Transplantation of umbilical cord mesenchymal stem cells into mice with focal segmental glomerulosclerosis delayed disease manifestation
Introduction
Focal segmental glomerulosclerosis (FSGS) is a common kidney disease, with proteinuria or nephrotic syndrome (NS) as the main manifestation. The characteristic pathological features are focal, segmental sclerosis in glomerluli and podocyte injury.
Our group collected 40 FSGS families, and whole-exome sequencing was performed on these familial FSGS (fFSGS) patients. COL4A3 mutations were identified in 5 (12.5%) FSGS families. We first discovered a novel mutation site in COL4A3 (C1616Y) (1). To the best of our knowledge, this mutation, which is located in the noncollagenous domain (NC1) of type IV collagen, is novel. The mutation in this region will affect the collagen IV α3, α4, and α5 chains, which form a trimer, causing kidney injury. Type IV collagen is one of the components of the extracellular matrix in the basement membrane. Abnormal expression, translocation or assembly of this molecule results in numerous nephropathic symptoms, such as Alport syndrome, thin basement membrane nephropathy (TBMN) and familial focal segmental glomerulosclerosis. (2).
Studies have found that glomerular and tubular damage in fFSGS is more severe than in sporadic FSGS patients (3). Currently, steroids and immunosuppressive therapy are not effective for fFSGS, and kidney transplantation is hindered by the shortage of available kidney donors, most of them progress into end stage renal disease (ESRD), which brings tremendous economic pressure and living burden to patients.
Stem cell therapy refers to intervening in the development of disease by introducing stem cells and improving the prognosis of patients. Stem cell therapy has made some progress in the study of type IV collagen-associated kidney disease in recent years (4,5). The mechanism by which stem cells act on the kidney is mainly a paracrine mechanism. The paracrine mechanism mainly regulates the secretion of a series of cytokines by stem cells to achieve immune regulation, anti-inflammatory, anti-fibrosis, anti-apoptosis and anti-oxidative stress. To date, stem cell therapy is one of the most promising approaches for treatment of fFSGS.
Methods
Animal model
The animal model used in this experiment was p.Gly1617Valfs X15 (C57BL/6) mutant mice. This mutation was first found in an FSGS family undergoing renal biopsy in our department. Four family members had the same mutation, C1616Y, in COL4A3 (1). Protein sequence alignment indicated the corresponding mutation to C1616Y in model mice, which was constructed by CRISPR/Cas9 genomic editing technology and named p.Gly1617Valfs X15. The animal model was housed in the SPF facility of the Experimental Animal Research Center of Ruijin Hospital, Shanghai Jiao Tong University School of Medicine. All animal experiments were performed in adherence to the laboratory animal welfare and ethics committee, which was approved by the Ethics Committee of Ruijin Hospital.
The experimental mice were grouped as follows: positive control group: mutant mice + saline; positive treatment group: mutant mouse + umbilical cord mesenchymal stem cells (UC-MSC); negative control group: wild type mice + saline, 13 mice per group.
All mice selected in the study were male mice, and the weight difference was less than 10%. All mice started the treatments at 8 weeks. One mouse was sacrificed in each group in week 12, 16, 20, 24 to get the kidney tissue.
Umbilical cord mesenchymal stem cells
UCMSCs were obtained from Shanghai Biological Specimen Bank-Cell Research Laboratory. The cells passed the ethical review and third-party purity testing. Umbilical cord tissue pieces were placed onto sterile and plastic Petri dishes at 37 °C for 4 hours, and then, the culture medium was added. When the cells were subcultured to the F3 generation, they were made into a suspension (concentration: 1×106/mL) for subsequent use.
Stem cell staining and in vivo tracking
To track the stem cells in animals, we stained stem cells in vitro with PKH-26 cell fluorescent dyeing agent (Sigma MINI26-1KT). 2×10–6 M of PKH26 (2 mL) and 1×107 cells/mL (2 mL) were configured as a 4 mL mixture, incubated for 5 minutes at 25 °C. Discard the supernatant after centrifugation. Then added normal saline to make 1×106 /mL concentration cell suspension, followed by observation under a fluorescence microscope. For in vivo tracking, sacrificed mouse kidneys were cryosectioned and then observed under a fluorescence microscope.
Cell transplantation
The UCMSCs were prestained with PKH26. They UCMSCs (1×106/mL) were then injected into the tail veins of the mice. For the control group, saline was injected instead. The numbers of the stem cells injected were determined by the body weight of the mouse: 5×104 cells/g mouse. Total amount of stem cells injection was divided in three times, the injection was conducted once a week, consecutively for three weeks.
Serum creatinine analysis
After eyelid vein blood collection, the blood samples were allowed to stand at room temperature for 1 hour, they were centrifuged at 3,500 rpm for 7 minutes, and the supernatant serums were extracted and stored at −80 °C until use.
Measurement of proteinuria
Urine specimens were collected from the experimental mice with metabolic cages at week 8, week 12, week 16, week 20, week 24, and week 28. Urine specimens were collected and recorded 24 hours later. Urine samples were used to quantify 24 urine proteins.
Histology
Fresh kidneys were fixed in 2.5% glutaraldehyde fixative for electron microscopy and in 4% paraformaldehyde, embedded in paraffin, and washed for light microscopy, immunofluorescence and immunohistochemistry. Observation by HE staining was performed under a light microscope. For immunofluorescence, 4-mm sections were air dried (2 h), fixed in acetone (10 min, 4 °C), air dried for a further 2 h, and denatured (1 h, 4 °C) using a solution of 6 M urea and 0.1 M glycine in PBS. Slides were then washed with PBS and incubated overnight with a Col4a3 primary antibody (NOVUS NBP1-55798) and slides were then washed in PBS, incubated at room temperature for 1 h with goat anti-rabbit secondary antibody (DAKO). Immunohistochemistry staining for the iL-6, iL-22, TNF-α was performed on periodate-lysine-paraformaldehyde (PLP)-fixed kidney cryostat sections (4 mm). Sections were incubated overnight at 4 °C with primary antibody (Servicebio). Sections were then washed with PBS, incubated with the appropriate horseradish peroxidase (HRP)-conjugated secondary antibody (Servicebio), washed with PBS, and visualized using DAB.
Statistical analyses
Statistical analyses were performed using IBM SPSS statistical package 24. Data are expressed as the mean ± standard deviation, and differences between groups were tested by one-way ANOVA. Survival analysis data were analyzed by Kaplan-Meier plots, and differences were compared with the log-rank test. In this study, P<0.05 was considered statistically significant.
Results
Phenotype of animal model
According to the novel mutation site found in our FSGS family, we established a novel animal model of FSGS. Our homogeneous (Hom) mouse model appeared to have proteinuria starting at week 4, then gradually grow, reached 29 mg/24 h at week 24. At week 28, the proteinuria decreased significantly, possibly because the mice reached the ESRD stage, the urine volume of these mice was less than 0.2 mL at 28 weeks (Figure 1A). Increased creatinine was observed at week 12, reached 62 µmol/L at week 28 (Figure 1B). Most deaths occurred before week 28, and no mice survived for more than 32 weeks. A large amount of glomerular sclerosis (76%) and atrophy (50%) can be observed in renal tissue at week 24 (Figure 1C). After verification, this FSGS animal model produced proteinuria and typical focal segmental sclerosis in glomeruli, which were consistent with the clinicopathological features of FSGS.
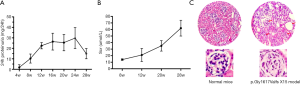
UCMSCs resided in the kidney
UCMSCs were first stained with PKH26 before being injected into the model mice via tail veins. To determine if they moved to the kidney, the mice were sacrificed 48 hours after injection, followed by the separation of kidney tissues for cryosections. Under fluorescent microscopy, we found many red fluorescent cells in the kidney tissue, indicating the presence of stem cells in the kidney (Figure 2). Moreover, trypan blue staining proved that their survival rate was >95%.
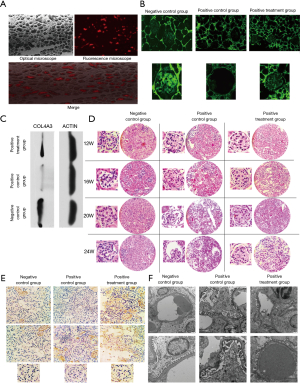
The transplantation of the UCMSCs led to a decrease in urine protein
The three groups of mice received either UCMSCs or saline treatment at week 8. Their 24 h urine samples were then collected and subjected to the quantification of urine protein consecutively at weeks 8, 10, 12, 16, 20, 24 and 28. A significant decrease in urine protein was observed in the treated group compared with the positive control group. However, significant proteinuria still existed compared with that of the negative control group (Figure 3). The reduction in urinary protein was significant starting at week 4 after the UCMSC transplantation, and the protein level rose again by week 16. Urinary protein is one of the most important hallmarks in the evaluation of kidney function. Compared with the positive control group, the improvement in urinary protein released in the treatment group indicated that stem cell therapy might delay the progression of Col4a3 mutation-induced renal functional damage.
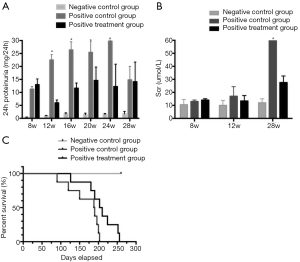
The effect of UCMSC transplantation on serum creatinine
Serum creatinine was 10.5, 13 and 14 μmol/L (baseline) for the negative control group, the positive control group, and the treatment group, respectively. After one month of treatment with either the stem cells or saline, these values were 10, 17, 13.4 μmol/L, respectively. At week 28, serum creatinine was 12.1, 60.6, and 27.5 μmol/L. There were no significant differences between the positive control group and the treatment group until week 12. However, at week 28, creatinine of the positive control group was significantly higher than that of the treatment group (Figure 3). This finding indicated that UCMSC transplantation helps alleviate the symptoms of this condition.
Phenotypic differences among the three groups under optical microscopic observation
Under optical microscopy, we found that the glomerular sclerosis and atrophy of the positive treatment group were significantly improved after the UCMSC treatment, but the glomeruli still showed segmental sclerosis changes compared with the negative control group. In the positive control group, significant sclerosis and fibrotic changes began to appear in the glomeruli at week 16 [glomerular sclerosis ratio (GSR): 28%, atrophy ratio (AR): 0%]. At week 20, some glomeruli showed sclerosis and atrophy, and inflammatory cell infiltration was observed in the renal interstitial region (GSR: 46%, AR: 20%). At week 24, glomerular sclerosis atrophy was observed, and some glomeruli disappeared (GSR: 75%, AR: 52%). In contrast, in the treated mice, sclerosis ratio observed in the glomeruli at W16, W20, and W24 (GSR: 18%, 30%, 45%) was lower, and the AR at W16, W20, W24 (AR: 0%, 8%, 25%) was lower. Significant differences were observed among the three groups under optical microscopy at each time point (Figure 2).
Immunofluorescence confirms the expression of the type IV collagen α3 chain, which partially compensated for renal functional injury in Col4a3 mutant mice
The onset of kidney injury in our model mice is mainly due to the abnormal or insufficient secretion of the type IV collagen α3 chain resulting from the Col4a3 mutation. Consequently, this change leads to abnormal structure of the basement membrane and podocytes, and the normal filtration function of the glomeruli is affected. Therefore, analysis of the secretion and distribution of the type IV collagen α3 chain after UCMSC treatment is important. Anti-mouse Col4α3 (NOVUS) was used as the primary antibody for immunofluorescence detection. Kidneys of three groups of mice were randomly taken at week 20. By immunofluorescence, we clearly observed that the type IV collagen α3 chain of the negative control group was normal and distributed along the basement membrane, while in the kidney from the positive control mice, the secretion of type IV collagen α3 chain was significantly reduced, and the lack of the type IV collagen α3 chain significantly affected the formation of the basement membrane and the filtration function of the glomerulus. In the kidney from the positive treatment group, the type IV collagen α3 chain in the glomeruli was obvious under immunofluorescence microscopy and showed significantly higher levels than the positive control group but did not reach to the level of the negative control group (Figure 2). By Western blot, we verified the expression of COL4A3 protein (Figure 2), and the gray value (COL4A3/Actin) of three groups were 0.45 (negative control group), 0.1 (positive control group), 0.25 (positive treatment group). Therefore, the Col4a3 mutant mice treated with UCMSCs showed increased levels of type IV collagen α3 chain secretion.
IL-22 expression was significantly increased in the mice of the treatment group
Tumor necrosis factor α (TNFα), interleukin-6 (iL-6) and interleukin 22 (iL-22) of the three groups of mice were examined by immunohistochemistry at week 20. TNFα and iL-6 were thought to be related to glomerular sclerosis, while iL-22 was recently shown to play an important role in post-injury repairmen (6). We did not find differences in the expression of TNFα and iL-6 in the three groups. However, we observed that the expression of iL-22 in the positive treatment group was significantly increased, while the negative control group and the positive control group did not show significant expression of iL-22. In the renal immunohistochemistry of the positive treatment group, iL-22 was mainly expressed in the renal interstitial region, and partial distribution was also observed in the glomerulus (Figure 2).
Phenotypic recovery could be observed under transmission electron microscopy
Abnormal or insufficient secretion of type IV collagen α3 leads to abnormal basement membrane and podocyte structures. This change results in morphological changes in the basement membrane and podocytes, which can be clearly observed under transmission electron microscopy. At week 20, the three groups of mice were randomly sacrificed, and their kidney tissues were taken for transmission electron microscopy. In the negative control group, the thickness of the basement membrane was uniform, the podocytic process was neatly arranged, the morphology of the podocytes was normal, and no fusion or detachment of the podocytic process was observed under the electron microscope, which was consistent with the morphology of the normal mice under electron microscopy. The ultrastructure of the positive control group was very irregular. The basement membranes showed uneven thicknesses, and the dense layer of basement membrane showed tear-like changes. Moreover, the podocytic process morphology was abnormal, and a many foot processes showed fusion, atrophy and detachment, which was a typical collagen IV-related nephropathy-like change. In the positive treatment group, the basement membrane was slightly uneven under electron microscopy, which was less severe than the positive control. There were no tear-like changes in the basement membrane dense layer. The podocytic process was slightly disordered, and low levels of podocytic process fusion were observed. No significant podocyte atrophy and detachment was observed. Compared with the images of light microscopy, significant differences in the ultrastructure of glomeruli among the three groups were found (Figure 2). The ultrastructure of the treatment group was close to that of the negative control group under electron microscopy.
USMSC transplantation prolongs the lifespan of the FSGS model mice
The negative control group animals did not die throughout the experiments, while the positive control group animals all died before week 29. Compared with the positive control group, the survival rate of the positive treatment group was improved, and the longest survival time was 36 weeks (Figure 3). However, a significant difference was not found between the positive treatment group and the positive control group (P=0.06), nor were UCMSC transplantation-related side effects observed in any of the mice.
Discussion
Many animal models of type IV collagen-associated kidney disease have been used to illustrate the pathogenesis of the disease while providing an opportunity for different therapeutic approaches; most studies focus on gene or cell therapy. These models include Samoyed dog model (7), an Alport syndrome Navasota dog model (8) by X-linked dominant inheritance, or a British dog Alport syndrome colon model (9); a Col4a3-/- mouse Alport syndrome model (10); a tg/tg mouse Alport syndrome model (11) by Col4α3/4 autosomal recessive mutation, a bulldog Alport syndrome animal model by autosomal dominant inheritance (12), and a Col4a3+/- mouse TBMN animal model (13). Different mutation sites on the COL4A3 gene may cause different diseases, such as Alport syndrome, TBMN, and benign familial hematuria (BFH). The COL4aA3 mutant in FSGS was recently discovered. In our research, we constructed a novel FSGS animal model according to the novel mutation sites found in our fFSGS patients. These animal models showed the clinicopathological features of FSGS. The survival time of these animal models was shorter than that of humans, allowing for the observation of multiple generations and multiple bodies in one trial (14).
In contrast to the animal models previously established, our mutation is located in the NCI region, and this abnormality not only affects the col4α3 chain but also affects the structure and function of the entire α3α4α5 trimer and thus exhibits a more severe phenotype. Our preliminary work demonstrated that the p.Gly1617Valfs X15 mouse model showed abnormal urinary protein at week 4, which continued to increase, and most mice showed a shortened lifespan before week 28. The mice have a hereditary disease, which causes insufficient secretion of type IV collagen, leading to abnormal formation of the basement membrane and podocytes. Early intervention would lead to better results. Therefore, we chose to start the UCMSC treatment at week 8. Simultaneously, we also injected UCMSCs into 8 wild-type mice, and no elevation of urinary protein and serum creatinine was observed compared with the negative control group, with no significant adverse effects.
Our study showed that UCMSC treatment can effectively reduce proteinuria for 4 weeks, although it began to rise again at week 16. Further experiments are needed to assess whether a boost of additional UCMSC treatment at week 16 is necessary to enhance these effects. At week 28, the proteinuria of the positive control group decreased significantly, possibly because the mice in this group reached the ESRD stage at 28 weeks, when severe glomerulosclerosis and poor renal filtration function may lead to a significant decrease in urinary protein; the urine volume of these mice was less than 0.2 mL at 28 weeks.
HE staining outcomes showed that although stem cell therapy cannot reverse the course of the mutant mice, it can indeed effectively delay the progression of glomerular sclerosis and atrophy compared with the positive control group. Immunofluorescence results demonstrated that stem cell therapy can effectively increase the secretion of normal type IV collagen α3 chain in glomeruli. Type IV collagen is intertwined by α3, α4, and α5 to form the an α3α4α5 trimer, which is necessary for a strong basement membrane. Therefore, the Col4a3 mutation would cause abnormalities in the basement membrane. In the positive treatment group, the expression level of the α3 chain of type IV collagen was more abundant than that of the treatment group. The immunofluorescence results further indicated that UCMSC therapy can specifically increase the secretion of the type IV collagen α3 chain to help repair the basement membrane, protect the podocytes, delay the progression of the disease, and improve the prognosis.
In addition, the electron microscopy images further confirmed the immunofluorescence results, in which the UCMSCs effectively improved the morphology of the basement membrane and podocytes in the positive treatment group. Renal pathological changes caused by type IV collagen mutations are often not obvious under light microscopy, but under electron microscopy, changes in the thickness of the basement membrane and abnormal morphology of the foot process can be easily observed.
Stem cell function is currently believed to involve two main pathways: one is through the paracrine pathway, and the other is through stem cells differentiation into damaged cells to replace their functions (15). The paracrine pathway is well recognized and mainly involves the regulation of a number of cytokines to improve renal function, and whether stem cells can differentiate into damaged cells is still in dispute. In our study, we found that iL-22 showed a significant increase after stem cell therapy. A recent article in Nature Review found that iL-22 can protect and repair the whole body’s organs, while stem cells can also protect and repair many organs (6). Stem cells may repair the organ after injury through iL-22. Therefore, we performed immunohistochemistry to detect the expression of iL-22 in the experimental mice, which showed enhanced expression. IL-22 functions mainly by acting on epithelial cells of various organs. The main mechanisms include inducing the production of specific chemokines, helping to enhance cell proliferation, promoting cellular mobility, etc. (6). Epithelial cells are widely distributed in the kidney, including glomerular podocytes and renal tubular epithelial cells, which are important for kidney function. IL-22 regulation of epithelial cells may also have protective effects on the kidney. IL-22 promotes podocyte proliferation and repairs podocytes when they are damaged (16). Korean scholars have found that upregulation of iL-22 can significantly inhibit the progression of IgA nephropathy (17).
The paracrine secretion mechanism of stem cells is mainly mediated through the secretion of a number of cytokines to promote immune cell activity, immune regulation, antifibrotic effects, antiapoptotic effects or oxidative stress (18). The mechanism of iL-22-mediated protection of epithelial cells also includes antifibrotic effects, antiapoptotic effects and oxidative stress (19). This protective mechanism is consistent with the functions of stem cells and iL-22. Stem cells can regulate immune cell activity, while iL-22 is mainly secreted by immune cells, such as group 3 innate-like lymphocytes (ILC3s) and T helper cells (20). The main target of iL-22 is epithelial cells and stem cells (21,22). Stem cells promote the production of iL-22, and iL-22 protects the tissue through stem cells. Therefore, they both complement each other.
In this study, we showed that UCMSC transplantation may have the potential to treat FSGS. IL-22 may play an important role in this process. Further studies are needed to illustrate the underlying mechanism.
Acknowledgments
Funding: The work was supported by the National Natural Science foundation of China (81570598, 81870460) and Shanghai Pujiang program (17PJ1405900).
Footnote
Conflicts of Interest: The authors have no conflicts of interest to declare.
Ethical Statement: All animal experiments were performed in adherence to the laboratory animal welfare and ethics committee, which was approved by the Ethics Committee of Ruijin Hospital. The authors are accountable for all aspects of the work in ensuring that questions related to the accuracy or integrity of any part of the work are appropriately investigated and resolved.
References
- Xie J, Wu X, Ren H, et al. COL4A3 mutations cause focal segmental glomerulosclerosis. J Mol Cell Biol 2014;6:498-505. [Crossref] [PubMed]
- Heikkila P, Parpala T, Lukkarinen O, et al. Adenovirus-mediated gene transfer into kidney glomeruli using an ex vivo and in vivo kidney perfusion system - first steps towards gene therapy of Alport syndrome. Gene Ther 1996;3:21-7. [PubMed]
- Hao X, Xie J, Ma J, et al. Increased risk of treatment failure and end-stage renal disease in familial focal segmental glomerular sclerosis. Contrib Nephrol 2013;181:101-8. [Crossref] [PubMed]
- LeBleu V, Sugimoto H, Mundel TM, et al. Stem cell therapies benefit Alport syndrome. J Am Soc Nephrol 2009;20:2359-70. [Crossref] [PubMed]
- Moschidou D, Corcelli M, Hau KL, et al. Human Chorionic Stem Cells: Podocyte Differentiation and Potential for the Treatment of Alport Syndrome. Stem Cells Dev 2016;25:395-404. [Crossref] [PubMed]
- Sabat R, Ouyang W, Wolk K. Therapeutic opportunities of the IL-22–IL-22R1 system. Nature Reviews Drug Discovery 2014;13:21-38. [Crossref] [PubMed]
- Jansen B, Thorner P, Baumal R, et al. Samoyed hereditary glomerulopathy (SHG). Evolution of splitting of glomerular capillary basement membranes. Am J Pathol 1986;125:536-45. [PubMed]
- Benali SL, Lees GE, Nabity MB, et al. X-Linked Hereditary Nephropathy in Navasota Dogs: Clinical Pathology, Morphology, and Gene Expression During Disease Progression. Vet Pathol 2016;53:803-12. [Crossref] [PubMed]
- Lees GE, Wilson PD, Helman RG, et al. Glomerular ultrastructural findings similar to hereditary nephritis in 4 English cocker spaniels. J Vet Intern Med 1997;11:80-5. [Crossref] [PubMed]
- Cosgrove D, Meehan DT, Grunkemeyer JA, et al. Collagen COL4A3 knockout: a mouse model for autosomal Alport syndrome. Genes Dev 1996;10:2981-92. [Crossref] [PubMed]
- Lu W, Phillips CL, Killen PD, et al. Insertional mutation of the collagen genes Col4a3 and Col4a4 in a mouse model of Alport syndrome. Genomics 1999;61:113-24. [Crossref] [PubMed]
- Hood JC, Savige J, Hendtlass A, et al. Bull terrier hereditary nephritis: a model for autosomal dominant Alport syndrome. Kidney Int 1995;47:758-65. [Crossref] [PubMed]
- Beirowski B, Weber M, Gross O. Chronic renal failure and shortened lifespan in COL4A3+/- mice: an animal model for thin basement membrane nephropathy. J Am Soc Nephrol 2006;17:1986-94. [Crossref] [PubMed]
- Heikkilä P, Tryggvason K, Thorner P. Animal models of Alport syndrome: advancing the prospects for effective human gene therapy. Exp Nephrol 2000;8:1-7. [Crossref] [PubMed]
- Sugimoto H, Mundel TM, Sund M, et al. Bone-marrow-derived stem cells repair basement membrane collagen defects and reverse genetic kidney disease. Proc Natl Acad Sci U S A 2006;103:7321-6. [Crossref] [PubMed]
- Weidenbusch M, Rodler S, Anders HJ. Interleukin-22 in kidney injury and regeneration. Am J Physiol Renal Physiol 2015;308:F1041-6. [Crossref] [PubMed]
- Suh JS, Cho SH, Chung JH, et al. A polymorphism of interleukin-22 receptor alpha-1 is associated with the development of childhood IgA nephropathy. J Interferon Cytokine Res 2013;33:571-7. [Crossref] [PubMed]
- Bi B, Schmitt R, Israilova M, et al. Stromal cells protect against acute tubular injury via an endocrine effect. J Am Soc Nephrol 2007;18:2486-96. [Crossref] [PubMed]
- Sonnenberg GF, Fouser LA, Artis D. Border patrol: regulation of immunity, inflammation and tissue homeostasis at barrier surfaces by IL-22. Nat Immunol 2011;12:383-90. [Crossref] [PubMed]
- Eyerich S, Eyerich K, Cavani A, et al. IL-17 and IL-22: siblings, not twins. Trends Immunol 2010;31:354-61. [Crossref] [PubMed]
- Hanash AM, Dudakov JA, Hua G, et al. Interleukin-22 Protects Intestinal Stem Cells from Immune-Mediated Tissue Damage and Regulates Sensitivity to Graft versus Host Disease. Immunity 2012;37:339-50. [Crossref] [PubMed]
- Feng D, Kong X, Weng H, et al. Interleukin-22 promotes proliferation of liver stem/progenitor cells in mice and patients with chronic hepatitis B virus infection. Gastroenterology 2012;143:188-98.e7. [Crossref] [PubMed]