Portal pressure monitoring—state-of-the-art and future perspective
Introduction
Portal hypertension (PH), a severe symptom of chronic liver diseases, is defined as a pathologically increased portal pressure gradient (PPG) greater than 5 mmHg (1). Measuring the hepatic perfusion pressure, PPG is the difference between the portal vein pressure (PVP) and hepatic vein pressure or inferior vena cava (IVC) pressures. It is caused by the interaction of the portal blood flow with the vascular resistance against the flow (2). The relationship can be given by Ohm’s law as follows:
Based on Ohm’s law, the increased PVP is attributed to an increased portal vein blood flow, an increased vascular resistance, or a combination of both (3). Based on the anatomical location of the increased resistance to blood flow within liver circulation, PH can be classified into three types: pre-hepatic PH, intra-hepatic PH, and post-hepatic PH (Table 1). Among the aforementioned diseases related to PH liver cirrhosis is the leading cause of PH worldwide. Other less common causes of PH account for less than 10% of cases, mostly due to vascular liver diseases such as extrahepatic portal vein occlusion (Budd-Chiari Syndrome) and idiopathic PH (4,5).
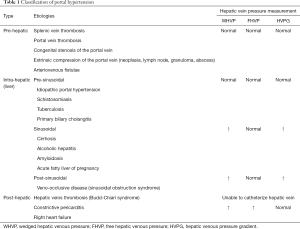
Full table
The normal range of PPG is 1–5 mmHg. When the value of PPG is in the range of 5–9 mmHg, it represents subclinical PH (6). However, when PPG increases to ≥10 mmHg, PH complications are more likely to occur, such as the formation of varices related to upper digestive bleeding, ascites, infection, hepatic encephalopathy, or renal failure (7,8). As the most common cause of PH, liver cirrhosis is a significant cause of global health burden. It is the eleventh-leading cause of adult deaths worldwide, and it results in 1.16 million deaths per year (9). PH complication may result in the need for liver transplantation, and it may also lead to death in patients with cirrhosis (10,11). Approximately 80–90% of asymptomatic PH patients already have an increased PPG [assessed clinically as an alternative indicator easy to obtain, namely the hepatic venous pressure gradient (HVPG)], and endoscopy has shown that 40% of patients already have gastroesophageal varices (6). According to the HVPG threshold, patients with PH could be classified into two levels: mild PH (HVPG >5 but <10 mmHg) and clinically significant PH (CSPH) (HVPG ≥10 mmHg) (1). For cirrhosis patients, CSPH further predicts the development of the decompensation of cirrhosis (12), higher risk of postsurgical decompensation, and increased risk of liver cancer (13,14). Based on the evidence, HVPG >12 mmHg indicates bleeding risk among CSPH patients with gastroesophageal varices (15). For, HVPG >16 mmHg, a higher risk of death is predicted (16). HVPG >20 mmHg is an independent prognostic factor of treatment failure and mortality (17,18). For cirrhosis patients waiting for liver transplantation, each 1-mmHg elevation in HVPG increases the risk of death by 3% during a median follow-up of 19 months (19). Therefore, measuring and monitoring the portal pressure (PPG or its approximate value HVPG) in PH patients is important to guide the clinical treatment and evaluate the treatment efficacy.
The absolute portal pressure can be affected by intraabdominal pressure, which can be risen by obesity and ascites. However, increased intraabdominal pressure can increase both the portal vein and IVC pressures, without altering the value of PPG (20). As a result, compared with absolute portal pressure, it is clinically more meaningful to represent hepatic perfusion pressure in terms of PPG. The portal pressure can be directly acquired from transhepatic or transvenous catheterization of hepatic portal vein. However, the IVC pressure is also required to catheterize to determine the PPG. Considering the high risk of intraperitoneal bleeding and the requirement of significant procedural experience, HVPG measurement, a gold-standard technique to assess PH, has replaced other invasive methods of measuring PPG in cirrhosis since 1951 (1,21). In spite of its safety and reproducibility, it was still invasive, relatively expensive, and only available in experienced constitutions. As a result, over the past decade, noninvasive methods for assessing PH have been increasingly investigated (22).
In this study, we focus on both invasive and noninvasive methods to monitor the portal pressure and explored their roles in clinical setting. The advantages and limitations of various techniques for future research are also inquired.
Invasive methods for assessing portal pressure—measurement of HVPG
The catheterization of hepatic vein with the measurement of HVPG is the gold-standard method of monitoring the portal pressure in cirrhosis (sinusoidal PH) (1). HVPG is defined as the difference between the wedged hepatic venous pressure (WHVP) and the free hepatic venous pressure (FHVP) (23).
In 1951, Myers and Taylor first reported WHVP, the pressure of the hepatic sinusoid, an indirect measure of PVP (21). The WHVP is obtained by blocking the hepatic vein to form a static column of blood, which represents the pressure of hepatic sinusoids. This has been demonstrated for cirrhosis attributed to alcohol, viral infection (hepatitis B virus and hepatitis C virus), and nonalcoholic steatohepatitis (1,24). As in cirrhosis, the connections between sinusoids are lost owing to fibrosis and nodule and septa formation; the sinusoidal pressure equals to the portal pressure (25). The FHVP, as its name suggests, is measured by the pressure of the nonoccluded hepatic vein.
HVPG is measured by catheterizing the right jugular vein or femoral vein under local anesthesia. Then, a balloon-tipped catheter is inserted under fluoroscopic guidance into a hepatic vein to acquire the FHVP and WHVP (Figure 1). The FHVP is obtained by keeping the tip of the catheter floating freely in the hepatic vein, 2–4 cm from its opening into the IVC. Inflating the balloon, the WHVP is measured by occluding the hepatic vein (26). The doctors connect the pressure sensor to the catheter and read the pressure value from the detector. The WHVP and FHVP should be measured until a stable value is obtained. All measurements should be taken minimally twice. The final value is calculated as the mean of these measurements. Although the technique of acquiring the HVPG values is relatively straightforward, specialist training is still required to achieve accurate measurements.
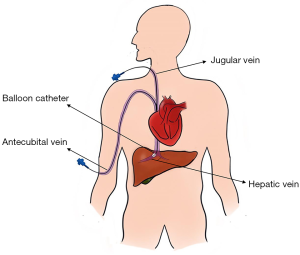
The value of HVPG is widely applied for the diagnosis of chronic liver disease, monitoring the development of PH, guiding clinical treatment, assessing new therapeutic agents, and survival prediction of cirrhosis patients (1). Based on the evidence from clinical trials, the HVPG is likely the most validated tool for assessing PH and cirrhosis prognosis (Table 2) (7,12-15,18,27-31). Compared with direct PPG measurement, the HVPG is easy, safe, and less invasive. However, it should be noted that the WHVP (and, consequently, the HVPG) represents liver sinusoidal pressure. Therefore, hardly does it provide useful data in prehepatic or presinusoidal PH (Table 1). Additionally, although the measurement of HVPG is less invasive, some complications such as hematoma, bleeding, bile leakage, arteriovenous fistulae, or Horner syndrome still occur in a small number of patients (26). In addition, this invasive method requires specific expertise and setting, which limit its applicability in nonteaching centers. As stated in the Baveno consensus conferences, noninvasive methods for assessing the PH are required (22). Patients would benefit from noninvasive methods that can provide similar information.
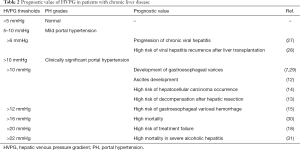
Full table
Noninvasive methods for assessing portal pressure
Although the measurement of HVPG is less invasive, some complications such as hematoma, bleeding, bile leakage, arteriovenous fistulae, or Horner syndrome still occur in a small number of patients (26). In addition, this invasive method requires specific expertise and setting, which limit its applicability in nonteaching centers. As stated in the Baveno consensus conferences, noninvasive methods for assessing the PH are required (22). Patients would benefit from noninvasive methods that provide similar information.
Serum markers
In recent years, several laboratory tests and serum markers have been able to evaluate the PH (32,33). Hayashi et al. directly measured PVPs in 40 patients and evaluated the association of PVP with the level of serum bile acid and the splenic volume. The equation for estimating PVP was given as follows: PVP = serum bile acid (µmol/L) ×2.593+ splenic volume (cm3) ×0.416+65.929 (r2=0.698) (33). Hsieh et al. investigated the correlations between noninvasive models (Table 3) and HVPG, including fibrosis-4 (FIB-4), aspartate aminotransferase to platelet ratio index, cirrhosis discriminant score, Lok index, Goteborg University Cirrhosis Index, and albumin-bilirubin (ALBI) score (34). They found that there was a weak correlation between all noninvasive markers and HVPG; the ALBI score had the best correlation (r=0.307, P<0.001) (34). Lim et al. evaluated the clinical implications of serum apelin (s-apelin), an endogenous ligand for angiotensin-like receptor 1, as a noninvasive prognostic role of chronic liver disease (35). The results showed that s-apelin was correlated with the measurement of HVPG (r2=0.356, P<0.001) (35). Procopet et al. assessed the diagnostic accuracy of six serum scores (Table 3) for diagnosing CSPH and found that the Lok score was the best with area under the receiver operating curve (AUROC) =0.84 (95% CI: 0.76–0.91, P<0.0001) (32). Thus, laboratory tests and serum markers show acceptable clinical utility, and many of them are not sufficiently accurate to diagnose PH and are not yet ready to be used in clinics.
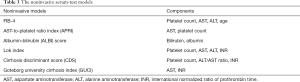
Full table
Radiological features—computed tomography (CT) and magnetic resonance imaging (MRI)
In a retrospective study, the score of liver surface nodularity quantified based on CT images was correlated with HVPG (r=0.75, P<0.001) (36). Based on a 385 patients-enrolled multi-institutional prospective diagnostic clinical trial (ClinicalTrials.gov identifier: NCT03138915), Liu et al. developed a radiomics-based model, and the results showed that HVPG exhibited good performance in detecting CSPH (C-index =0.849, 95% CI: 0.786–0.911). The sensitivity and specificity were 78.7% and 76.9%, respectively (37). Recently, the advances in the fields of three-dimensional modeling based on imaging and computational fluid dynamics analysis allow the noninvasive measurement of blood pressure (38,39). In a prospective multi-institutional study (ClinicalTrials.gov identifier: NCT02842697), Qi et al. developed a model to estimate the HVPG through CT angiographic images, termed virtual HVPG. The results showed that virtual HVPG correlated with invasive HVPG (r=0.61, P=0.001) (40). However, the interpretation of the virtual HVPG was relatively time consuming (about 2.5 hours for each case) in their study, and the number of patients without CSPH was small in their study. Therefore, the clinical usability of the virtual HVPG should be studied further.
Although some studies have shown that CT can predict HVPG, this technique has the disadvantage of requiring ionizing radiation. In a prospective study, MRI characteristics associated with both hepatic architecture and splanchnic hemodynamics were observed to be significantly correlated with HVPG. The liver T1 relaxation time and the velocity of splenic artery were also significantly correlated to HVPG (r=0.90, P<0.001) (41). Zhang et al. established a linear model to assess PVP based on the MR characteristics as follows: PVP (mmHg) =2.529+1.572× splenic venous diameter (mm) +0.231× splenic volume/body mass index (×104 cm5/kg) +3.44× aspartate aminotransferase-to-platelet ratio index (42). The model indicated accuracy in detecting PH with AUROC =0.945 (95% CI: 0.867–1.000), with sensitivity and specificity of 91.7% and 93.7% respectively (42). Chouhan et al. revealed that caval subtraction hepatic arterial fraction had a significant positive relationship with HVPG (r=0.780, P=0.014), based on the caval subtraction phase-contrast magnetic resonance imaging (PCMRI) procedure (43). However, the caval subtraction PCMRI is likely to be less successful in patients with abnormal hepatic venous outflows, such as the Budd-Chiari syndrome. In addition, a larger patient cohort is needed to further investigate whether this technique can be successfully used as a clinically practical alternative.
MR elastography (MRE) is a type of MRI technique that is based on quantitatively evaluating the mechanical characteristics of tissues through the propagation of shear waves (44). In a prospective cohort including 15 patients, the investigations showed that MRE was associated with HVPG (r2=0.377, P=0.02) (45). However, the size of the study was too small to draw reliable conclusions. Moreover, the MRE technique is only available at very few medical centers, and it is expensive compared to transient elastography (TE). The aforementioned limitation may therefore restrict its dissemination and application in clinical practice.
Ultrasound elastography (USE)
In the 1990s, Ophir et al. first described USE as an imaging technique, which is sensitive to tissue stiffness (46). In recent years, further studies have enabled quantitative assessments of liver, breast and spleen stiffness (47-50). Although the USE technique was originally applied to assess the hepatic fibrosis grade, some recent researches have expanded its use to assess potential liver function, such as PH, and risk of liver cancer development or liver failure (51-54). Based on the physical quantity measured, the USE technique can be classified into two types: strain imaging and shear wave imaging (SWI) (47). The SWI technique for measuring the Young’s modulus of tissue is mostly used in liver, breast and spleen stiffness examination. Currently, there are three technical methods for SWI: (I) TE, (II) two-dimensional shear wave elastography (2D SWE), and (III) point SWE (pSWE). Current studies in this field have revealed that USE may be a reliable noninvasive alternative to evaluate PH by measuring the liver stiffness (LSM) and/or spleen stiffness (SSM).
You et al. performed a meta-analysis included 11 studies to determine the relationship between liver stiffness measured using TE (TE-LSM) and HVPG. The results showed that TE-LSM significantly correlated with HVPG (r=0.783, 95% CI: 0.737–0.823) and represented good diagnostic performance for assessment of CSPH, with a sensitivity of 87.5% and specificity of 85.3% (55). However, the measurement results from TE can be affected by factors such as obesity and ascites, which may limit the application of the technique in advanced liver diseases (56). Compared with TE, measurement sites with the pSWE technique can be visualized with B-mode ultrasound. This advantage not only ensures LSM in the correct tissue site by avoiding ligament and vascular structures but also improves the accuracy of diagnosis (57). The results from a prospective cohort including 88 patients showed that pSWE values were correlated well with HVPG (r=0.646, P<0.001), and the sensitivity and specificity for pSWE were respectively 71.4% and 87.5% at the optimal cut-off (2.58 m/s). In the study, the TE performance was inaccurate in 22 patients (25%), whereas only one failure was noted for pSWE (0.9%) (58). The detection field of 2D SWE is larger than pSWE. It can show the measurement of elastography on a color display. Some studies have shown that, compared to TE, LSM by 2D SWE is a promising parameter to diagnose PH with higher success rate and better diagnostic value (59-61). The Baveno VI consensus meeting confirmed the role of TE-LSM in determining CSPH and PH (22). Moreover, SSM measured by USE has also been proven to be useful for assessing PH (57,62,63). The hemodynamic changes in PH can cause splenic congestion, which might induce splenic fibrosis and increased SSM. A meta-analysis including nine studies showed that SSM is strongly correlated with HVPG (r=0.72, 95% CI: 0.63–0.80) (64).
Some research studies have indicated that LSM is superior to SSM for diagnosing CSPH (61,65); however, the results of other studies provide contrary views (66,67). According to current literature, it is difficult to determine which one is the superior metric. Jansen et al. developed an algorithm to rule-in and rule-out CSPH based on LSM and SSM measured by 2D SWE (68). The accuracy of this algorithm was validated in a large cohort comprising 191 patients by Elkrief et al. (52). However, the results showed that these algorithms were not good enough to replace HVPG measurements or for use as the basis of clinical decision making.
Doppler ultrasonography (DUS)
DUS is a mainstay in the assessment of portal vein blood flow and hemodynamic changes (69,70). A prospective pilot study of 76 cirrhosis patients used DUS to assess the correlations between damping index (DI) of the hepatic vein waveforms and HVPG, and showed a relation between DI and HVPG (r2=0.468, P<0.001) (71). However, in another study including 138 cirrhosis patients, the investigators found that none of the DUS characteristics (such as the velocity of portal vein and the splenic vein, pulsatility, and resistive indices of the hepatic and splenic arteries) correlated with HVPG (72). The patient-related factors were observed to influence the DUS measurement values, such as respiration, timing of meals, and equipment; this might have contributed to the inconsistent results among the different studies (73). Considered together, the available data do not provide convincing results to recommend DUS measurements as a reliable alternative to HVPG (74).
Contrast-enhanced ultrasound (CEUS)
In recent years, the advancement of microbubble ultrasound contrast agents (UCAs), consisting of encapsulated microbubbles, and improvement of imaging technology have led to the possibility of using CEUS to evaluate hemodynamics and anatomical structures in real time (75). The microbubble is encapsulated with a stable shell of a lipid, albumin, or other material (76). High molecular weight and low solubility gases such as octafluoropropane or sulfur hexafluoride are filled in the core of the microbubble (Figure 2A,B). The microbubble UCAs act as nonlinear scatters of the incident ultrasound pulse, which can be distinguished from the linear response of the tissue, thus significant improving the contrast to tissue ratio by using nonlinear scattering signals from microbubbles. Since the UCAs were approved for imaging outside the heart by the Food and Drug Administration (FDA) in 2016 (76) (Table 4), the CEUS has been used in many applications, such as detecting liver tumors and evaluating the treatment response of chemotherapy (79,80). Currently, some studies have expanded the utility of CEUS to the assessment of PH (81-85).
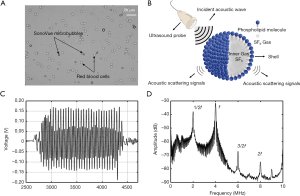
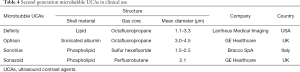
Full table
Transit time
Transit time, defined as the average time interval required for the bubbles to move from one vessel to another, is a representative parameter for microbubble hemodynamics (86). A study by Zhang et al. including 31 patients reported that the free portal pressure significantly correlated with hepatic-vein–hepatic-artery interval time (r=−0.900, P<0.001) and the portal-vein-hepatic-artery interval time (r=0.808, P<0.001) (Table 5) (82). However, the investigators applied the free portal pressure as standard reference. As mentioned previously, the free portal pressure is affected by intraabdominal pressure, surgery, and anesthesia. The hepatic vein arrival time (HVAT) is the average time taken for the UCA microbubbles to reach the hepatic vein after injection. Two studies revealed that HVAT had a significant correlation with HVPG and was accurate for detection of CSPH and severe PH (Table 5) (83,84). The splenic circulation measured by CEUS is also a promising predictor of PH. The peak enhancement time (PET), defined as the average time of the microbubble UCA from arriving at the splenic artery to reaching maximum intensity level in the splenic vein, was correlated with HVPG in a cohort of 91 patients (r=0.4573, P<0.0001) and provided a good rate of prediction of CSPH and severe PH (Table 5) (87). However, the transit time can be influenced by a poor echo window related to obesity, respiration, or changes to hepatic anatomy in patients with severe liver cirrhosis (83). Despite these promising data, in our opinion, transit time measurement with CEUS is more suitable for use as a supplementary or screening tool, rather than as a surrogate of HVPG.
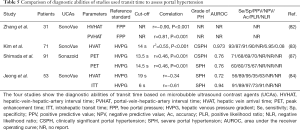
Full table
Subharmonic imaging—microbubble-based pressure sensors
Based on the compressible properties of gas-filled microbubble UCAs, their acoustic characteristics can change with the variations of blood pressure. Currently, three techniques are available to estimate the local blood pressure using microbubbles as sensors: based on the shift in resonance frequency of microbubbles (88-90), using the time of free bubbles disappearance as a measure of local blood pressure (91,92), and using the amplitude variations of the scattered echo of microbubbles (78,93-97). However, the first two approaches have high error rates, which prevent their preclinical or clinical applications. According to previous studies, the technique based on amplitude variation of the scattered echo is therefore the most promising option for clinical application (78,81,97,98).
The microbubbles act as nonlinear scatters of the incident ultrasound pulse and lead to echo response emissions consisting of fundamental (f), subharmonic (1/2f), second harmonic (2f), and ultraharmonic (3/2f) components (78) (Figure 2C,D). A study showed he subharmonic amplitude significantly correlated with ambient pressure (94). Moreover, the subharmonic signal generation can be classified into three stages—occurrence, growth, and saturation (Figure 3A) (96). The subharmonic amplitude decreased linearly with increasing hydrostatic pressure in the growth stage (78,93) (Figure 3B,C). As a consequence, Shi et al. proposed a technique using microbubbles as sensors to assess the changes of ambient pressure, called subharmonic-aided pressure estimation (SHAPE) (94). A pilot study measured the SHAPE image in both hepatic vein and portal vein (Figure 3D), and the results showed that SHAPE values correlated well with HVPG measurements in 45 patients (r=0.82) but had poor technical success rate (73%) (Figure 3E). In addition, SHAPE gradient strongly correlated with the HVPG in patients with severe PH (r=0.97); however, the sample size of their study was only six patients (81). Although SHAPE may be a useful clinical tool for monitoring PVPs in the future, there are still many difficulties to be addressed, including those on a case-by-case basis, depending on the physiological environment of the body and the differences that caused by attenuation, reverberation, and aberration through the abdominal wall. While this technique estimated local blood pressure using microbubbles as sensors, it still requires validation in larger cohorts.
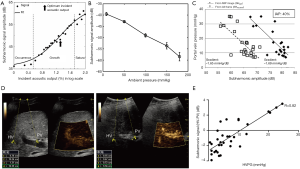
Conclusions
Liver disease account for a significant burden of disease and costs worldwide. As shown in the present review, although HVPG measurement is an invasive method to assess portal pressure and to evaluate the treatment response, it is still irreplaceable in clinical practice. In the past few decades, numerous noninvasive methods have shown promising results for monitoring portal pressure and assessing PH; however, challenges still remain in accurate evaluation. The cause of PH varies considerably: in Western and developed countries, alcoholic and nonalcoholic fatty liver disease related to cirrhosis is the major causes, whereas in Asian countries, viral hepatitis continues to be the primary cause. As a result, more prospective studies focused on different populations and disease etiologies are needed in the future. In addition, new evaluation techniques, such as 2D SWE, pSWE, and SHAPE can be used for consensus or guidelines, which are needed for investigators to interpret test results consistently. In recent years, some new technologies have emerged, such as radiomics and artificial intelligence. They are more likely to bring precision medicine closer to PH patients. Although the road to noninvasive measurement of portal pressure is still long, noninvasive methods hold great potential for a great variety of clinical applications, and further development may lead to their widespread clinical adoption in the future.
Acknowledgments
We thank Yunzhu Li, MD. of Peking Union Medical College (PUMC) Hospital for design and modification of the figures.
Funding: This work was funded by the CAMS Innovation Fund for Medical Sciences (CIFMS) (Grant No. 2016-I2M-1-001), National Natural Science Foundation of China (Grant No. 31500818, 11774369, 81671698, 81571689) and Shenzhen key laboratory of ultrasound imaging and therapy (Grant No. ZDSYS20180206180631473).
Footnote
Conflicts of Interest: The authors have no conflicts of interest to declare.
Ethical Statement: The authors are accountable for all aspects of the work in ensuring that questions related to the accuracy or integrity of any part of the work are appropriately investigated and resolved.
References
- Garcia-Tsao G, Abraldes JG, Berzigotti A, et al. Portal hypertensive bleeding in cirrhosis: Risk stratification, diagnosis, and management: 2016 practice guidance by the American Association for the study of liver diseases. Hepatology 2017;65:310-35. [Crossref] [PubMed]
- Bosch J, Pizcueta P, Feu F, et al. Pathophysiology of portal hypertension. Gastroenterol Clin North Am 1992;21:1-14. [PubMed]
- Bosch J, Garcia-Pagan JC. Complications of cirrhosis. I. Portal hypertension. J Hepatol 2000;32:141-56. [Crossref] [PubMed]
- Bosch J, Iwakiri Y. The portal hypertension syndrome: etiology, classification, relevance, and animal models. Hepatol Int 2018;12:1-10. [Crossref] [PubMed]
- Valla D. Splanchnic Vein Thrombosis. Semin Thromb Hemost 2015;41:494-502. [Crossref] [PubMed]
- Berzigotti A, Seijo S, Reverter E, et al. Assessing portal hypertension in liver diseases. Expert Rev Gastroenterol Hepatol 2013;7:141-55. [Crossref] [PubMed]
- Garcia-Tsao G, Groszmann RJ, Fisher RL, et al. Portal pressure, presence of gastroesophageal varices and variceal bleeding. Hepatology 1985;5:419-24. [Crossref] [PubMed]
- Groszmann RJ, Bosch J, Grace ND, et al. Hemodynamic events in a prospective randomized trial of propranolol versus placebo in the prevention of a first variceal hemorrhage. Gastroenterology 1990;99:1401-7. [Crossref] [PubMed]
- Asrani SK, Devarbhavi H, Eaton J, et al. Burden of liver diseases in the world. J Hepatol 2019;70:151-71. [Crossref] [PubMed]
- Tsochatzis EA, Bosch J, Burroughs AK. Liver cirrhosis. Lancet 2014;383:1749-61. [Crossref] [PubMed]
- Viganò L, Lleo A, Aghemo A. Non-alcoholic fatty liver disease, non-alcoholic steatohepatitis, metabolic syndrome and hepatocellular carcinoma-a composite scenario. Hepatobiliary Surg Nutr 2018;7:130-3. [Crossref] [PubMed]
- Ripoll C, Groszmann R, Garcia-Tsao G, et al. Hepatic venous pressure gradient predicts clinical decompensation in patients with compensated cirrhosis. Gastroenterology 2007;133:481-8. [Crossref] [PubMed]
- Berzigotti A, Reig M, Abraldes JG, et al. Portal hypertension and the outcome of surgery for hepatocellular carcinoma in compensated cirrhosis: a systematic review and meta-analysis. Hepatology 2015;61:526-36. [Crossref] [PubMed]
- Ripoll C, Groszmann RJ, Garcia-Tsao G, et al. Hepatic venous pressure gradient predicts development of hepatocellular carcinoma independently of severity of cirrhosis. J Hepatol 2009;50:923-8. [Crossref] [PubMed]
- Bosch J, Garcia-Pagan JC. Prevention of variceal rebleeding. Lancet 2003;361:952-4. [Crossref] [PubMed]
- Merkel C, Bolognesi M, Sacerdoti D, et al. The hemodynamic response to medical treatment of portal hypertension as a predictor of clinical effectiveness in the primary prophylaxis of variceal bleeding in cirrhosis. Hepatology 2000;32:930-4. [Crossref] [PubMed]
- Moitinho E, Escorsell A, Bandi JC, et al. Prognostic value of early measurements of portal pressure in acute variceal bleeding. Gastroenterology 1999;117:626-31. [Crossref] [PubMed]
- Abraldes JG, Villanueva C, Banares R, et al. Hepatic venous pressure gradient and prognosis in patients with acute variceal bleeding treated with pharmacologic and endoscopic therapy. J Hepatol 2008;48:229-36. [Crossref] [PubMed]
- Ripoll C, Banares R, Rincon D, et al. Influence of hepatic venous pressure gradient on the prediction of survival of patients with cirrhosis in the MELD Era. Hepatology 2005;42:793-801. [Crossref] [PubMed]
- Luca A, Cirera I, Garcia-Pagan JC, et al. Hemodynamic effects of acute changes in intra-abdominal pressure in patients with cirrhosis. Gastroenterology 1993;104:222-7. [Crossref] [PubMed]
- Myers JD, Taylor WJ. An estimation of portal venous pressure by occlusive catheterization of a hepatic venule. J Clin Invest 1951;30:662-3.
- de Franchis R. Expanding consensus in portal hypertension: Report of the Baveno VI Consensus Workshop: Stratifying risk and individualizing care for portal hypertension. J Hepatol 2015;63:743-52. [Crossref] [PubMed]
- Groszmann RJ, Wongcharatrawee S. The hepatic venous pressure gradient: anything worth doing should be done right. Hepatology 2004;39:280-2. [Crossref] [PubMed]
- Adinolfi LE, Marrone A, Rinaldi L. Non-alcoholic fatty liver disease: beyond the liver is an emerging multifaceted systemic disease. Hepatobiliary Surg Nutr 2018;7:143-6. [Crossref] [PubMed]
- Bosch J, Garcia-Pagan JC, Berzigotti A, et al. Measurement of portal pressure and its role in the management of chronic liver disease. Semin Liver Dis 2006;26:348-62. [Crossref] [PubMed]
- Bosch J, Abraldes JG, Berzigotti A, et al. The clinical use of HVPG measurements in chronic liver disease. Nat Rev Gastroenterol Hepatol 2009;6:573-82. [Crossref] [PubMed]
- Kumar M, Kumar A, Hissar S, et al. Hepatic venous pressure gradient as a predictor of fibrosis in chronic liver disease because of hepatitis B virus. Liver Int 2008;28:690-8. [Crossref] [PubMed]
- Blasco A, Forns X, Carrion JA, et al. Hepatic venous pressure gradient identifies patients at risk of severe hepatitis C recurrence after liver transplantation. Hepatology 2006;43:492-9. [Crossref] [PubMed]
- Groszmann RJ, Garcia-Tsao G, Bosch J, et al. Beta-blockers to prevent gastroesophageal varices in patients with cirrhosis. N Engl J Med 2005;353:2254-61. [Crossref] [PubMed]
- Silva-Junior G, Baiges A, Turon F, et al. The prognostic value of hepatic venous pressure gradient in patients with cirrhosis is highly dependent on the accuracy of the technique. Hepatology 2015;62:1584-92. [Crossref] [PubMed]
- Rincon D, Lo Iacono O, Ripoll C, et al. Prognostic value of hepatic venous pressure gradient for in-hospital mortality of patients with severe acute alcoholic hepatitis. Aliment Pharmacol Ther 2007;25:841-8. [Crossref] [PubMed]
- Procopet B, Cristea VM, Robic MA, et al. Serum tests, liver stiffness and artificial neural networks for diagnosing cirrhosis and portal hypertension. Dig Liver Dis 2015;47:411-6. [Crossref] [PubMed]
- Hayashi H, Beppu T, Okabe H, et al. Combined measurements of serum bile acid level and splenic volume may be useful to noninvasively assess portal venous pressure. J Gastroenterol 2012;47:1336-41. [Crossref] [PubMed]
- Hsieh YC, Lee KC, Wang YW, et al. Correlation and prognostic accuracy between noninvasive liver fibrosismarkers and portal pressure in cirrhosis: Role of ALBI score. PLoS One 2018;13:e0208903. [Crossref] [PubMed]
- Lim YL, Choi E, Jang YO, et al. Clinical Implications of the Serum Apelin Level on Portal Hypertension and Prognosis of Liver Cirrhosis. Gut Liver 2016;10:109-16. [Crossref] [PubMed]
- Sartoris R, Rautou PE, Elkrief L, et al. Quantification of Liver Surface Nodularity at CT: Utility for Detection of Portal Hypertension. Radiology 2018;289:698-707. [Crossref] [PubMed]
- Liu F, Ning Z, Liu Y, et al. Development and validation of a radiomics signature for clinically significant portal hypertension in cirrhosis (CHESS1701): a prospective multicenter study. EBioMedicine 2018;36:151-8. [Crossref] [PubMed]
- Min JK, Leipsic J, Pencina MJ, et al. Diagnostic accuracy of fractional flow reserve from anatomic CT angiography. JAMA 2012;308:1237-45. [Crossref] [PubMed]
- Lu MT, Ferencik M, Roberts RS, et al. Noninvasive FFR Derived From Coronary CT Angiography: Management and Outcomes in the PROMISE Trial. JACC Cardiovasc Imaging 2017;10:1350-8. [Crossref] [PubMed]
- Qi X, An W, Liu F, et al. Virtual Hepatic Venous Pressure Gradient with CT Angiography (CHESS 1601): A Prospective Multicenter Study for the Noninvasive Diagnosis of Portal Hypertension. Radiology 2019;290:370-7. [Crossref] [PubMed]
- Palaniyappan N, Cox E, Bradley C, et al. Non-invasive assessment of portal hypertension using quantitative magnetic resonance imaging. J Hepatol 2016;65:1131-9. [Crossref] [PubMed]
- Zhang QW, Wang Y, Wang J, et al. A non-invasive magnetic resonance imaging-based model predicts portal venous pressure. J Dig Dis 2016;17:175-85. [Crossref] [PubMed]
- Chouhan MD, Mookerjee RP, Bainbridge A, et al. Caval Subtraction 2D Phase-Contrast MRI to Measure Total Liver and Hepatic Arterial Blood Flow: Proof-of-Principle, Correlation With Portal Hypertension Severity and Validation in Patients With Chronic Liver Disease. Invest Radiol 2017;52:170-6. [Crossref] [PubMed]
- Huwart L, Sempoux C, Vicaut E, et al. Magnetic resonance elastography for the noninvasive staging of liver fibrosis. Gastroenterology 2008;135:32-40. [Crossref] [PubMed]
- Gharib AM, Han MAT, Meissner EG, et al. Magnetic Resonance Elastography Shear Wave Velocity Correlates with Liver Fibrosis and Hepatic Venous Pressure Gradient in Adults with Advanced Liver Disease. Biomed Res Int 2017;2017:2067479.
- Ophir J, Cespedes I, Ponnekanti H, et al. Elastography: a quantitative method for imaging the elasticity of biological tissues. Ultrason Imaging 1991;13:111-34. [Crossref] [PubMed]
- Sigrist RMS, Liau J, Kaffas AE, et al. Ultrasound Elastography: Review of Techniques and Clinical Applications. Theranostics 2017;7:1303-29. [Crossref] [PubMed]
- Wang CZ, Zheng J, Huang ZP, et al. Influence of measurement depth on the stiffness assessment of healthy liver with real-time shear wave elastography. Ultrasound Med Biol 2014;40:461-9. [Crossref] [PubMed]
- Xiao Y, Yu Y, Niu L, et al. Quantitative evaluation of peripheral tissue elasticity for ultrasound-detected breast lesions. Clin Radiol 2016;71:896-904. [Crossref] [PubMed]
- Xiao Y, Zeng J, Niu L, et al. Computer-aided diagnosis based on quantitative elastographic features with supersonic shear wave imaging. Ultrasound Med Biol 2014;40:275-86. [Crossref] [PubMed]
- Roccarina D, Rosselli M, Genesca J, et al. Elastography methods for the non-invasive assessment of portal hypertension. Expert Rev Gastroenterol Hepatol 2018;12:155-64. [Crossref] [PubMed]
- Elkrief L, Ronot M, Andrade F, et al. Non-invasive evaluation of portal hypertension using shear-wave elastography: analysis of two algorithms combining liver and spleen stiffness in 191 patients with cirrhosis. Aliment Pharmacol Ther 2018;47:621-30. [Crossref] [PubMed]
- Kumar A, Khan NM, Anikhindi SA, et al. Correlation of transient elastography with hepatic venous pressure gradient in patients with cirrhotic portal hypertension: A study of 326 patients from India. World J Gastroenterol 2017;23:687-96. [Crossref] [PubMed]
- Singh S, Fujii LL, Murad MH, et al. Liver stiffness is associated with risk of decompensation, liver cancer, and death in patients with chronic liver diseases: a systematic review and meta-analysis. Clin Gastroenterol Hepatol 2013;11:1573-84.e1-2; quiz e88-9.
- You MW, Kim KW, Pyo J, et al. A Meta-analysis for the Diagnostic Performance of Transient Elastography for Clinically Significant Portal Hypertension. Ultrasound Med Biol 2017;43:59-68. [Crossref] [PubMed]
- Ferraioli G, Tinelli C, Dal Bello B, et al. Accuracy of real-time shear wave elastography for assessing liver fibrosis in chronic hepatitis C: a pilot study. Hepatology 2012;56:2125-33. [Crossref] [PubMed]
- Attia D, Schoenemeier B, Rodt T, et al. Evaluation of Liver and Spleen Stiffness with Acoustic Radiation Force Impulse Quantification Elastography for Diagnosing Clinically Significant Portal Hypertension. Ultraschall Med 2015;36:603-10. [Crossref] [PubMed]
- Salzl P, Reiberger T, Ferlitsch M, et al. Evaluation of portal hypertension and varices by acoustic radiation force impulse imaging of the liver compared to transient elastography and AST to platelet ratio index. Ultraschall Med 2014;35:528-33. [Crossref] [PubMed]
- Zhu YL, Ding H, Fu TT, et al. Portal hypertension in hepatitis B-related cirrhosis: Diagnostic accuracy of liver and spleen stiffness by 2-D shear-wave elastography. Hepatol Res 2019;49:540-9. [Crossref] [PubMed]
- Elkrief L, Rautou PE, Ronot M, et al. Prospective comparison of spleen and liver stiffness by using shear-wave and transient elastography for detection of portal hypertension in cirrhosis. Radiology 2015;275:589-98. [Crossref] [PubMed]
- Procopet B, Berzigotti A, Abraldes JG, et al. Real-time shear-wave elastography: applicability, reliability and accuracy for clinically significant portal hypertension. J Hepatol 2015;62:1068-75. [Crossref] [PubMed]
- Buechter M, Manka P, Theysohn JM, et al. Spleen stiffness is positively correlated with HVPG and decreases significantly after TIPS implantation. Dig Liver Dis 2018;50:54-60. [Crossref] [PubMed]
- Tseng Y, Li F, Wang J, et al. Spleen and liver stiffness for noninvasive assessment of portal hypertension in cirrhotic patients with large esophageal varices. J Clin Ultrasound 2018;46:442-9. [Crossref] [PubMed]
- Song J, Huang J, Huang H, et al. Performance of spleen stiffness measurement in prediction of clinical significant portal hypertension: A meta-analysis. Clin Res Hepatol Gastroenterol 2018;42:216-26. [Crossref] [PubMed]
- Rifai K, Cornberg J, Bahr M, et al. ARFI elastography of the spleen is inferior to liver elastography for the detection of portal hypertension. Ultraschall Med 2011;32 Suppl 2:E24-30. [Crossref] [PubMed]
- Zykus R, Jonaitis L, Petrenkiene V, et al. Liver and spleen transient elastography predicts portal hypertension in patients with chronic liver disease: a prospective cohort study. BMC Gastroenterol 2015;15:183. [Crossref] [PubMed]
- Sharma P, Kirnake V, Tyagi P, et al. Spleen stiffness in patients with cirrhosis in predicting esophageal varices. Am J Gastroenterol 2013;108:1101-7. [Crossref] [PubMed]
- Jansen C, Bogs C, Verlinden W, et al. Shear-wave elastography of the liver and spleen identifies clinically significant portal hypertension: A prospective multicentre study. Liver Int 2017;37:396-405. [Crossref] [PubMed]
- Berzigotti A, Piscaglia F. Ultrasound in portal hypertension--part 1. Ultraschall Med 2011;32:548-68. [Crossref] [PubMed]
- Berzigotti A, Piscaglia F. EFSUMB Education and Professional Standards Committee. Ultrasound in portal hypertension--part 2--and EFSUMB recommendations for the performance and reporting of ultrasound examinations in portal hypertension. Ultraschall Med 2012;33:8-32. [PubMed]
- Kim MY, Baik SK, Park DH, et al. Damping index of Doppler hepatic vein waveform to assess the severity of portal hypertension and response to propranolol in liver cirrhosis: a prospective nonrandomized study. Liver Int 2007;27:1103-10. [Crossref] [PubMed]
- Choi YJ, Baik SK, Park DH, et al. Comparison of Doppler ultrasonography and the hepatic venous pressure gradient in assessing portal hypertension in liver cirrhosis. J Gastroenterol Hepatol 2003;18:424-9. [Crossref] [PubMed]
- Bernatik T, Strobel D, Hahn EG, et al. Doppler measurements: a surrogate marker of liver fibrosis? Eur J Gastroenterol Hepatol 2002;14:383-7. [Crossref] [PubMed]
- Maruyama H, Yokosuka O. Ultrasonography for Noninvasive Assessment of Portal Hypertension. Gut Liver 2017;11:464-73. [Crossref] [PubMed]
- Lim A, Cosgrove D. Functional studies. Eur Radiol 2004;14 Suppl 8:110-5. [Crossref] [PubMed]
- Chong WK, Papadopoulou V, Dayton PA. Imaging with ultrasound contrast agents: current status and future. Abdom Radiol (NY) 2018;43:762-72. [Crossref] [PubMed]
- Petelska AD, Janica JR, Kotynska J, et al. The effect of contrast medium SonoVue® on the electric charge density of blood cells. J Membr Biol 2012;245:15-22. [Crossref] [PubMed]
- Li F, Li D, Yan F. Improvement of Detection Sensitivity of Microbubbles as Sensors to Detect Ambient Pressure. Sensors (Basel) 2018;18:4083. [Crossref] [PubMed]
- Westwood M, Joore M, Grutters J, et al. Contrast-enhanced ultrasound using SonoVue(R) (sulphur hexafluoride microbubbles) compared with contrast-enhanced computed tomography and contrast-enhanced magnetic resonance imaging for the characterisation of focal liver lesions and detection of liver metastases: a systematic review and cost-effectiveness analysis. Health Technol Assess 2013;17:1-243. [Crossref] [PubMed]
- Lassau N, Koscielny S, Chami L, et al. Advanced hepatocellular carcinoma: early evaluation of response to bevacizumab therapy at dynamic contrast-enhanced US with quantification--preliminary results. Radiology 2011;258:291-300. [Crossref] [PubMed]
- Eisenbrey JR, Dave JK, Halldorsdottir VG, et al. Chronic liver disease: noninvasive subharmonic aided pressure estimation of hepatic venous pressure gradient. Radiology 2013;268:581-8. [Crossref] [PubMed]
- Zhang CX, Hu J, Hu KW, et al. Noninvasive analysis of portal pressure by contrast-enhanced sonography in patients with cirrhosis. J Ultrasound Med 2011;30:205-11. [Crossref] [PubMed]
- Kim MY, Suk KT, Baik SK, et al. Hepatic vein arrival time as assessed by contrast-enhanced ultrasonography is useful for the assessment of portal hypertension in compensated cirrhosis. Hepatology 2012;56:1053-62. [Crossref] [PubMed]
- Jeong WK, Kim TY, Sohn JH, et al. Severe portal hypertension in cirrhosis: evaluation of perfusion parameters with contrast-enhanced ultrasonography. PLoS One 2015;10:e0121601. [Crossref] [PubMed]
- Berzigotti A, Nicolau C, Bellot P, et al. Evaluation of regional hepatic perfusion (RHP) by contrast-enhanced ultrasound in patients with cirrhosis. J Hepatol 2011;55:307-14. [Crossref] [PubMed]
- Maruyama H, Shiha G, Yokosuka O, et al. Non-invasive assessment of portal hypertension and liver fibrosis using contrast-enhanced ultrasonography. Hepatol Int 2016;10:267-76. [Crossref] [PubMed]
- Shimada T, Maruyama H, Kondo T, et al. Impact of splenic circulation: non-invasive microbubble-based assessment of portal hemodynamics. Eur Radiol 2015;25:812-20. [Crossref] [PubMed]
- Fairbank WM Jr, Scully MO. A new noninvasive technique for cardiac pressure measurement: resonant scattering of ultrasound from bubbles. IEEE Trans Biomed Eng 1977;24:107-10. [Crossref] [PubMed]
- Li F, Wang L, Fan Y, et al. Simulation of noninvasive blood pressure estimation using ultrasound contrast agent microbubbles. IEEE Trans Ultrason Ferroelectr Freq Control 2012;59:715-26. [Crossref] [PubMed]
- Ishihara K, Kitabatake A, Tanouchi J, et al. New Approach to Noninvasive Manometry Based on Pressure Dependent Resonant Shift of Elastic Microcapsules in Ultrasonic Frequency Characteristics. Jpn J Appl Phys 1988;27:125. [Crossref]
- Bouakaz A, Frinking PJ, de Jong N, et al. Noninvasive measurement of the hydrostatic pressure in a fluid-filled cavity based on the disappearance time of micrometer-sized free gas bubbles. Ultrasound Med Biol 1999;25:1407-15. [Crossref] [PubMed]
- Postema M, Bouakaz A, de Jong N. Noninvasive microbubble-based pressure measurements: a simulation study. Ultrasonics 2004;42:759-62. [Crossref] [PubMed]
- Dave JK, Halldorsdottir VG, Eisenbrey JR, et al. Investigating the efficacy of subharmonic aided pressure estimation for portal vein pressures and portal hypertension monitoring. Ultrasound Med Biol 2012;38:1784-98. [Crossref] [PubMed]
- Shi WT, Forsberg F, Raichlen JS, et al. Pressure dependence of subharmonic signals from contrast microbubbles. Ultrasound Med Biol 1999;25:275-83. [Crossref] [PubMed]
- Dave JK, Halldorsdottir VG, Eisenbrey JR, et al. Noninvasive LV pressure estimation using subharmonic emissions from microbubbles. JACC Cardiovasc Imaging 2012;5:87-92. [Crossref] [PubMed]
- Dave JK, Halldorsdottir VG, Eisenbrey JR, et al. On the implementation of an automated acoustic output optimization algorithm for subharmonic aided pressure estimation. Ultrasonics 2013;53:880-8. [Crossref] [PubMed]
- Gupta I, Eisenbrey J, Stanczak M, et al. Effect of Pulse Shaping on Subharmonic Aided Pressure Estimation In Vitro and . J Ultrasound Med 2017;36:3-11. [Crossref] [PubMed]
- Dave JK, Kulkarni SV, Pangaonkar PP, et al. Non-Invasive Intra-cardiac Pressure Measurements Using Subharmonic-Aided Pressure Estimation: Proof of Concept in Humans. Ultrasound Med Biol 2017;43:2718-24. [Crossref] [PubMed]