
Transesophageal echocardiography (TEE) is now routinely used during cardiac surgical procedures for monitoring cardiac function non-invasively. The aim of the study was to evaluate whether the change in SV induced by the EEO maneuver under TEE monitoring could reliably predict fluid responsiveness in cardiac surgery patients in the operating theatre.
Methods
This study was approved by the Ethical Committee of Zhongshan Hospital affiliated to Fudan University (No. B2018-033R), and informed consent was obtained from all study participants. This trial has been registered at clinicaltrials.gov as NCT03485833.
Patient selection
Patients receiving elective coronary artery bypass grafting between March and July of 2018 in the cardiac surgical operating theatre of Zhongshan Hospital, Fudan University, were screened for enrollment in the study. The exclusion criteria included patients younger than 18 years old; patients with cardiac arrhythmia (e.g., atrial fibrillation or frequent premature beats); patients with moderate to severe valve disease (e.g., valve stenosis and/or regurgitation); patients with intracardiac shunt; patients with right heart dysfunction (tricuspid annular plane systolic excursion <16 mm, on the basis of TTE); patients with a left ventricular ejection fraction less than 30% before surgery; patients having contraindications for TEE probe insertion; and patients with poor cardiac echogenicity. Poor cardiac echogenicity was defined as an inability to align the Doppler beam at the left ventricular outflow tract (LVOT) and to obtain a reliable measurement of the velocity time integral (VTI), the key variable in the study, during the procedure. Patients were also excluded if the target-controlled infusion (TCI) of propofol or the doses of vasopressors or inotropes were modified to treat any unstable hemodynamic state during the procedure.
All patients were monitored with a five-lead electrocardiogram, pulse oximetry and non-invasive arterial pressure measurement. After insertion of an 8Fr dual-lumen central venous catheter through the right internal jugular vein and a radial artery catheter, all enrolled patients were induced with propofol TCI (target effect site concentration 2.5–3.0 µg/mL), sufentanil (0.3–0.5 µg/kg) and rocuronium (0.8 mg/kg), and then intubated. The absence of spontaneous breath was confirmed by a supervisor according to the anesthesia ventilator waveform and parameters. Ventilation was then performed in volume-controlled mode (Primus IE, Dräger Medical, Lübeck, Germany). The ventilatory parameters were adjusted as follows: tidal volume (Vt): 8 mL/kg predicted body weight, respiratory rate: 10 breaths per minute, inspiratory to expiratory ratio: 1:2, positive end expiratory pressure: 5 cmH2O and FiO2: 50%. Maintenance of anesthesia was achieved with propofol TCI (target effect site concentration 2 µg/mL). Administration of norepinephrine and/or dobutamine was considered according to the hemodynamic state. TEE probe insertion was routinely performed after the induction of anesthesia. The following baseline data were recorded for each patient: age (years), height (cm), weight (kg), gender, diagnosis, type of cardiac surgery, European system for cardiac operative risk evaluation (EuroSCORE), vasoactive drug infusion dose and preoperative echocardiographic parameters.
Measurements
The measured hemodynamic variables included heart rate (HR) (beats/minute), central venous pressure (CVP) (mmHg), mean arterial pressure (MAP) (mmHg). Stroke volume variation (SVV) was obtained from a FloTrac/Vigileo (Edwards Lifesciences, Irvine, CA, USA) continuous hemodynamic monitoring system. The pressure transducers were consistently adjusted to the level of the patient’s right atrium. The recorded ventilator settings included Vt, plateau pressure, peak pressure and exhaled carbon dioxide (CO2).
TEE measurements were performed by the same operator (KF Guo, who holds a certification in ultrasound evaluation, has 20-year experience in anesthesiology, and was blinded to the hemodynamic variables collected by other investigators (Y Yu and JY Hou). The probe was positioned to obtain a deep transgastric long axis view to acquire the optimum signal for velocity measurements in LVOT. A Philips IE33 ultrasound device (Philips Healthcare, Hamburg, Germany) was used to determine the VTI, the peak velocity (Vmax) and the mean velocity. The VTI was measured from the hand-drawn contours, and measurements were analyzed offline by investigators blinded to the patients’ response to fluid challenge. All measurements were averaged on three consecutive cardiac cycles.
The intra-observer variability and inter-observer variability of VTI measurement were investigated in 9 patients. The coefficient of variation (CV) was 1.6% for intra-observer variability and 1.8% for inter-observer variability. The least significant change (LSC) was calculated as described previously (13). The coefficient of error was calculated as the coefficient of variation divided by √n (n = number of replicates of measurements in each patient). The LSC was calculated as: Coefficient of error×1:96×√2.The LSC was 3.2% for VTI. Bland-Altman analysis showed good concordance between estimation of ΔVTI-EEO by the two investigators, with a mean bias of −0.008 and limits of agreement between −0.035 and 0.019. The reliability of the measurements was also analyzed with intraclass correlation coefficients (ICCs) assessing intra-observer and inter-observer correlation.
Study design
Measurements were taken from patients in the supine position after induction of anesthesia, before skin incision and after hemodynamic stabilization, which was defined as a change in MAP less than 10% during 5 minutes. TEE examinations and the collection of hemodynamic data were performed at baseline (baseline1), 20 seconds EEO maneuver, 1 min after the end of the EEO maneuver when the hemodynamic status returned to the initial baseline (baseline2), and after a 6 mL 0.9% saline per kg infusion in 10 min (fluid challenge) (Figure 1). Patients were classified as “fluid responders” if there was an increase in VTI ≥15% after the fluid challenge, and the remaining patients were classified as “fluid nonresponders”. During the procedure, the ventilatory settings and other treatments were kept constant.
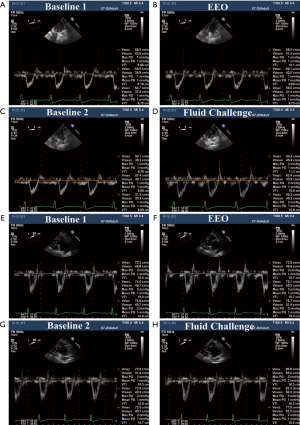
Statistical analysis
The normality of data was assessed with a Kolmogorov-Smirnov test. All continuous variables were normally distributed except the doses of norepinephrine and dobutamine. The normally distributed data were summarized as the mean ± SD. The non-normal data were reported as the medians (25–75% interquartile range) and compared between the groups using the Wilcoxn rank sum test. Variables before and after fluid challenge were compared with a paired Student’s t-test. Receiver operating characteristic curves (with 95% CI) were generated for the following variables: (I) percentage change in VTI induced by a 20-second EEO maneuver (ΔVTI-EEO); (II) percentage change in Vmax induced by a 20-second EEO maneuver (ΔVmax-EEO); (III) percentage change in pulse pressure (PP) induced by a 20-second EEO maneuver (ΔPP-EEO); (IV) baseline SVV; and (V) baseline CVP. AUC of ROC curves were compared with DeLong’s test. Differences with P<0.05 were considered statistically significant. Statistical analysis was performed in SPSS Statistics 21 (IBM, Montauk, VA, USA) and R-3.4.3. The reproducibility of VTI was assessed with Bland-Altman analysis. Statistical analysis was performed with MedCalc 11.6.0 software (MedCalc, Mariakerke, Belgium).
Results
During the study period (March 2018 to July 2018), 101 patients underwent coronary artery bypass grafting and met all the inclusion criteria. Eighteen (18.8%) patients were excluded, owing to poor echogenicity, and 8 (7.9%) patients were excluded because of changes in norepinephrine doses to treat hypotension during the study period. Seventy-five patients were included in the final analysis. Among them, 83% (n=62) were male. All patients were in sinus rhythm. The mitral and/or aortic valve regurgitation grades were less than mild. The baseline patient characteristics and clinical data are shown in Table 1.
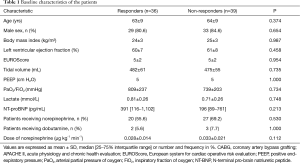
Full table
Hemodynamic and TEE data in responders and non-responders at all study times (baseline1, EEO, baseline2 and after fluid challenge) are reported in Table 2. Fluid challenge significantly increased VTI in LVOT by more than 15% in 36 (48%) patients (responders). The remaining 39 (52%) patients did not exhibit a significant change in VTI (non-responders). At baseline 1, MAP and PP were significantly lower (72±11 vs. 78±15 mmHg, P<0.05; 46±11 vs. 53±13 mmHg, P<0.05, respectively), and the SVV was significantly higher (16%±7% vs. 10%±5%, P<0.05) in responders than in non-responders. The heart rate and CVP were similar in both groups (65±12 vs. 61±9 beats min-1, P>0.05; 7±3 vs. 7±4 mmHg, P>0.05, respectively). TEE measurements indicated that both VTI and Vmax were lower in responders than in non-responders (12±3 vs. 14±3 cm, P<0.05; 68±13 vs. 72±15 cm second-1, P<0.05, respectively).
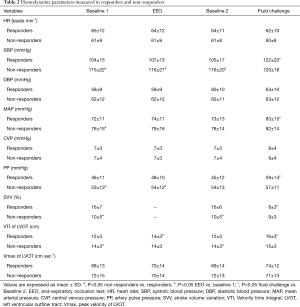
Full table
Before fluid challenge, the EEO maneuver increased VTI to a significantly greater extent in responders than in non-responders, whereas Vmax was not significantly affected. All other hemodynamic parameters remained stable during the EEO test (Table 2).
Fluid responsiveness was predicted by an increase of VTI greater than or equal to 5% during the EEOT (ΔVTI-EEO) with a sensitivity of 81% and a specificity of 93% (Table 3). The positive predictive and negative predictive values were 0.91 and 0.84, respectively. The AUC of ΔVTI-EEO to predict fluid responsiveness was significantly higher [0.90 (0.83–0.97)] than those for CVP (Z=4.93, P<0.01), SVV (Z=2.25, P=0.02), ΔPP-EEO (Z=2.64, P=0.01), and ΔVmax-EEO (Z=2.60, P=0.01) (Figure 2).
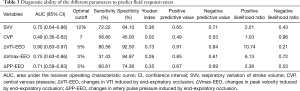
Full table
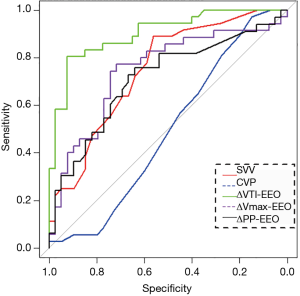
We included another nine patients to investigate the intraobserver and interobserver variabilities. We found good concordance between estimation of ΔVTI-EEO by the two investigators, with a mean bias of -0.008 and limits of agreement between −0.035 and 0.019. The reliability of the measurements was also analyzed with intraclass correlation coefficients to assess intra-observer and inter-observer correlations. The intraclass correlation coefficients of intraobserver and interobserver reliability were 0.986 (0.938–0.997) and 0.992 (0.964–0.998), respectively.
Discussion
This study demonstrated that an increase in VTI induced by EEO accurately predicted fluid responsiveness in mechanically ventilated cardiac surgery patients in the operating theatre. Moreover, the VTI was superior to CVP, SVV, ΔPP-EEO, and ΔVmax-EEO for this purpose.
Although it has been reported that the EEO test can accurately predict fluid responsiveness in critically ill patients, including patients with cardiac arrhythmias and spontaneous breathing (6,10,11,14), controversy remains regarding this maneuver. Predicting fluid responsiveness with the EEO test in the operating theatre seems more applicable and convenient, because patients are intubated and under influence of full sedation and muscle relaxation. Two studies have evaluated the accuracy of the EEO test in the operating theatre. Guinot et al. have reported that changes in SV measured by esophageal Doppler during an EEO maneuver cannot not predict fluid responsiveness (12). However, the investigators did not measure the changes in aortic diameter that physiologically accompany the changes in arterial blood pressure (15), thus potentially providing a partial explanation for the negative results of the study. In another study, Biais et al. have reported that changes in SV induced by EEO can predict fluid responsiveness (7). In their study, pulse contour analysis was used to estimate SV (7). However, they included a small number of highly selected patients. Patients with cardiac dysfunction and use of vasopressors or inotropes before and during anesthesia were excluded from that study. To our knowledge, the present study is the first to evaluate the EEO test in predicting fluid responsiveness in cardiac surgical patients using TEE monitoring in the operating theatre.
Pulsed-wave Doppler provides an estimation of SV on the basis of calculation of the VTI and the diameter of the LVOT. Because the area of the LVOT does not change over time, changes in the VTI allow the changes in SV to be assessed (16-18). In this study, we found that an increase in VTI greater than 5% during an EEO test predicted fluid responsiveness with a receiver operating characteristic area of 0.90 as well as satisfactory positive and negative predictive values. This finding was consistent with those from previous studies using calibrated or uncalibrated pulse contour analysis to evaluate the utility of the EEO test to predict fluid responsiveness (6,7,11). Weber et al. have revealed that the esophageal Doppler peak velocity is predictive of fluid responsiveness (19). However, ΔVmax induced by EEO was found to be poorly predictive of fluid responsiveness in this study. We presumed that the changes in PP induced by EEO might be able to reflect the changes in SV induced by EEO. Nevertheless, changes in PP during EEO test were not able to predict fluid responsiveness in this study. Since we measured PP at a peripheral level and not in the aorta, we cannot exclude that the pulse wave amplification phenomenon at the peripheral level might be responsible for this result (20,21).
With the rapid development of point-of-care ultrasound in recent years, non-invasive ultrasound appears to be an ideal bedside tool for fluid status assessment (22,23). Two studies have assessed the hemodynamic changes induced by EEO, by using transthoracic echocardiography monitoring (8,9). Jozwiak et al. have confirmed that an increase in VTI greater than or equal to 5% during an EEO test can reliably predict fluid responsiveness, with a sensitivity of 93% and a specificity of 100%. When combining EEO and an end-inspiratory occlusion maneuver, a change in VTI ≥13% can predict fluid responsiveness, with a sensitivity of 93% and a specificity of 93% (8). Georges et al. have revealed that a 9% increase in VTI induced by the 12-second EEO test can predict fluid responsiveness, with a sensitivity of 89% and a specificity of 95% (9). TEE is now routinely used during cardiac surgery, thus allowing for continuous measurement of SV. After the depth and scanning angle of the probe is fixed, the echocardiographic views can also be well fixed, a crucial aspect for evaluating the hemodynamic changes during both EEO and fluid challenge. Even though the cut-off value (5%) was close to the intra-observer variability for the VTI measurement (8,24), we found that the VTI change during the EEO was a reliable predictor of fluid responsiveness.
Our study has several limitations. First, we enrolled mechanically ventilated patients with only coronary artery disease. Whether the conclusions can be extrapolated to other populations remains uncertain. Although we did not calculate the sample size required in this study, the number of enrolled patients was larger than those in similar studies evaluating the prediction ability of EEO (7-9,12). Second, we performed an EEO during 20 seconds instead of 15 or 12 seconds, as previously described (6,9). It was considered that extended EEO tests were feasible and convenient to perform in the operating theatre and might possibly lead to higher magnitude changes in SV. Third, we did not measure the SV on the basis of calibrated pulse contour analysis. The response to the EEO has already been assessed by using pulse contour analysis-derived cardiac output in previous studies (6,7). Although the absolute value of SV measured by TEE might not be equal to that measured by the thermodilution method (25,26), TEE is considered to detect rapid changes in SV induced by EEO.
Conclusions
This study showed that an increase in VTI of at least 5% induced by a 20-second EEO can reliably predict fluid responsiveness in cardiac surgical patients in the operating theatre, whereas the EEO-induced changes in Vmax and PP cannot. EEO tests monitored by TEE can therefore be regarded as a method to predict fluid responsiveness during cardiac surgery.
Acknowledgments
We thank Jian-Feng Luo from the Department of Biostatistics, School of Public Health, Fudan University for his kind support regarding the statistical analysis. We also deeply thank all the cardiac surgeons from the Department of Cardiac Surgery, Zhongshan Hospital, Fudan University for their patience in the operating theatre.
Funding: This article was supported by grants from the research funds of Zhongshan Hospital (2018ZSQN53 and 2019ZSQN13).
Footnote
Conflicts of Interest: The authors have no conflicts of interest to declare.
Ethical Statement: This study was approved by the Ethical Committee of Zhongshan Hospital affiliated to Fudan University (No. B2018-033R), and informed consent was obtained from all study participants. This trial has been registered at clinicaltrials.gov as NCT03485833. Authors are accountable for all aspects of the work (if applied, including full data access, integrity of the data and the accuracy of the data analysis) in ensuring that questions related to the accuracy or integrity of any part of the work are appropriately investigated and resolved.
References
- Monnet X, Marik PE, Teboul JL. Prediction of fluid responsiveness: an update. Ann Intensive Care 2016;6:111. [Crossref] [PubMed]
- Byrne L, Van Haren F. Fluid resuscitation in human sepsis: Time to rewrite history? Ann Intensive Care 2017;7:4. [Crossref] [PubMed]
- Alvarado Sánchez JI, Amaya Zuniga WF, Monge Garcia MI. Predictors to Intravenous Fluid Responsiveness. J Intensive Care Med 2018;33:227-40. [Crossref] [PubMed]
- Morparia KG, Reddy SK, Olivieri LJ, et al. Respiratory variation in peak aortic velocity accurately predicts fluid responsiveness in children undergoing neurosurgery under general anesthesia. J Clin Monit Comput 2018;32:221-6. [Crossref] [PubMed]
- Ma GG, Hao GW, Yang XM, et al. Internal jugular vein variability predicts fluid responsiveness in cardiac surgical patients with mechanical ventilation. Ann Intensive Care 2018;8:6. [Crossref] [PubMed]
- Monnet X, Osman D, Ridel C, et al. Predicting volume responsiveness by using the end-expiratory occlusion in mechanically ventilated intensive care unit patients. Crit Care Med 2009;37:951-6. [Crossref] [PubMed]
- Biais M, Larghi M, Henriot J, et al. End-Expiratory Occlusion Test Predicts Fluid Responsiveness in Patients With Protective Ventilation in the Operating Room. Anesth Analg 2017;125:1889-95. [Crossref] [PubMed]
- Jozwiak M, Depret F, Teboul JL, et al. Predicting Fluid Responsiveness in Critically Ill Patients by Using Combined End-Expiratory and End-Inspiratory Occlusions With Echocardiography. Crit Care Med 2017;45:e1131-8. [Crossref] [PubMed]
- Georges D, de Courson H, Lanchon R, et al. End-expiratory occlusion maneuver to predict fluid responsiveness in the intensive care unit: an echocardiographic study. Crit Care 2018;22:32. [Crossref] [PubMed]
- Silva S, Jozwiak M, Teboul JL, et al. End-expiratory occlusion test predicts preload responsiveness independently of positive end-expiratory pressure during acute respiratory distress syndrome. Crit Care Med 2013;41:1692-701. [Crossref] [PubMed]
- Monnet X, Bleibtreu A, Ferre A, et al. Passive leg-raising and end-expiratory occlusion tests perform better than pulse pressure variation in patients with low respiratory system compliance. Crit Care Med 2012;40:152-7. [Crossref] [PubMed]
- Guinot PG, Godart J, de Broca B, et al. End-expiratory occlusion manoeuvre does not accurately predict fluid responsiveness in the operating theatre. Br J Anaesth 2014;112:1050-4. [Crossref] [PubMed]
- Monnet X, Persichini R, Ktari M, et al. Precision of the transpulmonary thermodilution measurements. Crit Care 2011;15:R204. [Crossref] [PubMed]
- Monnet X, Dres M, Ferre A, et al. Prediction of fluid responsiveness by a continuous non-invasive assessment of arterial pressure in critically ill patients: comparison with four other dynamic indices. Br J Anaesth 2012;109:330-8. [Crossref] [PubMed]
- Monnet X, Teboul JL. End-expiratory occlusion test: please use the appropriate tools! Br J Anaesth 2015;114:166-7. [Crossref] [PubMed]
- Cecconi M, De Backer D, Antonelli M, et al. Consensus on circulatory shock and hemodynamic monitoring. Task force of the European Society of Intensive Care Med. Intensive Care Med 2014;40:1795-815. [Crossref] [PubMed]
- Miller A, Mandeville J. Predicting and measuring fluid responsiveness with echocardiography. Echo Res Pract 2016;3:G1-12. [Crossref] [PubMed]
- Lazzeri C, Peris A. Fluid responsiveness raises many questions-echocardiography may be the answer. J Thorac Dis 2017;9:946-9. [Crossref] [PubMed]
- Weber T, Wagner T, Neumann K, et al. Low predictability of three different noninvasive methods to determine fluid responsiveness in critically ill children. Pediatr Crit Care Med 2015;16:e89-94. [Crossref] [PubMed]
- De la Puente-Diaz de Leon V, de Jesus Jaramillo-Rocha V, Teboul JL, et al. Changes in Radial Artery Pulse Pressure During a Fluid Challenge Cannot Assess Fluid Responsiveness in Patients With Septic Shock. J Intensive Care Med 2017.885066617732291. [Epub ahead of print]. [PubMed]
- Monnet X, Letierce A, Hamzaoui O, et al. Arterial pressure allows monitoring the changes in cardiac output induced by volume expansion but not by norepinephrine. Crit Care Med 2011;39:1394-9. [Crossref] [PubMed]
- Trifi A, Abdellatif S, Daly F, et al. Ultrasound stroke volume variation induced by passive leg raising and fluid responsiveness: An observational cohort study. Med Intensiva 2019;43:10-7. [Crossref] [PubMed]
- Álvarez-Fernández JA, Nunez-Reiz A. Clinical ultrasound in the ICU: changing a medical paradigm. Med Intensiva 2016;40:246-9. [PubMed]
- Muller L, Toumi M, Bousquet PJ, et al. An increase in aortic blood flow after an infusion of 100 ml colloid over 1 minute can predict fluid responsiveness: the mini-fluid challenge study. Anesthesiology 2011;115:541-7. [Crossref] [PubMed]
- Mantovani MM, Fantoni DT, Gimenes AM, et al. Clinical monitoring of cardiac output assessed by transoesophageal echocardiography in anaesthetised dogs: a comparison with the thermodilution technique. BMC Vet Res 2017;13:325. [Crossref] [PubMed]
- Muñoz L, Velandia A, Reyes LE, et al. Evaluation of concordance among three cardiac output measurement techniques in adult patients during cardiovascular surgery postoperative care. Med Intensiva 2017;41:539-45. [Crossref] [PubMed]