Are management decisions in critical patients changed with use of hemodynamic parameters from transpulmonary thermodilution technique?
Introduction
Hemodynamic monitoring plays an important role in the management of today’s acutely ill patient, particularly in the early stages of resuscitation. Monitoring modalities may help to identify underlying pathophysiological processes so that appropriate forms of therapy can be selected (1). Various monitoring systems are being developed and are available, in addition to conventional hemodynamic variables. The pulse contour analysis (PCA) and indicator dilution techniques [e.g., transpulmonary thermodilution (TPTD)] were introduced in the early 2000s (2). These advanced monitoring systems provide variables such as cardiac output (CO), stroke volume variation (SVV), pulmonary vascular permeability index (PVPI), and global end-diastolic volume (GEDV) using techniques that are less invasive than pulmonary artery catheterization (PAC). Despite development of hemodynamic monitoring, there are no optimal and universally applicable hemodynamic variables, which makes it difficult for physicians to choose and apply advanced hemodynamic monitoring.
In this survey on the utilization of advanced monitoring, survey respondents were provided variables of conventional monitoring, PCA, and TPTD for a patient with respiratory distress after a pedestrian traffic accident. We investigated the changes in decisions regarding patient management according to each variable, to evaluate whether advanced hemodynamic monitoring is helpful in decision-making for treatment.
Methods
Using a voting system at a medical conference, surveys were administered to 25 surgeons working in a nationally designated trauma center or on a trauma team. The survey involved the case of 67-year-old male who presented to the emergency room after a pedestrian traffic accident. On arrival, he was drowsy, with a Glasgow Coma Scale score of 10 and sluggish pupils reflex of 3 mm. Vital signs were as follows: blood pressure, 86/50 mmHg; pulse rate, 81 beats/min; respiratory rate, 20 breaths/min; and body temperature, 36.0 °C. The patient’s left leg below the knee joint was severely damaged, with active bleeding and extensive soft-tissue loss. Brain computed tomography (CT) scan showed tiny subdural hemorrhage, subarachnoid hemorrhage, and temporal bone fracture without mass effect. There was no chest or abdominal injury. Enhanced CT of the lower extremity showed an open, comminuted, displaced fracture in the neck of the fibula and dislocation of the proximal tibiofibular joint. During exploration of the injured leg, total destruction of the peroneal nerve, popliteal artery and vein were detected. Saphenous vein interposition was performed for the ruptured popliteal artery, and then open reduction and external fixation was done. A total 15 units of packed red blood cells and 16 units of fresh frozen plasma were transfused during the first 24 hours. Thirty hours later, color changes in the lower leg were observed due to graft failure; subsequently, leg amputation above the knee was performed. The VolumeView® system (Edwards Lifesciences Corp., Irvine, CA, USA) was used for patient monitoring. A central venous catheter with a thermistor was placed in the right subclavian vein, and a 4 French arterial catheter to assess volumetric parameters was placed in the right common femoral artery.
In the surveys, three clinical situations were given, and the decision choices given for each situation were as follow: immediately, 8 hours, and on the second day after amputation. Three kinds of variables from hemodynamic monitoring systems were provided for each clinical situation: conventional hemodynamic variables, including central venous pressure; variables from PCA; and variables from the TPTD technique. The variables from the Swan-Ganz catheter were not provided, given that the catheter was not used in this patient. Changes according to each provided hemodynamic variable were investigated and analyzed. Surveys were conducted blindly using the voting system.
Results
Physician demographics
Survey respondents comprised 12 general surgeons and 10 thoracic and cardiovascular surgeons. A total of 16 physicians had experience in managing critically ill injured patients of more than 5 years after completing their specialty training. Among them, 80% (20/25) of physicians knew how to interpret hemodynamic variables from PCA, and 68% (17/25) had used the FloTrac® system (Edwards Lifesciences Corp.) in an intensive care unit (ICU). Seventeen (68%) physicians were aware of the TPTD technique but only 12 (48%) had experience with it, including use of the VolumeView® system (Table 1).
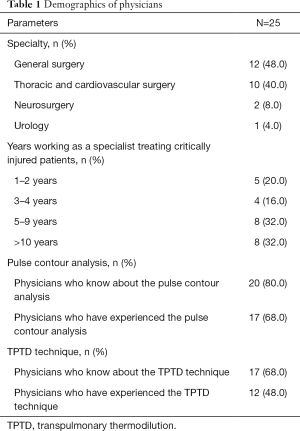
Full table
Situation #1: immediate postoperative status after amputation
Chest radiograph and conventional monitoring data are shown in Figure 1A. The total amount of fluids infused was 120 mL/h and urine output were 250–300 mL/h. Body weight was reduced from 89.0 to 84.5 kg after amputation. Administered drugs were as follows: norepinephrine, 25 µg/min; remifentanil, 0.05 µg/kg/min; and dexmedetomidine, 0.6 µg/kg/h. Arterial blood gas analysis (ABGA) showed pH 7.46, PaCO2 38 mmHg, PaO2 67 mmHg, HCO3 27 mmol/L, and SaO2 94%.
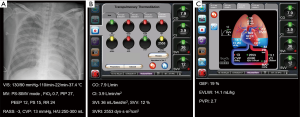
Figure 1B shows the hemodynamic variables from PCA: CO 7.9 L/min (normal range, 4.0–8.0 L/min); cardiac index (CI) 3.9 L/min/m2 (normal range, 2.5–4.0 L/min/m2); stroke volume index (SVI) 36 mL/beat/m2 (normal range, 33–47 mL/beat/m2); SVV 12% (normal range, <10–15%); systemic vascular resistance index (SVRI) 2,553 dyn·s/cm−5/m2 (normal range, 1,970–2,390 dyn·s/cm−5/m2).
Variables from the TPTD technique were as follows: global ejection fraction (GEF) 19% (normal range, 25–35%); extravascular lung water index (EVLWI) 14.1 mL/kg (normal range, 3–7 mL/kg); PVPI 2.7 (>3, lung injury; <3, hydrostatic or cardiogenic edema) (Figure 1C).
Surveys queried the choice of patient management with the variables given from each hemodynamic monitoring system. When only conventional variables were given, observation (n=8) and decrease of norepinephrine (n=8) were the most common responses of survey participants. With variables from PCA, 13 respondents chose to decrease norepinephrine. When variables from the TPTD technique were presented, 10 respondents chose to increase dobutamine (Figure 2).
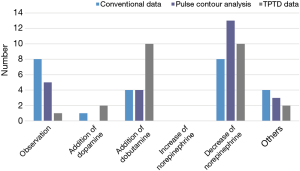
In this case, dobutamine (10 µg/kg/min) was initiated. Dexmedetomidine was stopped, and infusions of cisatracurium (5 mg/h) and propofol (30 µg/kg/min) were started for treatment of acute respiratory distress syndrome (ARDS), as a deep sedation strategy. The mode and setting of mechanical ventilation were changed as follows: pressure support/pressure-controlled ventilation mode; fraction of inspired oxygen (FiO2) 0.6; peak inspiratory pressure (PIP) 29 cmH2O; positive end-expiratory pressure (PEEP) 13 cmH2O; pressure support of 16 cmH2O; and respiratory rate 24/min. Two hours later, urine output was decreased to 100 mL/h; subsequently, continuous furosemide (8 mg/h) was started.
Situation #2: 8 hours after amputation
Chest radiograph and conventional monitoring data are shown in Figure 3A. The total amount of fluids infused was 100 mL/h and urine output were 200–300 mL/h. Body weight of the patient was decreased to 83.2 kg, 1.3 kg less than the previous day. Administered drugs were as follows: norepinephrine, 20 µg/min; dobutamine, 10 µg/kg/min; remifentanil, 0.05 µg/kg/min; propofol, 30 µg/kg/min; cisatracurium, 5 mg/h; and furosemide, 8 mg/h. ABGA showed pH 7.45, PaCO2 44 mmHg, PaO2 70 mmHg, HCO3 30.6 mmol/L, and SaO2 95%. Hemodynamic variables from PCA were as follows: CO 8.0 L/min; CI 3.9 L/min/m2; SVI 37 mL/beat/m2; SVV 11%; SVRI 1,981 dyn·s/cm−5/m2 (Figure 3B). Variables from the TPTD technique were GEF of 20%, EVLWI of 13.0 mL/kg, and PVPI of 2.5 (Figure 3C)
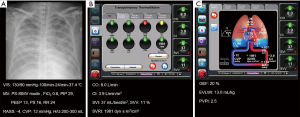
Surveys were conducted again, using the same method. Observation was the most common response with conventional variables (n=16), variables from PCA (n=16), and variables from TPTD techniques (n=11). Other responses included volume restriction and increasing the amount of furosemide infusion (Figure 4).
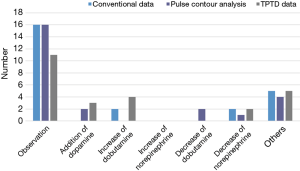
In clinical practice situation #2, primarily observation was chosen. Twelve hours later, norepinephrine (15 µg/min) and furosemide (5 mg/h) were decreased because vital signs were stable and hourly urine output was increased (>300 mL/h for 2 hours).
Situation #3: second postoperative day after amputation
Chest radiograph and conventional monitoring data are shown in Figure 5A. The total amount of fluids infused was 100 mL/h and urine output were 150–300 mL/h. Body weight decreased a total 2.2 kg, compared with the previous day. Drugs administered were as follows: norepinephrine 15 µg/min; dobutamine 10 µg/kg/min; remifentanil 0.05 µg/kg/min; propofol 30 µg/kg/min; cisatracurium 5 mg/h; and furosemide 5 mg/h. ABGA showed pH 7.55, PaCO2 33 mmHg, PaO2 108 mmHg, HCO3 30.9 mmol/L, and SaO2 99%.
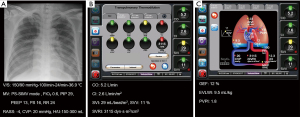
Variables from PCA were as follows: CO 5.2 L/min; CI 2.6 L/min/m2; SVI 29 mL/beat/m2; SVV, 11%; and SVRI, 3,115 dyn·s/cm−5/m2 (Figure 5B). Variables from the TPTD technique were GEF of 12%, EVLWI of 9.5 mL/kg, and PVPI of 1.8 (Figure 5C).
In the survey, decrease of norepinephrine was the most common response with conventional variables (n=16), variables from PCA (n=24), and variables from TPTD techniques (n=8). Seven and five participants changed to addition of dopamine or dobutamine, respectively, when variables from TPTD were given (Figure 6).
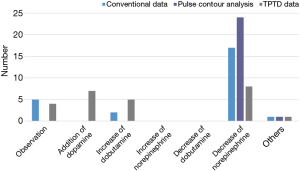
For patient management in situation #3, primarily dopamine (10 µg/kg/min) was initiated; norepinephrine was then slowly tapered off. Because point-of-care ultrasound showed bilateral fluid accumulation in the thorax, closed thoracostomy with a small-bore catheter was performed.
Clinical course on the third day after amputation
Clinical and hemodynamic variables are shown in Figure 7. Infused drugs and their amounts were as follows: norepinephrine 8 µg/min; dobutamine 10 µg/kg/min; dopamine 10 µg/kg/min; remifentanil 0.05 µg/kg/min; dexmedetomidine 0.5 µg/kg/h; and furosemide 2 mg/h. ABGA showed pH 7.46, PaCO2 45 mmHg, PaO2 69 mmHg, HCO3 32.8 mmol/L, and SaO2 98%. The patient’s respiratory and hemodynamic condition was stable throughout the treatment course and he was discharged without any complication.
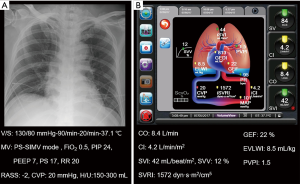
Final survey results
After completion of the patient management decision surveys, a questionnaire was administered querying whether the decisions made were influenced by advanced hemodynamic monitoring methods. A total 88% (22/25) of physicians stated that their decision changed when variables from advanced monitoring were presented. Among them, 82% (18/22) of physicians chose hemodynamic variables from the TPTD technique as their reason for the change (Figure 8).
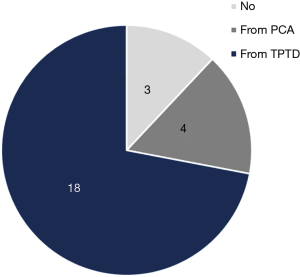
Discussion
The key principles of hemodynamic monitoring include providing accurate and reproducible measurements of relevant variables, which can guide therapy. The method chosen must also be easy to use, readily available, operator independent, and cost effective (1). Physicians should try to choose devices that have these features and that are appropriate for each clinical situation. During the past several decades, technologies of hemodynamic monitoring have improved, from being invasive to noninvasive and from a static to a functional approach (3). With the help of advanced monitoring, the management of critically ill patients has also evolved.
However, as yet, there are no optimal hemodynamic variables that are universally applicable to all patients. Even though thermodilution with PAC, which was introduced in the early 1970s, has been considered optimal for CO monitoring, this approach is extremely invasive and has failed to show improvement in mortality when applied in critically ill patients (4-6). The use of PAC has decreased with the advent of newer technologies. According to a Swiss web-based survey regarding the kind of monitoring used in the adult ICU, echocardiography (95%), PAC (85%), and TPTD (82%) were the most commonly available. It seems that the PAC method is being progressively replaced by new monitoring techniques, such as TPTD, which was the preferred technique in our survey (7). Another survey conducted among ICU clinicians in 16 European countries also showed that TPTD was the preferred technique for measuring CO (8). However, a survey performed in Belgium and Germany on knowledge of the TPTD technique revealed that knowledge, interpretation, and measurement techniques were all suboptimal among ICU personnel, although these methods were used regularly (9).
As for the choice of monitoring system, clinicians must be aware of the most appropriate modality for each clinical situation, according to the system’s advantages and limitations. With regard to quantifying pulmonary edema, the measurement of EVLWI can provide early detection of lung water accumulation, to more optimally guide fluid therapy by differentiating hydrostatic versus increased pulmonary capillary permeability (2), as in this case. Measured EVLWI that is utilized in managing fluid balance in ARDS (10,11) has shown lower cumulative fluid balance, decreased ICU mortality (12), reduced duration of mechanical ventilation, and shorter length of ICU stay (13). A previous study that aimed to evaluate the relationship between measured EVLWI, pulmonary vascular permeability using the TPTD technique, and severity categories as defined by the Berlin definition (14) showed an association between increased EVLWI and pulmonary vascular permeability (15). Moreover, most studies consider that TPTD is as reliable as PCA (16-19) and outperforms uncalibrated devices (20-22). As SVV measured by PCA has been shown to be a good predictor of fluid responsiveness in various clinical settings (23), TPTD can also be utilized to guide fluid therapy, such as during the early resuscitation phase, to achieve a minimum level of preload to allow for sufficient vital organ perfusion (24). TPTD may show less respiratory phase-dependent variation (25).
Despite the theoretical advantages of advanced monitoring, its clinical effectiveness has been inconsistent as monitoring itself does not guarantee improvement in patient survival unless it is paired with a proper therapeutic protocol. In a recent study, TPTD-based fluid management failed to show improved outcome and caused a more positive fluid balance in non-septic shock patients (26). With respect to septic shock, TPTD is considered superior to PAC by intensivists (8); however, TPTD has not been associated with improved survival (26,27). TPTD, as well as PCA, becomes unreliable when CO is very low. Frequent recalibration is required when the vasomotor tone changes with both PCA and TPTD (5,28-30). TPTD also remains considerably invasive because it requires a specific femoral artery catheter (3).
This study has several limitations. First, only surgeons participated, and the number of participants was relatively small. Intensivists, who could have provided additional valuable information, were not available to participate in this survey. Second, half of the respondents had never used hemodynamic monitoring with the TPTD technique before the survey. Third, the patient was not fully sedated and was mechanically ventilated using lung-protective settings; therefore, there might be technical errors because the recommendations for ventilation, such as full sedation, high tidal volume, and low PEEP, were not followed. High PEEP and low tidal volume may have interfered with heart-lung interaction and resulted in reduced accuracy of the variables. Fourth, the clinical efficacy of TPTD was unevaluable because this study was based on a single case. Fifth, the variables from Swan-Ganz catheter were not provided, given that the catheter was not used in this patient. Such information could have aided further comparisons between invasive and non-invasive techniques.
However, in this case, with the help of PAC and TPTD monitoring, respiratory distress in the patient was managed for cardiogenic pulmonary edema, as EVLWI and PVPI were within the normal ranges and GEF was only 12–19%. In addition, decisions were not made using a single value but rather using trends in the variables. On the first postoperative day, two-dimensional echocardiography was done to check heart function, which showed stress-induced cardiomyopathy with lowered ejection fraction (below 40%); both results suggest that the main cause of edema was cardiogenic. Therefore, TPTD provided valuable information for establishing an optimal treatment strategy in a patient with ARDS.
Conclusions
In summary, decisions regarding patient management may be changed using various parameters from different hemodynamic monitoring systems. Although there is no optimal method, use of an advanced monitoring system might be helpful in decision-making for critically ill patients. Information of multiple parameters and trends in change from advanced hemodynamic monitoring may be more valuable than a single value in making such decisions. If clinicians select the system that is most appropriate in each case, according to the system’s advantages and limitations, and interpretation is based on correctly obtained variables, use of parameters from different monitoring systems could boost the effectiveness of patient management. The accumulation of experiences, research, and technical developments in hemodynamic monitoring will lead to improved management of critically ill patients.
Acknowledgments
None.
Footnote
Conflicts of Interest: This abstract was presented by oral presentation to the English session in the 45th annual meeting of the Japanese society of intensive care medicine.
Ethical Statement: The authors are accountable for all aspects of the work in ensuring that questions related to the accuracy or integrity of any part of the work are appropriately investigated and resolved. The need for ethics approval and consent of the present study was waived by Institutional Review Board of the Dankook University Hospital. Written informed consent was obtained from all patients.
References
- Vincent JL, Rhodes A, Perel A, et al. Clinical review: Update on hemodynamic monitoring--a consensus of 16. Crit Care 2011;15:229. [Crossref] [PubMed]
- Assaad S, Shelley B, Perrino A. Transpulmonary Thermodilution: Its Role in Assessment of Lung Water and Pulmonary Edema. J Cardiothorac Vasc Anesth 2017;31:1471-80. [Crossref] [PubMed]
- Ramsingh D, Alexander B, Cannesson M. Clinical review: Does it matter which hemodynamic monitoring system is used? Crit Care 2013;17:208. [Crossref] [PubMed]
- Sandham JD, Hull RD, Brant RF, et al. A randomized, controlled trial of the use of pulmonary-artery catheters in high-risk surgical patients. N Engl J Med 2003;348:5-14. [Crossref] [PubMed]
- Richard C, Warszawski J, Anguel N, et al. Early use of the pulmonary artery catheter and outcomes in patients with shock and acute respiratory distress syndrome: a randomized controlled trial. JAMA 2003;290:2713-20. [Crossref] [PubMed]
- Harvey S, Harrison DA, Singer M, et al. Assessment of the clinical effectiveness of pulmonary artery catheters in management of patients in intensive care (PAC-Man): a randomised controlled trial. Lancet 2005;366:472-7. [Crossref] [PubMed]
- Siegenthaler N, Giraud R, Saxer T, et al. Haemodynamic monitoring in the intensive care unit: results from a web-based Swiss survey. Biomed Res Int 2014;2014:129593. [Crossref] [PubMed]
- Torgersen C, Dunser MW, Schmittinger CA, et al. Current approach to the haemodynamic management of septic shock patients in European intensive care units: a cross-sectional, self-reported questionnaire-based survey. Eur J Anaesthesiol 2011;28:284-90. [PubMed]
- Hofkens PJ, Verrijcken A, Merveille K, et al. Common pitfalls and tips and tricks to get the most out of your transpulmonary thermodilution device: results of a survey and state-of-the-art review. Anaesthesiol Intensive Ther 2015;47:89-116. [Crossref] [PubMed]
- Michard F. Bedside assessment of extravascular lung water by dilution methods: temptations and pitfalls. Crit Care Med 2007;35:1186-92. [Crossref] [PubMed]
- Berkowitz DM, Danai PA, Eaton S, et al. Accurate characterization of extravascular lung water in acute respiratory distress syndrome. Crit Care Med 2008;36:1803-9. [Crossref] [PubMed]
- Eisenberg PR, Hansbrough JR, Anderson D, et al. A prospective study of lung water measurements during patient management in an intensive care unit. Am Rev Respir Dis 1987;136:662-8. [Crossref] [PubMed]
- Mitchell JP, Schuller D, Calandrino FS, et al. Improved outcome based on fluid management in critically ill patients requiring pulmonary artery catheterization. Am Rev Respir Dis 1992;145:990-8. [Crossref] [PubMed]
- Ranieri VM, Rubenfeld GD, Thompson BT, et al. Acute respiratory distress syndrome: the Berlin Definition. JAMA 2012;307:2526-33. [PubMed]
- Kushimoto S, Endo T, Yamanouchi S, et al. Relationship between extravascular lung water and severity categories of acute respiratory distress syndrome by the Berlin definition. Crit Care 2013;17:R132. [Crossref] [PubMed]
- Della Rocca G, Costa MG, Coccia C, et al. Cardiac output monitoring: aortic transpulmonary thermodilution and pulse contour analysis agree with standard thermodilution methods in patients undergoing lung transplantation. Can J Anaesth 2003;50:707-11. [Crossref] [PubMed]
- Cottis R, Magee N, Higgins DJ. Haemodynamic monitoring with pulse-induced contour cardiac output (PiCCO) in critical care. Intensive Crit Care Nurs 2003;19:301-7. [Crossref] [PubMed]
- Cecconi M, Dawson D, Grounds RM, et al. Lithium dilution cardiac output measurement in the critically ill patient: determination of precision of the technique. Intensive Care Med 2009;35:498-504. [Crossref] [PubMed]
- Sakka SG, Reuter DA, Perel A. The transpulmonary thermodilution technique. J Clin Monit Comput 2012;26:347-53. [Crossref] [PubMed]
- Zollner C, Haller M, Weis M, et al. Beat-to-beat measurement of cardiac output by intravascular pulse contour analysis: a prospective criterion standard study in patients after cardiac surgery. J Cardiothorac Vasc Anesth 2000;14:125-9. [Crossref] [PubMed]
- Bein B, Meybohm P, Cavus E, et al. The reliability of pulse contour-derived cardiac output during hemorrhage and after vasopressor administration. Anesth Analg 2007;105:107-13. [Crossref] [PubMed]
- Johansson A, Chew M. Reliability of continuous pulse contour cardiac output measurement during hemodynamic instability. J Clin Monit Comput 2007;21:237-42. [Crossref] [PubMed]
- Suehiro K, Tanaka K, Matsuura T, et al. The Vigileo-FloTrac system: arterial waveform analysis for measuring cardiac output and predicting fluid responsiveness: a clinical review. J Cardiothorac Vasc Anesth 2014;28:1361-74. [Crossref] [PubMed]
- Sanchez M, Garcia-de-Lorenzo A, Herrero E, et al. A protocol for resuscitation of severe burn patients guided by transpulmonary thermodilution and lactate levels: a 3-year prospective cohort study. Crit Care 2013;17:R176. [Crossref] [PubMed]
- Sakka SG, Reinhart K, Meier-Hellmann A. Comparison of pulmonary artery and arterial thermodilution cardiac output in critically ill patients. Intensive Care Med 1999;25:843-6. [Crossref] [PubMed]
- Trof RJ, Beishuizen A, Cornet AD, et al. Volume-limited versus pressure-limited hemodynamic management in septic and nonseptic shock. Crit Care Med 2012;40:1177-85. [Crossref] [PubMed]
- Zhang Z, Ni H, Qian Z. Effectiveness of treatment based on PiCCO parameters in critically ill patients with septic shock and/or acute respiratory distress syndrome: a randomized controlled trial. Intensive Care Med 2015;41:444-51. [Crossref] [PubMed]
- Bendjelid K. When to recalibrate the PiCCO? From a physiological point of view, the answer is simple. Acta Anaesthesiol Scand 2009;53:689-90. [Crossref] [PubMed]
- Monnet X, Anguel N, Naudin B, et al. Arterial pressure-based cardiac output in septic patients: different accuracy of pulse contour and uncalibrated pressure waveform devices. Crit Care 2010;14:R109. [Crossref] [PubMed]
- Monnet X, Letierce A, Hamzaoui O, et al. Arterial pressure allows monitoring the changes in cardiac output induced by volume expansion but not by norepinephrine. Crit Care Med 2011;39:1394-9. [Crossref] [PubMed]