Neurally adjusted ventilatory assist versus pressure support ventilation in patient-ventilator interaction and clinical outcomes: a meta-analysis of clinical trials
Introduction
Assisted ventilatory modes target at satisfying the ventilator insufflation to the patient’s effort. However, they always do not match patients’ effort. Mismatching between patient demand and level of assistance is potentially harmful; under assistance and over assistance may both produce patient-ventilator asynchrony and lead to poor clinical outcomes (1,2). Asynchrony can be improved by optimum adjustment of ventilator settings [e.g., a lower level of support, inspiratory trigger, setting up external positive end expiratory pressure (PEEP)], although asynchronies still exert and influence ventilated patients after optimal adjustments.
Pressure support ventilation (PSV) is one of the main assisted ventilatory modes. The problem of this kind of technology is ventilator asynchrony, a mismatch between the patient’s neural output and the ventilator’s inspiratory and expiratory times (2). NAVA, a new mode of ventilator, uses the electrical activity of the diaphragm to drive the ventilator deliver positive pressure to trigger and cycle inspiration in proportion to the patients’ effort (3). The NAVA can adapt each change in the patient’s ventilatory demand, and better keep a harmonious relationship between the ventilator assistance and the patient’s effort. The advantage of NAVA is to identify the start of neural exhalation, which cannot be recognized by PSV. Nowadays, a new setting of NAVA occurs, which is named neurally controlled pressure support ventilation, it sets the NAVA level at 15 cmH2O/uv with an upper airway pressure (Paw) limit to obtain the same overall Paw applied during pneumatically triggered and cycled-off pressure support (4-7).
Furthermore, some studies showed that the NAVA could improve patient-ventilator synchrony and create a more natural breathing pattern, which leaded to better comfort and oxygenation (8,9). All the advantages above make NAVA an attractive alternative for patients experiencing clinically significant asynchrony. Before 2019, only one letter published for this theory (10), which merely talking about asynchrony index (AI). In 2019, two recent meta-analysis studies about NAVA have been published (11,12). In the meta-analysis conducted by Pettenuzzo et al. (12), they included the studies contained exact AI value or severe level of AI (AI >10%), but our study only contained the articles talking about exact AI value. The number of studies containing exact AI value in their article is 14, however, we found 5 articles cannot include in our studies, one of them was not published in English (13), 3 of them exactly did not contain AI value in their studies through reading the whole articles (7,14,15), the last one contained many AI value in different levels of support (16), so we cannot choose the best one to include in our meta-analysis. In the end, we try to collect and take the published studies to make another meta-analysis for confirmation.
Methods
Search strategy
Two investigators independently searched the articles in the databases (PubMed, the Web of Science, Scope, Medline). The reference lists of eligible studies and relevant papers were also manually searched and reviewed. Searching terms included “NAVA”, “neurally adjusted ventilatory assist” and “asynchrony”. In PubMed, we used (“Neurally adjusted ventilated assist” or “NAVA”) and (“asynchrony” or “synchrony”) for search strategy. Searching terminal date was 2019/6/13. Firstly, we found 406 articles after duplications excluded by reading the title and abstract, and then excluded 101 articles by reading the title and abstract. Finally, 12 articles were left after reading the whole articles (4,5,9,17-25) (Figure 1).
Inclusion and exclusion
Inclusions contain: (I) researched study comparing NAVA with PSV in adult patients, (II) primary outcome: asynchrony index, (III) only be published in English.
Exclusions contain: (I) review, retrospective research, case report, (II) insufficient data in the articles (insufficiency of data mainly indicated that the study did not contain the exact asynchrony index value (e.g., Asynchrony index >10% is not the same as the exact asynchrony index value (continuous variable).
Definition
Ventilator asynchrony can be classified as ineffective efforts, double-triggering, auto-triggering, and premature triggering (26,27). AI, one of the important indicators, is defined as the number of asynchrony events divided by the total respiratory cycles computed as the sum of the number of ventilator cycles (triggered or not) and of wasted efforts (12). Ineffective efforts occur when the patient’s inspiratory effort fails to trigger a ventilator breath (28,29). Double triggering occurs when the patient’s ventilatory demand is high and the ventilator inspiratory time is short (30). Auto triggering is a cycle transmitted by the ventilator in the absence of patient effort and can be generated by cardiogenic oscillations or leaks in the ventilator circuit (31).
Data elected
Two authors independently reviewed the identified abstracts and selected articles to full review. The third reviewer addressed the discrepancies. For each selected publication, the following baseline and study characteristics were extracted: first author, publication year, country, participant characteristics, predications for enrolling in the study, and the baseline characteristics of these studies were concluded (Table 1). The risk of bias of the included studies was shown in Figure 2 and Figure 3. The results showed that all the studies were comparable and could be integrated (all were prospective studies). Efficacy outcome measures were AI, ineffective efforts, auto-triggering, double triggering, premature triggering, VT, MV, Pawpeak, Pawmean, EAdipeak, P0.1, VT/EAdi, ICU mortality, duration of ventilation, ICU stay time, and hospital stay time.
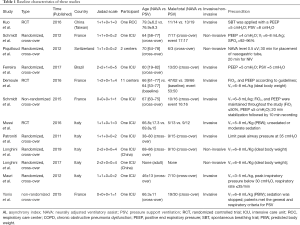
Full table
Risk of bias assessment
Risk of bias of trials included in this meta-analysis was assessed according to the recommendations of the Cochrane Handbook of Systematic Reviews of Interventions, in the following domains: selection bias (random sequence generation and allocation concealment), performance bias (blinding of participants and personnel), detection bias (blinding of outcome assessment), attrition bias (incomplete outcome data), and reporting bias (selective outcome reporting) (http://handbook.cochrane.org). Jadad scale used to calculate the quality of every enrolled study. The quality appraisal mostly based on whether the authors added quality appraisal indicators (e.g., whether said in the article about the concealment of randomization, whether said about the randomization number occurring) in their articles.
Statistic analysis
We pooled data and used mean difference [MD, with 95% confidence interval (CI)] for continuous outcomes: AI, ineffective efforts, auto-triggering, double triggering, premature triggering, Pawpeak, Pawmean, VT, MV, EAdi, P 0.1, EAdi/VT,, duration of ventilation, hospital stay time, and ICU stay time. Odds ratio (OR) was used for dichotomy variable: ICU mortality. We would use a fixed-effect model if there was no considerable heterogeneity among studies. We would use a random-effects model if the I2 statistic was above 50% and Cochran’s Q statistic had a P value ≤0.1. Subgroup analyses were performed to compare AI grouped by mechanical ventilation, and by adult because of the high heterogeneity. Funnel plots were used to screen for potential publication bias. All statistical analyses were carried out with Review Manager 5.3 (The Cochrane Collaboration).
Results
We found 406 articles after duplications excluded, and then excluded 224 articles by reading the title and abstract. Finally, 12 articles were left after reading the whole articles (4,5,9,17-25) (Figure 1). The studies included in our meta-analysis were all prospective clinical trials, published from 2012 to 2019. The studies were conducted in China (19), France (17,21,22,25,32), Switzerland (20), Brazil (18), and Italy (4,5,9,23,24). Table 1 presents the basic characteristics of included trials and demographic data of participants. Two trials were multicenter studies and the Jadad Scales of all included studies ranged from 1 to 5, the relatively low scores of the included studies must result from the particularity of these studies that investigated the kinds of ventilation modes. In these studies, the blind methods cannot be implemented. However, all the studies included in our meta-analysis were prospective studies, so it is higher quality than common retrospective studies. The risk of bias and Jadad score showed that the most the studies contained were randomized studies (only two of them were non-randomized studies), although only four of them the randomized allocation methods. The blindness cannot be applied in all the studies. Other bias in Figure 2 and Figure 3 mainly talked about publish bias and so on (Figure 2, Figure 3 and Table 1).
Patient-ventilator asynchrony
AI
For the AI, our study included 12 studies with a total of 331 adult patients; the results comparing groups were significantly lower in NAVA group (224 patients) than PSV group (225 patients) [mean difference (MD) −12.82, 95% confidence interval (CI): −21.20 to −4.44]. Heterogeneity testing showed that I2=88%, indicating high heterogeneity. Because of the high heterogeneity, we used the subgroup analysis to solve it. Subgroup analysis grouped by mechanical ventilation showed that the AI of NAVA was lower than PSV in invasive mechanical ventilation (MD −9.52, 95% CI: −17.85 to −1.20, I2=87%), and non-invasive ventilation (NIV) (MD −24.55, 95% CI: −35.40 to −13.70, I2=0%). Subgroup analysis grouped by randomized research design (randomized design or non-randomized design) demonstrated that NAVA had lower AI in randomized design (MD −16.79, 95% CI: −25.35 to −8.24, I2=69%), and did not show benefit in non-randomized design (MD 0.36, 95% CI: −3.24 to 3.96, I2=18%). Subgroup analysis grouped by Jadad score ≥4 or <4 showed that the AI of NAVA was lower than PSV in Jadad score ≥4 (MD −12.18, 95% CI: −17.79 to −6.57, I2=0%), and <4 (MD −13.93, 95% CI: −24.79 to −3.08, I2 =88%) (Figures 4-6).
Ineffective efforts
For presenting the result of ineffective efforts, our study included 6 studies (9,20-22,24,25) involving a total of 197 events, and showed that NAVA (99 patients) was not significantly different from PSV (98 patients) (MD 0.05, 95% CI: −0.19 to 0.28, I2=0%), although lacking significantly different evidence. Heterogeneity testing showed that I2=0%, indicating high heterogeneity (Figure 7).
Auto-triggering
For the result of Auto-triggering, our study enrolled 6 studies (9,20-22,24,25), including a total of 197 events, and the result demonstrated that NAVA (99 patients) was significantly lower than the PSV (98 patients) (MD −0.28, 95% CI: −0.51 to −0.05, I2=10%). Heterogeneity testing showed that I2=10%, indicating low heterogeneity (Figure 8).
Double triggering
For presenting the result of Double triggering, our study enrolled 6 studies (9,20-22,24,25), including a total of 197 events, and demonstrated that NAVA (99 patients) was not significantly higher than PSV (98 patients) (MD 0.10, 95% CI: −0.02 to 0.22, I2=12%). Heterogeneity testing showed that I2=12%, indicating low heterogeneity (Figure 9).
Premature triggering
For presenting the result of premature triggering, our study included 3 studies (20,21,24) and a total of 80 events, and showed that NAVA group (40 patients) was significantly lower than PSV group (40 patients) in premature triggering (MD −2.49, 95% CI: −3.77 to −1.21, I2=29%). Heterogeneity testing showed that I2=29%, indicating low heterogeneity (Figure 10).
Clinical outcomes
ICU mortality
For the result of ICU mortality, our study included 3 studies (9,17,19) and a total of 186 patients, ICU mortality (OR 0.50, 95% CI: 0.23 to 1.08, I2=0%) did not reflect significant difference in groups. Heterogeneity testing showed that I2=0%, indicating low heterogeneity (Figure 11).
Duration of ventilation
For the result of ventilation days, our study included 2 studies (17,19) and a total of 161 patients, and showed that NAVA was significantly lower than PSV in ventilation days (MD −2.82, 95% CI: −5.55 to −0.08, I2=0%). Heterogeneity testing showed that I2=0%, indicating low heterogeneity (Figure 12).
ICU stay time
For the result of ICU days, our study included 2 studies (17,19)and a total of 161 patients, ICU days (MD 1.96, 95% CI: −2.09 to 6.01, I2=0%) did not reflect significant difference in groups. Heterogeneity testing showed that I2=0%, indicating low heterogeneity (Figure 13).
Hospital stay time
For the result of hospital days, our study included 2 studies (17,19) and a total of 161 patients, hospital days (MD 2.07, 95% CI: −1.99 to 6.13, I2=0%) did not reflect significant difference in groups. Heterogeneity testing showed that I2=0%, indicating low heterogeneity (Figure 14).
Breathing pattern respiratory muscle unloading
For the Breathing pattern (Pawpeak, Pawmean, VT, MV) and respiratory muscle unloading (EAdipeak, P 0.1, VT/EAdi), NAVA and PSV did not show significant differences (Figures S1-S7).
Potential publication bias of AI was performed and shown as funnel plot. The result of funnel plot showed that it might exist publication bias for the AI outcome (Figure 15).
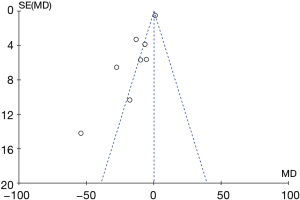
Discussion
Comparing NAVA with PSV, our study showed that NAVA could significantly help for patient-ventilation interaction (AI, auto triggering, premature triggering) in adult ventilated patients. The results were similar to the meta-analysis conducted by Pettenuzzo et al. (12). However, their studies did not contain the subgroup analysis between invasive and non-invasive mechanical ventilation, the comparisons of respiratory muscle unloading, and clinical outcomes.
As the primary outcome in our study, AI is defined as the number of asynchrony events divided by the total respiratory cycles computed as the sum of the number of ventilator cycles (triggered or not) and of wasted efforts (12). The computation of AI as the percentage of patents with AI greater than 10% (i.e., severe asynchrony) was also considered in clinical practice, as this threshold of asynchrony was found to be associated with worse clinical outcome (12).
Subgroup analyses grouped by mechanical ventilation, randomized design, and Jadad scores all showed NAVA had lower AI than PSV. During NIV, the occurrence of leaks may greatly affect patient-ventilator interactions, thereby be difficult to determine the optimal settings of ventilator (33). In NAVA, assistance is delivered based on neural triggering, which is not affected by leak, and can improve the tolerance.
In theory, according to the mechanism of NAVA, the EAdi triggers the assist when the patient initiates an inspiratory effort, and a decrease in EAdi terminates the assist. NAVA does not depend on measurements of airway pressure or flow and keeps the assist synchronous with the inspiratory efforts (14,34-37). Thus, NAVA has two important features: the transmitted pressure is simultaneous with the diaphragmatic activity, and the VT is controlled by the output of the patient’s respiratory center (3).
In our study, the duration of ventilation significantly decreased in NAVA group than the control group. Ventilator asynchrony is associated with prolonged mechanical ventilation, prolonged ICU and hospital stays, and increased mortality (26). Although in our study, two groups were not significantly different in the ICU mortality, ICU stay time and hospital stay time.
In our study, the ventilator-related complications (e.g., barotrauma, VAP) were lack in the comparisons. Under assistance and over assistance may both produce patient-ventilator asynchrony that is associated with poorer clinical outcomes. In our study, the duration of ventilation is longer in NAVA than PSV. The longer duration of mechanical ventilation is associated with increased incidence of ventilator-associated pneumonia.
In clinical practice, when patient-ventilator asynchrony cannot be reversed by sedation or up-regulate PEEP, NAVA could be the good choice. In these 12 enrolled articles, only 8 studies showed underlying disease [chronic obstructive pneumonia disease (19), acute respiratory distress syndrome, (24), and acute respiratory failure (4,9,17,20,22,23)] that led to mechanical ventilation. In theory, NAVA application in patients at risk of patient-ventilator asynchrony, ventilator-induced lung injury, and respiratory muscle atrophy should be recommended. Nowadays, some studies using EAdi signal in mechanically ventilated adults for detecting asynchrony, and titrating neural drive and sedation. Furthermore, NAVA could reduce the economic burden of ICU patients. NAVA has its limitations, such as contraindications to EAdi catheter placement (e.g., recent gastric or esophageal surgery and the presence of esophageal varicose veins), presence of a tracheotomy, a progressive infectious process (e.g., nosocomial pneumonia, nosocomial bacteremia, and hemodynamic failure) (25).
In addition, NAVA also has some problems. In the one hand, the accurate positioning of the NAVA catheter is necessary (38). In the other hand, the body position, PEEP and intra-abdominal pressure can all affect the position of the diaphragm (39).
Conclusions
NAVA is more beneficial in ventilator-people interaction and clinical outcomes than PSV.
Acknowledgments
None.
Footnote
Conflicts of Interest: The authors have no conflicts of interest to declare.
Ethical Statement: The authors are accountable for all aspects of the work in ensuring that questions related to the accuracy or integrity of any part of the work are appropriately investigated and resolved.
References
- de Wit M, Miller KB, Green DA, et al. Ineffective triggering predicts increased duration of mechanical ventilation. Crit Care Med 2009;37:2740-5. [PubMed]
- Thille AW, Rodriguez P, Cabello B, et al. Patient-ventilator asynchrony during assisted mechanical ventilation. Intensive Care Med 2006;32:1515-22. [Crossref] [PubMed]
- Sinderby C, Navalesi P, Beck J, et al. Neural control of mechanical ventilation in respiratory failure. Nat Med 1999;5:1433-6. [Crossref] [PubMed]
- Longhini F, Liu L, Pan C, et al. Neurally-Adjusted Ventilatory Assist for Noninvasive Ventilation via a Helmet in Subjects With COPD Exacerbation: A Physiologic Study. Respir Care 2019;64:582-9. [Crossref] [PubMed]
- Longhini F, Pan C, Xie J, et al. New setting of neurally adjusted ventilatory assist for noninvasive ventilation by facial mask: a physiologic study. Crit Care 2017;21:170. [Crossref] [PubMed]
- Cammarota G, Longhini F, Perucca R, et al. New Setting of Neurally Adjusted Ventilatory Assist during Noninvasive Ventilation through a Helmet. Anesthesiology 2016;125:1181-9. [Crossref] [PubMed]
- Liu L, Xia F, Yang Y, et al. Neural versus pneumatic control of pressure support in patients with chronic obstructive pulmonary diseases at different levels of positive end expiratory pressure: a physiological study. Crit Care 2015;19:244. [Crossref] [PubMed]
- Lee J, Kim HS, Sohn JA, et al. Randomized crossover study of neurally adjusted ventilatory assist in preterm infants. J Pediatr 2012;161:808-13. [Crossref] [PubMed]
- Di Mussi R, Spadaro S, Mirabella L, et al. Impact of prolonged assisted ventilation on diaphragmatic efficiency: NAVA versus PSV. Crit Care 2016;20:1. [Crossref] [PubMed]
- Sehgal IS, Dhooria S, Aggarwal AN, et al. Asynchrony index in pressure support ventilation (PSV) versus neurally adjusted ventilator assist (NAVA) during non-invasive ventilation (NIV) for respiratory failure: systematic review and meta-analysis. Intensive Care Med 2016;42:1813-5. [Crossref] [PubMed]
- Bruni A, Garofalo E, Pelaia C, et al. Patient-ventilator asynchrony in adult critically ill patients. Minerva Anestesiol 2019;85:676-88. [Crossref] [PubMed]
- Pettenuzzo T, Aoyama H, Englesakis M, et al. Effect of Neurally Adjusted Ventilatory Assist on Patient-Ventilator Interaction in Mechanically Ventilated Adults: A Systematic Review and Meta-Analysis. Crit Care Med 2019;47:e602-9. [Crossref] [PubMed]
- Wallet F, Wallon G, Pecquerie N, et al. Impact de la NAVA sur l’asynchronisme patient machine en post operatoire de chirurgie abdominale. Annales Françaises d'Anesthésie et de Réanimation 2014;33:A134-5. [Crossref]
- Colombo D, Cammarota G, Bergamaschi V, et al. Physiologic response to varying levels of pressure support and neurally adjusted ventilatory assist in patients with acute respiratory failure. Intensive Care Med 2008;34:2010-8. [Crossref] [PubMed]
- Vaschetto R, Cammarota G, Colombo D, et al. Effects of propofol on patient-ventilator synchrony and interaction during pressure support ventilation and neurally adjusted ventilatory assist. Crit Care Med 2014;42:74-82. [Crossref] [PubMed]
- Carteaux G, Cordoba-Izquierdo A, Lyazidi A, et al. Comparison Between Neurally Adjusted Ventilatory Assist and Pressure Support Ventilation Levels in Terms of Respiratory Effort. Crit Care Med 2016;44:503-11. [Crossref] [PubMed]
- Demoule A, Clavel M, Rolland-Debord C, et al. Neurally adjusted ventilatory assist as an alternative to pressure support ventilation in adults: a French multicentre randomized trial. Intensive Care Med 2016;42:1723-32. [Crossref] [PubMed]
- Ferreira JC, Diniz-Silva F, Moriya HT, et al. Neurally Adjusted Ventilatory Assist (NAVA) or Pressure Support Ventilation (PSV) during spontaneous breathing trials in critically ill patients: a crossover trial. BMC Pulm Med 2017;17:139. [Crossref] [PubMed]
- Kuo NY, Tu ML, Hung TY, et al. A randomized clinical trial of neurally adjusted ventilatory assist versus conventional weaning mode in patients with COPD and prolonged mechanical ventilation. Int J Chron Obstruct Pulmon Dis 2016;11:945-51. [Crossref] [PubMed]
- Piquilloud L, Tassaux D, Bialais E, et al. Neurally adjusted ventilatory assist (NAVA) improves patient-ventilator interaction during non-invasive ventilation delivered by face mask. Intensive Care Med 2012;38:1624-31. [Crossref] [PubMed]
- Schmidt M, Dres M, Raux M, et al. Neurally adjusted ventilatory assist improves patient-ventilator interaction during postextubation prophylactic noninvasive ventilation. Crit Care Med 2012;40:1738-44. [Crossref] [PubMed]
- Schmidt M, Kindler F, Cecchini J, et al. Neurally adjusted ventilatory assist and proportional assist ventilation both improve patient-ventilator interaction. Crit Care 2015;19:56. [Crossref] [PubMed]
- Patroniti N, Bellani G, Saccavino E, et al. Respiratory pattern during neurally adjusted ventilatory assist in acute respiratory failure patients. Intensive Care Med 2012;38:230-9. [Crossref] [PubMed]
- Mauri T, Bellani G, Grasselli G, et al. Patient-ventilator interaction in ARDS patients with extremely low compliance undergoing ECMO: a novel approach based on diaphragm electrical activity. Intensive Care Med 2013;39:282-91. [Crossref] [PubMed]
- Yonis H, Crognier L, Conil JM, et al. Patient-ventilator synchrony in Neurally Adjusted Ventilatory Assist (NAVA) and Pressure Support Ventilation (PSV): a prospective observational study. BMC Anesthesiol 2015;15:117. [Crossref] [PubMed]
- Murias G, Lucangelo U, Blanch L. Patient-ventilator asynchrony. Curr Opin Crit Care 2016;22:53-9. [Crossref] [PubMed]
- Kondili E, Prinianakis G, Georgopoulos D. Patient-ventilator interaction. Br J Anaesth 2003;91:106-19. [Crossref] [PubMed]
- Parthasarathy S, Jubran A, Tobin MJ. Cycling of inspiratory and expiratory muscle groups with the ventilator in airflow limitation. Am J Respir Crit Care Med 1998;158:1471-8. [Crossref] [PubMed]
- Leung P, Jubran A, Tobin MJ. Comparison of assisted ventilator modes on triggering, patient effort, and dyspnea. Am J Respir Crit Care Med 1997;155:1940-8. [Crossref] [PubMed]
- Tokioka H, Tanaka T, Ishizu T, et al. The effect of breath termination criterion on breathing patterns and the work of breathing during pressure support ventilation. Anesth Analg 2001;92:161-5. [Crossref] [PubMed]
- Imanaka H, Nishimura M, Takeuchi M, et al. Autotriggering caused by cardiogenic oscillation during flow-triggered mechanical ventilation. Crit Care Med 2000;28:402-7. [Crossref] [PubMed]
- Baudin F, Pouyau R, Cour-Andlauer F, et al. Neurally adjusted ventilator assist (NAVA) reduces asynchrony during non-invasive ventilation for severe bronchiolitis. Pediatr Pulmonol 2015;50:1320-7. [Crossref] [PubMed]
- Vignaux L, Vargas F, Roeseler J, et al. Patient-ventilator asynchrony during non-invasive ventilation for acute respiratory failure: a multicenter study. Intensive Care Med 2009;35:840-6. [Crossref] [PubMed]
- Spahija J, de Marchie M, Albert M, et al. Patient-ventilator interaction during pressure support ventilation and neurally adjusted ventilatory assist. Crit Care Med 2010;38:518-26. [Crossref] [PubMed]
- Terzi N, Pelieu I, Guittet L, et al. Neurally adjusted ventilatory assist in patients recovering spontaneous breathing after acute respiratory distress syndrome: physiological evaluation. Crit Care Med 2010;38:1830-7. [Crossref] [PubMed]
- Beck J, Reilly M, Grasselli G, et al. Patient-ventilator interaction during neurally adjusted ventilatory assist in low birth weight infants. Pediatr Res 2009;65:663-8. [Crossref] [PubMed]
- Moerer O, Beck J, Brander L, et al. Subject-ventilator synchrony during neural versus pneumatically triggered non-invasive helmet ventilation. Intensive Care Med 2008;34:1615-23. [Crossref] [PubMed]
- Barwing J, Ambold M, Linden N, et al. Evaluation of the catheter positioning for neurally adjusted ventilatory assist. Intensive Care Med 2009;35:1809-14. [Crossref] [PubMed]
- Barwing J, Pedroni C, Quintel M, et al. Influence of body position, PEEP and intra-abdominal pressure on the catheter positioning for neurally adjusted ventilatory assist. Intensive Care Med 2011;37:2041-5. [Crossref] [PubMed]