Effects of GLP-1 receptor analogue liraglutide and DPP-4 inhibitor vildagliptin on the bone metabolism in ApoE−/− mice
Introduction
There is a close relationship among osteoporosis, atherosclerosis and hyperlipidemia (1). Dyslipidemia has been proven to be a major risk factor of osteoporosis related fracture and atherosclerotic diseases (2-4). Patients with atherosclerosis may experience the loss of bone mass, which may eventually lead to the osteoporotic fractures. It has been reported that hyperlipidemia can induce osteoporosis in animal models (5). However, diabetic osteoporosis (DOP) has not been reported in diabetic mice and rats.
ApoE−/− mice are commonly used in the studies on atherosclerosis (6,7) because ApoE gene defect may cause the disorder of lipid metabolism. Studies have revealed that either normal or high fat diet may induce severe hyperlipidemia in ApoE−/− mice (6,7). Thus, ApoE−/− mice are also used in studies on the osteoporosis or bone loss since the close relationship between hyperlipidemia and osteoporosis (8).
ApoE−/− mice with high fat diet not only develop significantly elevated fasting blood glucose (FBG), cholesterol, triglyceride (TG), free fatty acids (FFA) and fasting insulin, but also have decreased insulin sensitivity and insulin resistance which can cause islet β cell dysfunction, leading to the diabetes.
There is evidence showing that the development of DOP is closely related to the AGEs and receptors for advanced glycation end products (RAGE) (9). Increased AGEs may decrease the number and activity of osteoblasts and then reduce bone formation. Glucagon-like peptide1 receptor analogues (GLP-1RA) liraglutide and dipeptidyl peptidase-4 inhibitors (DPP-4i) vildagliptin are two widely used glucose lowering drugs and can also reduce AGEs. In addition, liraglutide can induce the insulin release and reduce AGEs accumulation. GLP-1 receptors are expressed on the osteoblasts (10) and thus GLP-1 may be beneficial for the bone metabolism (11) via stimulating the proliferation and differentiation of osteoblasts. Vildagliptin can prevent the rapid degradation of GLP-1 through inhibiting DPP-4. Currently, the effects of liraglutide and vildagliptin on bone metabolism are still poorly understood.
Some meta-analyses (12-14) have revealed a strong association between diabetes (both type 1 and type 2 diabetes mellitus) and increased risk for hip fractures in men and women regardless races. Besides diabetes, prediabetes and obesity-related insulin resistance may also adversely affect the bone microstructure (15) through a variety of mechanisms, resulting in the osteopenia and osteoporosis (16).
Currently, the concentration of AGEs used for intraperitoneal injection in animal models is still unclear. Our pilot study showed the mortality was very high when the concentration of AGEs was 30 mg/kg/d, and intraperitoneal AGEs at 25 mg/kg/d for 10 weeks could increase the serum concentration of AGEs by three times.
In the present study, ApoE−/− mice with euglycemia were intraperitoneally injected with AGEs to induce chronic diabetic complications, and the effects of liraglutide and vildagliptin on the bone metabolism were further investigated.
Methods
Animals and groups
Ninety male ApoE−/− mice aged 10 weeks were purchased from Beijing Vitalriver Co., Ltd. (Beijing, China) and allowed to accommodate to the environment for 2 weeks. These mice were housed in the Experimental Animal Center of Shanghai East Hospital and given ad libitum access to the food and water in a specific pathogen free (SPF) environment with 12-h light/dark cycle. Two weeks later, animals were fed with a high-fat diet (Slaccas, Shanghai, China) and randomly divided into two groups, control group (n=45, Group C) and AGEs group (AGEs, i.p. 25 mg/kg/d, n=45, Group A). Mice in each group were further divided into 3 subgroups: vildagliptin subgroups [35 mg/kg/d, n=15, Group CV (control + vildagliptin) and Group AV (AGE + vildagliptin)], liraglutide subgroups [0.4 mg/kg/d, n=15, Group CL (control + liraglutide) and Group AL (AGEs + liraglutide)] and control groups (n=15, Group CC (control + control) and Group AC (AGEs + control)]. The body weight, random blood glucose and individual food intake were monitored once weekly. After 10 weeks, these mice were sacrificed after intraperitoneal anesthesia, blood was collected, and the femur was rapidly harvested. The levels of biochemical markers related to bone metabolism were detected, and the femoral morphology was examined by micro-CT.
Preparation of AGEs
AGEs-bovine serum albumin (AGEs-BSA) was prepared according to previously reported (17). BSA (50 g/L, Sigma-Aldrich, St Louis, MO, USA), D-glucose (500 mM, Sigma-Aldrich, St Louis, MO, USA), penicillin (100 U/mL, GIBCO, Invitrogen Inc., Carlsbad, CA, USA) and streptomycin (0.1 mg/mL, GIBCO, Invitrogen Inc., Carlsbad, CA, USA) were dissolved in phosphate buffer saline (PBS; 0.2 M, pH 7.4) followed by incubation overnight at room temperature. After sterilization through a 0.22-µm filter, the solution was incubated at 37 °C in dark for 90 days, followed by dialysis against the 0.1 M phosphate buffer for 24 h to remove unincorporated glucose. The successfully prepared AGEs were confirmed by fluorescence spectrophotometry with the slit at 2.5 nm, voltage at 700 mV, excitation wavelength of 370 nm and emission wavelength of 440 nm. The solution was then lyophilized.
Biochemical bone index
The serum levels of AGEs, total cholesterol (TC), and TG were measured using commercially available ELISA kits (Bio-Swamp Co., Ltd., Beijing, China), according to the manufacturer’s instructions. The serum levels of osteocalcin (OC), N-terminal propeptide of type I procollagen (PINP), parathyroid hormone (PTH), human cross-linked carboxy-terminal telopeptide of type I collagen (CTX), and tartrate-resistant acid phosphatase (TRACP) were measured using corresponding ELISA kits (Bio-Swamp Co., Ltd., Beijing, China) according to manufacturer’s instructions.
Detection of RAGE-mRNA expression by real-time PCR
Total RNA was extracted from the femoral tissues using the Trizol reagent (Invitrogen Inc., Carlsbad, CA, USA). Purified RNA was used as a template for the first-strand cDNA synthesis using the Primer Script RT reagent kit (Takara Bio, Otsu, Japan). Real-time PCR was performed with a SYBR Green PCR kit (Takara Bio, Otsu, Japan) on the ABI 7500 real-time PCR system (Applied Biosystems, Foster City, CA, USA) according to the manufacturer’s instructions. mRNA expression of RAGE was determined using the Ct method and normalized to the GADPH mRNA expression. Primers (Sangon Biotech, Shanghai, China) for RAGE were as follows: forward 5'-GAA GGC TCT GTG GGT GAG TC-3' and reverse 5'-ATT CAG CTC TGC ACG TTC CT-3'. Primers (Sangon Biotech, Shanghai, China) for GADPH were as follows: forward 5'-CCT GAC CAC CAA CTG CTT AGC-3' and reverse 5'-CCA GTG AGC TTC CCG TCT AGC-3'.
Detection of RAGE protein expression by Western blotting
Total proteins were extracted from the femoral tissues with RIPA lysis buffer. Equal amounts of protein were separated by 10% or 12% SDS-PAGE gels and then transferred onto polyvinylidene fluoride (PVDF) membrane (Millipore, Massachusetts, USA). Non-specific binding was blocked with 5% non-fat milk for 1 h. The membranes were incubated with primary antibodies against RAGE (1:500; Abcam, Cambridge, MA, USA) and GAPDH (1:2,000; Boster Bioengineering Co., Wuhan, China) overnight at 4 °C and then with secondary antibody (HRP-conjugated IgG; 1:2,000; Jackson Immuno Research, West Grove, PA, USA) for 1 h. Visualization was done with ECL chemiluminescence system, and the protein expression was analyzed using the Gel-Pro Analyzer 4 software and normalized to the protein expression of GAPDH.
Examination of femoral metrology by Micro CT
The femurs were fixed in 10% Millonig’s formalin and then transferred to 70% ethanol. The SkyScan-1176 micro-computed tomography (µCT) system (Bruker micro CT, Belgium) was used for the bone microstructure examination. Scans were performed using 8.96-µm voxel size, 50 KV, 350 µA and 0.45 degrees rotation. For trabecular bone, micro-CT was performed on a 1-mm region of metaphyseal spongiosa in the distal femur. The regions located 0.5 mm above the growth plate (femur). For cortical bone, measurements were performed on a 0.5-mm region of mid-diaphysis of the femur. After 3D reconstruction, the CTan software was used for the bone analysis. The bone mineral density (BMD), bone volume/tissue volume (BV/TV), structure model index (SMI), trabecular thickness (Tb.Th), trabecular number (Tb.N), and trabecular separation/spacing (Tb.Sp) were determined for the trabecular bone. Cortical bone measurements included cortical bone mineral density (BMD), bone volume/tissue volume (BV/TV), cortical bone thickness (Ct.Th), and porosity of cortical bone (Po%[tot]) were measured.
Statistical analysis
Statistical analysis was performed with SPSS version 19.0, and data are expressed as mean ± standard deviation . The independent sample t-test was used for comparisons between two groups, and one way analysis of variance (ANOVA) was used for comparisons among groups, followed by post hoc least significant difference (LSD) test. A value of P<0.05 was considered statistically significant.
Results
Vildagliptin increases OC, while liraglutide reduces PTH
The OC was 1.50±2.42 ng/mL in the CV group, 0.42±0.14 ng/mL in the Group CL and 0.63±1.12 ng/mL in the Group CC (Figure 1). As compared to Group CC, the OC in the Group CV tended to increase although there was no marked difference (P=0.058). Compared with the Group CV, the OC significantly decreased in the Group CL (P<0.01) (Figure 1A). The PTH was 278.81±30.10 pg/mL in the Group CC, 283.71±54.64 pg/mL in the Group CV and 246.93±27.02 pg/mL in the Group CL. As compared to the CC and CV groups, the PTH significantly decreased in the CL group (P<0.05) (Figure 1C). There were no significant differences among three groups in the PINP, TRACP and CTX (Figure 1B,D,E).
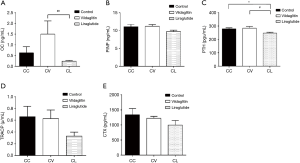
mRNA and protein expressions of RAGE remained unchanged in the femurs of CC, CV and CL groups
The RBG was 8.62±0.82 mmol/L in the CC group, 8.15±0.67 mmol/L in the CV group and 6.79±0.56 mmol/L in the CL group. Compared with the Group CC, the RBG significantly decreased in the Group CV (P<0.05), but markedly decreased in the Group CL (P<0.01). Compared with the Group CV, the RBG significantly decreased in the Group CL (P<0.01) (Figure 2A). In addition, the serum AGEs was 6.56±1.87 ng/mL in the Group CC and 5.79±1.75 ng/mL in the group CV, showing no significant difference between them. However, as compared to the Group CC, the AGEs in the Group CL declined significantly (4.81±1.00 ng/mL; P<0.01) (Figure 2B).
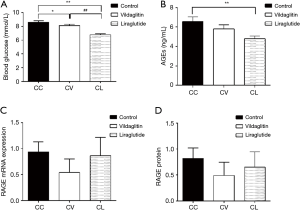
The mRNA and protein expressions of RAGE were detected by RT-PCR and Western blotting, respectively. There were no significant differences among three groups in the mRNA and protein expressions of RAGE (Figure 2C,D).
Bone biochemical indexes in different groups
The TRACP was 0.58±0.36 U/mL in the Group AC and 0.28±0.06 U/mL in the Group AL (Figure 3), showing marked difference between them (P<0.05) (Figure 3D). There were no significant differences among three groups in the OC, PINP, PTH and CTX (Figure 3A,B,C,E).
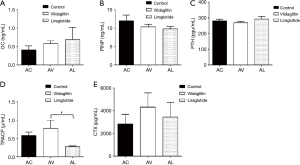
mRNA and protein expressions of RAGE in the femurs among AC, AV and AL groups
The RBG was 8.03±0.42 mmol/L in the Group AC, 8.41±0.48 mmol/L in the Group AV and 6.09±0.31 mmol/L in the Group AL. Compared with the Group AC and Group AV, the RBG declined significantly in the Group AL (P<0.01) (Figure 4A).
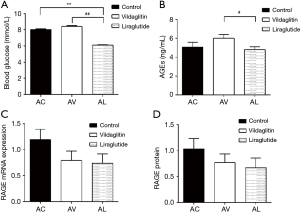
The serum AGEs was 5.05±1.95 ng/mL in the Group AC and 6.02±1.53 ng/mL in the Group AV, showing no significant difference between them. The AGEs in the Group AL was 4.80±1.14 ng/mL, which was markedly lower than in the Group AC (P<0.05) (Figure 4B).
The mRNA and protein expressions of RAGE in the femurs were comparable among AC, AV and AL groups (Figure 4C,D).
Effects of vildagliptin and liraglutide on the bone biochemical indexes in the AGEs treated ApoE−/−mice
The OC was 1.50±2.42 ng/mL in the Group CV and 0.58±0.30 ng/mL in the Group AV (Figure 5), showing significant difference between them (P<0.05) (Figure 5A). The PTH was 246.93±27.02 pg/mL in the Group CL, which was markedly lower than in the Group AL (292.08±63.62 pg/mL; P<0.01) (Figure 5C). The CTX in the Group CV (1,223.14±225.03 pg/mL) was significantly higher than in the Group AV (4,323.85±4,844.59 pg/mL; P<0.01) (Figure 5E). The CTX in the Group AL (3,439.15±4,498.26 pg/mL) increased significantly as compared to the Group CL (995.99±553.55 pg/mL; P<0.05) (Figure 5E). There were no significant differences in the PINP and TRACP between CC and AC groups, between CV and AV groups, and between CL and AL groups (Figure 5B,D).
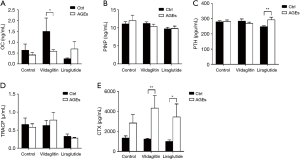
Effects of vildagliptin and liraglutide on the mRNA and protein expressions of RAGE in the femurs of AGEs treated ApoE−/− mice
Compared with the Group CC, RBG declined significantly in the Group AC (P<0.01); Compared with the Group CL, RBG declined significantly in the Group AL (P<0.01) (Figure 6A). The serum AGEs in the Group AC was markedly lower than in the Group CC (P<0.05); the AGEs increased in the Group AV as compared to the Group CC although there was no significant difference (Figure 6B).
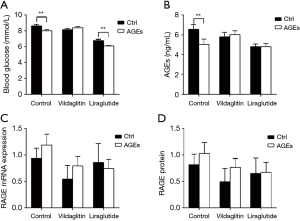
There were no marked differences in the mRNA and protein expressions of RAGE between CC and AC groups, between CV and AV groups and between CL and AL groups (Figure 6C,D).
There was no significant difference in the femoral morphology
The cancellous BMD was 0.19±0.02 g/cm3 in the Group AL, which was significantly lower than in the Group CL (P<0.05). The cortical BMD in the Group AV was 1.08±0.01 g/cm3, which was markedly higher than in the Group CV (P<0.05). Compared with the Group CV, the cortical Cr.Th (0.20±0.01 mm) increased dramatically in the Group AV (P<0.05). However, there were no significant differences in other femoral morphology indexes between CC and AC groups, between CV and AV groups, and between CL and AL groups (Figure 7).
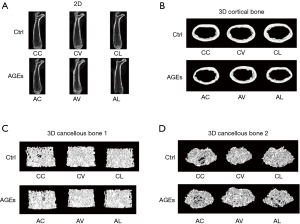
Discussion
Our results indicated AGEs could adversely affect the bone metabolism of ApoE−/− mice. Intraperitoneal AGEs reduced OC and elevated CTX. A large number of studies have proven that AGEs at a high concentration and AGE/RAGE axis imbalance are closely related to the occurrence and development of DOP. With the increases in the AGEs and RAGE, the apoptosis of mesenchymal stem cells increases and the tibial cancellous bone experiences massive loss (18). AGEs can also inhibit the proliferation, self-renewal and differentiation of osteoblasts in vitro (19). Moreover, the increases in the collagen crosslinking and bone matrix can increase the brittleness of collagenous fibers and accumulate microdamage of the bones, resulting in the degeneration of posterior yield stress and toughness of the bones. OC, a factor reflecting bone formation, is a small carboxylated protein synthesized by osteoblasts and osteocytes. Studies in mice (20) have shown that OC can promote insulin secretion and improve insulin sensitivity. It has been reported that OC receptors are expressed on both islet β-cells and intestinal epithelial cells. The binding of OC to its receptors may induce β-cell proliferation and GLP-1 release, leading to the insulin secretion. Undercarboxylated osteocalcin (ucOC) is another form of OC, has a low affinity to hydroxyapatite, and thus is more readily released into the circulation. ucOC is the hormonally active form of OC. Homozygous osteocalcin-deficient (Ocn−/−) mice are hyperglycemic and hypoinsulinemic, and have reduced pancreatic β-cell mass due to the decreased β-cell proliferation (21). In the obese mice, administration of ucOC can stimulate β-cell proliferation and insulin production, which may improve the glucose homeostasis and diet-induced obesity (22). This suggests that OC and/or ucOC may affect glucose metabolism. A recent clinical study suggests the skeleton may normalize the slightly increased HbA1c in the glucose-tolerant individuals with prediabetes by enhancing osteoblast and osteoclast activities (23). In addition, OC is also negatively related to HbA1c after adjusting all the other parameters related to HbA1c (24-26). This is consistent with our finding that administration of AGEs reduced OC.
The OC bioactivity can be induced by bone resorption, because the ability of OC to augment insulin release relies on the acidic bone microenvironment provided by enhanced osteoclastic activity (20,27,28). In our study, the CTX, an index reflecting bone resorption, increased significantly in the ApoE−/− mice treated with AGEs. Other studies (23) also reveal CTX has a positive association with HbA1c in women with normal glucose tolerance. This may prevent further aggravation of impaired glucose metabolism. To suppress the increase in blood glucose in case of prediabetes, the bone turnover begins to increase and so does CTX secretion, while OC/ucOC passively elevates to induce the release of more insulin to lower blood glucose. Thus, the effects of OC on glucose homeostasis are related to the bone resorption (27,29). Liu et al. (30) proposed that moderately enhanced CTX at baseline was protective against the deterioration of glucose metabolism. Thus, the bone is actually an endocrine organ which can regulate the insulin secretion and glucose homeostasis.
Liraglutide and vildagliptin are two common glucose-lowering drugs, all of which are incretins, but they exert effects via different ways. Liraglutide is a GLP-1 analogue with prolonged half life as compared to natural GLP-1. By activating GLP-1 receptor, liraglutide exerts hypoglycemic effect as natural GLP-1. DPP-4 is an enzyme for the GLP-1 degradation. Vildagliptin is a DPP-4 inhibitor that can inhibit the degradation of GLP-1 by inhibiting the DPP-4 enzyme, which increases the GLP-1 concentration. Liraglutide can promote insulin release, indirectly act on osteoblasts and stimulate proliferation and differentiation of osteoblasts. Our study showed liraglutide treatment could reduce the AGEs and decrease the PTH. PTH and calcitonin (CT) are two main hormones to maintain calcium homeostasis in vivo, and they are antagonistic and complementary to each other. The sustained PTH stimulation can enhance bone resorption. Intermittent application of low dose PTH can promote bone formation (31). Physiologically, CT is to enhance bone formation. It has been confirmed that the thyroid parafollicular cells, which can secrete CT, also have the expression of GLP-1 receptors. The binding of GLP-1 to GLP-1 receptors is able to enhance the secretion of CT. When the level of sclerostin decreases, the osteoclast formation is inhibited (11). Although the blood CT was not detected in the present study, theoretically, the blood CT in the ApoE−/− mice increases after treatment with liraglutide, the PTH reduces, and the bone resorption is inhibited. Our study indicates that liraglutide can improve bone metabolism by decreasing PTH.
A variety of studies have shown that GLP-1 receptor agonist may stimulate osteoblastogenesis (11) and inhibit osteoclastogenesis. Specifically, the osteoblastogenesis has been hypothesized to be related to the activation of Wnt/β-catenin pathway and/or increased OPG/RANKL ratio. In addition, the osteoclastogenesis inhibition has been suggested to be mediated by the reduction of sclerostin. Hypoglycemia can reduce the accumulation of AGEs and decrease the bone resorption. In brief, our study demonstrates that liraglutide can improve bone metabolism.
Vildagliptin is a DPP-4 inhibitor. Our results showed that vildagliptin treatment decreased AGEs to some extents, accompanied by a marked increase in the OC. This suggests that OC is negatively related to HbA1c. Thus, vildagliptin may facilitate the bone formation by reducing AGEs.
DPP-4 has many substrates. GLP-1 and GIP are the two main incretin substrates. GLP-2 and insulin-like growth factor 1 (IGF-1) are the two non-incretin substrates. Studies have shown that GIP receptor deletion (GIPR KO) mice have increased osteoclasts, thinned bone cortex and decreased BMD (32). It indicates that GIP is beneficial for the bone strength and quality. GLP-2 receptors are expressed on the osteoclasts. The binding of GLP-2 to its receptor may inhibit the bone resorption (33). IGF-1 receptors are expressed on the osteoclasts and osteoblasts. The binding of IGF-1 to its receptor may promote the proliferation of osteoblasts (33). In addition, IGF-1 can increase the OPG, reduce the RANKL expression, and thus inhibit the bone resorption. Meanwhile, IGF-1 is an important mediator in the interaction between PTH and bone. These findings indicate that DPP4-i can improve the bone metabolism. In our study, results showed vildagliptin was able to improve the bone metabolism. In future studies, we will detect some other indicators such as GIP, GLP-2, and IGF-1 to investigate whether vildagliptin can improve bone metabolism through these pathways.
However, a meta-analysis of 51 RCTs by Mamza et al. showed no association between fracture events and DPP-4 inhibitors (34). Population-based cohort studies also indicate the use of DPP-4 inhibitors have no influence on the fracture rate (35). Fu et al. (36) claimed that there was no statistically significant difference in the risk for fracture between each DPP-4is. Limited clinical data of DPP-4i indicate a neutral effect on the bone health and the risk for bone fractures (37,38). Similar results were also proven by a retrospective population-based cohort study (39). Thus, the role of DPP4-is in the risk for bone fracture remains unclear and controversial.
The mRNA and protein expressions of RAGE in the femur were also detected, and significant differences were not observed among groups. Ding et al. (40) found the bone intensity and BMD increased in the RAGE knockout mice, and the osteoclasts decreased as compared to the wild-type mice. Hamada et al. (41) examined the biomechanical properties of the femur and tibia by histomorphometry in the RAGE knockout rats, and their results showed the BMD increased, bone resorption parameters (osteoclast surface, the number of osteoclast/bone circumference) decreased significantly, and the osteoclast function of RAGE defect rats also decreased. Zhou et al. (42) induced the differentiation of osteoclasts in vitro and found that their bone absorption decreased significantly as compared to the normal osteoclasts. Meanwhile, the RAGE mRNA expression was detected in the osteoclast-like cells in vitro. Results from in vivo experiments further confirmed above findings: the bone mechanical strength and bone mass increased in the RAGE knockout mice as compared to the wild-type mice; and the bone resorption markers in the serum and the number of osteoclasts reduced as compared to the wild-type mice (40).
Stolzing et al. (18) showed AGEs were able to promote the apoptosis of bone marrow mesenchymal stem cells. AGEs-RAGE system may not only deplete bone marrow mesenchymal stem cells, but also compromise their potential differentiation to osteoblasts. Studies have shown that the body mass loss increases after ovariectomy, which is the most obvious in the area rich in cancellous bone, and the bone loss at the distal femoral metaphyseal is 3 times that of the total femur (43). Raskin et al. (44) used bone densometer to measure the acquired changes in the femur and tibia of ovariectomized rats and they found evident bone mass loss at the whole femur and distal femur. These results indicate the whole femur and the distal femur are suitable for the assessment of osteoporosis.
The femoral morphology was also examined by micro-CT in the present study. Our results showed vildagliptin and liraglutide failed to improve the bone metrology, and no significant change was observed in BMD.
In conclusion, our study shows AGEs may adversely affect the bone metabolism. The elevated AGEs may exacerbate the osteogenesis and increase the bone resorption, but vildagliptin and liraglutide may improve the bone metabolism. Our findings shed lights on the further exploration of mechanisms underlying the bone changes associated with dysglycemia, as well as on the design and use of diabetes medications in patients with concomitant osteopenia and osteoporosis.
Acknowledgments
Funding: This work was supported by the Key Specialty Construction Project of Pudong Health and Family Planning Commission of Shanghai (PWZzk2017-12).
Footnote
Conflicts of Interest: The authors have no conflicts of interest to declare.
Ethical Statement: All the procedures were done according to the Guideline for Experimental Animal Use and Care as well as the Declaration of Helsinki. All authors are accountable for all aspects of the work, including full data access, integrity of the data and the accuracy of the data analysis.
References
- Stojanovic OI, Lazovic M, Lazovic M, et al. Association between atherosclerosis and osteoporosis, the role of vitamin D. Arch Med Sci 2011;7:179-88. [Crossref] [PubMed]
- Parhami F, Garfinkel A, Demer LL. Role of lipids in osteoporosis. Arterioscler Thromb Vasc Biol 2000;20:2346-8. [Crossref] [PubMed]
- Wu LY, Yang TC, Kuo SW, et al. Correlation between bone mineral density and plasma lipids in Taiwan. Endocr Res 2003;29:317-25. [Crossref] [PubMed]
- Parhami F, Tintut Y, Beamer WG, et al. Atherogenic high-fat diet reduces bone mineralization in mice. J Bone Miner Res 2001;16:182-8. [Crossref] [PubMed]
- Mandal CC. High Cholesterol Deteriorates Bone Health: New Insights into Molecular Mechanisms. Front Endocrinol (Lausanne) 2015;6:165. [Crossref] [PubMed]
- Plump AS, Smith JD, Hayek T, et al. Severe hypercholesterolemia and atherosclerosis in apolipoprotein E-deficient mice created by homologous recombination in ES cells. Cell 1992;71:343-53. [Crossref] [PubMed]
- Zhang SH, Reddick RL, Piedrahita JA, et al. Spontaneous hypercholesterolemia and arterial lesions in mice lacking apolipoprotein E. Science 1992;258:468-71. [Crossref] [PubMed]
- Hjortnaes J, Butcher J, Figueiredo JL, et al. Arterial and aortic valve calcification inversely correlates with osteoporotic bone remodelling: a role for inflammation. Eur Heart J 2010;31:1975-84. [Crossref] [PubMed]
- Rico H, Hernandez ER, Cabranes JA, et al. Suggestion of a deficient osteoblastic function in diabetes mellitus: the possible cause of osteopenia in diabetics. Calcif Tissue Int 1989;45:71-3. [Crossref] [PubMed]
- Nuche-Berenguer B, Portal-Nunez S, Moreno P, et al. Presence of a functional receptor for GLP-1 in osteoblastic cells, independent of the cAMP-linked GLP-1 receptor. J Cell Physiol 2010;225:585-92. [Crossref] [PubMed]
- Ceccarelli E, Guarino EG, Merlotti D, et al. Beyond glycemic control in diabetes mellitus: effects of incretin-based therapies on bone metabolism. Front Endocrinol (Lausanne) 2013;4:73. [Crossref] [PubMed]
- Janghorbani M, Van Dam RM, Willett WC, et al. Systematic review of type 1 and type 2 diabetes mellitus and risk of fracture. Am J Epidemiol 2007;166:495-505. [Crossref] [PubMed]
- Koh WP, Wang R, Ang LW, et al. Diabetes and risk of hip fracture in the Singapore Chinese Health Study. Diabetes Care 2010;33:1766-70. [Crossref] [PubMed]
- Liao CC, Lin CS, Shih CC, et al. Increased risk of fracture and postfracture adverse events in patients with diabetes: two nationwide population-based retrospective cohort studies. Diabetes Care 2014;37:2246-52. [Crossref] [PubMed]
- Potikanond S, Rattanachote P, Pintana H, et al. Obesity does not aggravate osteoporosis or osteoblastic insulin resistance in orchiectomized rats. J Endocrinol 2016;228:85-95. [Crossref] [PubMed]
- Hofbauer LC, Brueck CC, Singh SK, et al. Osteoporosis in patients with diabetes mellitus. J Bone Miner Res 2007;22:1317-28. [Crossref] [PubMed]
- Vlassara H, Striker LJ, Teichberg S, et al. Advanced glycation end products induce glomerular sclerosis and albuminuria in normal rats. Proc Natl Acad Sci U S A 1994;91:11704-8. [Crossref] [PubMed]
- Stolzing A, Sellers D, Llewelyn O, et al. Diabetes induced changes in rat mesenchymal stem cells. Cells Tissues Organs 2010;191:453-65. [Crossref] [PubMed]
- Meng HZ, Zhang WL, Liu F, et al. Advanced Glycation End Products Affect Osteoblast Proliferation and Function by Modulating Autophagy Via the Receptor of Advanced Glycation End Products/Raf Protein/Mitogen-activated Protein Kinase/Extracellular Signal-regulated Kinase Kinase/Extracellular Signal-regulated Kinase (RAGE/Raf/MEK/ERK) Pathway. J Biol Chem 2015;290:28189-99. [Crossref] [PubMed]
- Liu JM, Rosen CJ, Ducy P, et al. Regulation of Glucose Handling by the Skeleton: Insights From Mouse and Human Studies. Diabetes 2016;65:3225-32. [Crossref] [PubMed]
- Lee NK, Sowa H, Hinoi E, et al. Endocrine regulation of energy metabolism by the skeleton. Cell 2007;130:456-69. [Crossref] [PubMed]
- Ferron M, McKee MD, Levine RL, et al. Intermittent injections of osteocalcin improve glucose metabolism and prevent type 2 diabetes in mice. Bone 2012;50:568-75. [Crossref] [PubMed]
- Xuan Y, Sun LH, Liu DM, et al. Positive association between serum levels of bone resorption marker CTX and HbA1c in women with normal glucose tolerance. J Clin Endocrinol Metab 2015;100:274-81. [Crossref] [PubMed]
- Zhou M, Ma X, Li H, et al. Serum osteocalcin concentrations in relation to glucose and lipid metabolism in Chinese individuals. Eur J Endocrinol 2009;161:723-9. [Crossref] [PubMed]
- Maddaloni E, D'Onofrio L, Lauria A, et al. Osteocalcin levels are inversely associated with Hba1c and BMI in adult subjects with long-standing type 1 diabetes. J Endocrinol Invest 2014;37:661-6. [Crossref] [PubMed]
- Im JA, Yu BP, Jeon JY, et al. Relationship between osteocalcin and glucose metabolism in postmenopausal women. Clin Chim Acta 2008;396:66-9. [Crossref] [PubMed]
- Ferron M, Wei J, Yoshizawa T, et al. Insulin signaling in osteoblasts integrates bone remodeling and energy metabolism. Cell 2010;142:296-308. [Crossref] [PubMed]
- Karsenty G, Ferron M. The contribution of bone to whole-organism physiology. Nature 2012;481:314-20. [Crossref] [PubMed]
- Fulzele K, Riddle RC, DiGirolamo DJ, et al. Insulin receptor signaling in osteoblasts regulates postnatal bone acquisition and body composition. Cell 2010;142:309-19. [Crossref] [PubMed]
- Liu TT, Liu DM, Xuan Y, et al. The association between the baseline bone resorption marker CTX and incident dysglycemia after 4 years. Bone Res 2017;5:17020. [Crossref] [PubMed]
- Janssen HC, Samson MM, Verhaar HJ. Vitamin D deficiency, muscle function, and falls in elderly people. Am J Clin Nutr 2002;75:611-5. [Crossref] [PubMed]
- Mieczkowska A, Irwin N, Flatt PR, et al. Glucose-dependent insulinotropic polypeptide (GIP) receptor deletion leads to reduced bone strength and quality. Bone 2013;56:337-42. [Crossref] [PubMed]
- Glorie L, D'Haese PC, Verhulst A. Boning up on DPP4, DPP4 substrates, and DPP4-adipokine interactions: Logical reasoning and known facts about bone related effects of DPP4 inhibitors. Bone 2016;92:37-49. [Crossref] [PubMed]
- Mamza J, Marlin C, Wang C, et al. DPP-4 inhibitor therapy and bone fractures in people with Type 2 diabetes - A systematic review and meta-analysis. Diabetes Res Clin Pract 2016;116:288-98. [Crossref] [PubMed]
- Majumdar SR, Josse RG, Lin M, et al. Does Sitagliptin Affect the Rate of Osteoporotic Fractures in Type 2 Diabetes? Population-Based Cohort Study. J Clin Endocrinol Metab 2016;101:1963-9. [Crossref] [PubMed]
- Fu J, Zhu J, Hao Y, et al. Dipeptidyl peptidase-4 inhibitors and fracture risk: an updated meta-analysis of randomized clinical trials. Sci Rep 2016;6:29104. [Crossref] [PubMed]
- Adil M, Khan RA, Kalam A, et al. Effect of anti-diabetic drugs on bone metabolism: Evidence from preclinical and clinical studies. Pharmacol Rep 2017;69:1328-40. [Crossref] [PubMed]
- Wolverton D, Blair MM. Fracture risk associated with common medications used in treating type 2 diabetes mellitus. Am J Health Syst Pharm 2017;74:1143-51. [Crossref] [PubMed]
- Driessen JH, van den Bergh JP, van Onzenoort HA, et al. Long-term use of dipeptidyl peptidase-4 inhibitors and risk of fracture: A retrospective population-based cohort study. Diabetes Obes Metab 2017;19:421-8. [Crossref] [PubMed]
- Ding KH, Wang ZZ, Hamrick MW, et al. Disordered osteoclast formation in RAGE-deficient mouse establishes an essential role for RAGE in diabetes related bone loss. Biochem Biophys Res Commun 2006;340:1091-7. [Crossref] [PubMed]
- Hamada Y, Kitazawa S, Kitazawa R, et al. The effects of the receptor for advanced glycation end products (RAGE) on bone metabolism under physiological and diabetic conditions. Endocrine 2010;38:369-76. [Crossref] [PubMed]
- Zhou G, Li C, Cai L. Advanced glycation end-products induce connective tissue growth factor-mediated renal fibrosis predominantly through transforming growth factor beta-independent pathway. Am J Pathol 2004;165:2033-43. [Crossref] [PubMed]
- Levin ME, Boisseau VC, Avioli LV. Effects of diabetes mellitus on bone mass in juvenile and adult-onset diabetes. N Engl J Med 1976;294:241-5. [Crossref] [PubMed]
- Raskin P, Stevenson MR, Barilla DE, et al. The hypercalciuria of diabetes mellitus: its amelioration with insulin. Clin Endocrinol (Oxf) 1978;9:329-35. [Crossref] [PubMed]