β-(1→3)-Glucan-mannitol conjugates: scope and amazing results
Introduction
β-(1→3)-Glucans are amongst natural compounds that form a bridge between folkloric medicine and modern approaches of the pharmacopeia. Indeed, they were used in Chinese populations for their beneficial contributions on health which were later identified as stimulating agents of the immune system of mammals. Consequently, these polysaccharides that are found in seaweeds, fungi and yeasts have been the subject of many studies in complementary disciplines: analysis, synthetic chemistry, physicochemistry, biology and medicine (1). The main objectives of glycoscientists involved in this field are therefore to elucidate (I) the mechanisms inducing a biological answer in vivo according to the molecular weight, the branching units, and/or the hydrosolubility (2-6); and (II) the minimal structure from these polydisperse polysaccharides which is really active (7-9). The common objective is to identify and precisely characterize fine interactions between β-(1→3)-Glucans and natural macromolecular receptors (10-24). Our laboratory, in collaboration with Vetvicka’s group, is engaged in the chemical synthesis of oligo-β-(1→3)-Glucans having well defined molecular structure in order to remove any ambiguity in interpretation of biological results and so to offer greater opportunities to further develop potential drugs. We have thus demonstrated that small linear glucans, unable to generate well-defined conformations in water, are nevertheless able to stimulate the immune system in murine model. Moreover, we have studied their interaction with CR3.
β-(1→3)-Glucans extracted from brown seaweeds are composed of at least two families of polysaccharides. The first one, which accounts for one third of the mixture, is characterized by a glucose reducing end. Conversely, the second family presents a mannitol residue grafted in primary position (25,26). However, analytical data obtained in our laboratory suggest that another connection exists in Nature (V. Ferrières, unpublished data). Based on this observation, we present herein the synthesis of glucans conjugated to the 6-position or to the 3-hydroxyl of mannitol residue for their potential as bioactive oligosaccharides and analytical standards (Figure 1).
Results and discussion
According to structural and reaction considerations, a mannitol residue on the reducing end of an oligosaccharide can result from the reduction of a mannose entity. Such a strategy allowed us to prepare the molecular targets but also some analogues presenting one different stereogenic centre, i.e., mannose instead of glucose at the reducing end (Figure 1). Moreover, we focused our attention toward the possible elongation of the glucan chain thanks to an iterative sequence glycosylation/deprotection (8). Consequently, the first requirements for the synthesis of 1 and 3 dealt with the preparation of a mannosyl acceptor having a free primary hydroxyl, and glycosyl donors characterized by a good leaving group at the anomeric position (Figure 2). The former was prepared from mannose by first acido-catalyzed Fischer glycosylation of benzyl alcohol (27,28) followed by standard regioselective tritylation (29,30). The resulting intermediate 6 was subsequently either benzylated (31,32) or benzoylated and final detritylation afforded 7a (30,33) and 7b, respectively. The difference between both acceptors relies on nucleophilicity of the released hydroxyl which is less reactive in 7b than in 7a because of the electron withdrawing ability of the neighbouring benzoyl groups. This observation was experimentally corroborated by coupling reactions with the known thioglycoside 8 bearing a 2-naphthylmethyl (NAP) group at its 3-position, orthogonal to all other protections. Best glycosylation results from 7a and 8, followed by selective deprotection under oxido-reductive conditions, thereby giving the desired disaccharide 9 which was used as acceptor for further glycosylation with 8. Unfortunately, a trisaccharide presenting an unwanted new α-glycosidic linkage was obtained. We, and other authors, have previously observed such an amazing behavior of glycosyl donors bearing a participating protecting group, able to orientate the reaction towards the 1,2-trans anomer. Two interpretations can be given to support this particular reactivity: (I) a stereochemical mismatching between the donor and the acceptor in the transition state (34); and/or (II) a mechanism pathway involving three elemental steps: the formation of an orthoester which is opened by the nucleophilic attack of the triflate anion on the β-face finally followed by the rearrangement of the resulting intermediate into the α-compound (35).
With this information at hands, we turned out our strategy using donors already presenting the required β-configuration between the two glucosyl entities (Figure 3). Under suitable promoting conditions, the thioglycosides 11a (36) and 11b (37), as well as the trichloracetimidate 11c afforded the desired trisaccharide 12 which was chromatographically purified and isolated in 50%, 48%, and 80% yields, respectively. We observed that, in all cases, the best Lewis acid required to catalyze the reaction was trimethysilyl triflate (TMSOTf). We experimentally showed that other Lewis catalysts were not suitable to efficiently perform the rearrangement of the orthoester intermediate into the desired glycoside. Moreover, the control of the temperature during the transfer of the glycosyl part to the nucleophilic acceptor was also decisive. As a result, the more efficient conditions were obtained with the trichloroacetimidate donor 11c. Subsequent transesterification under Zemplen conditions and pallado-catalyzed debenzylation, both quantitatively, allowed the synthesis of the first target 3. The latter was finally reduced thanks to the use of sodium borohydride to give the laminaribiosyl-(1→6)-mannitol 1.
It resulted from this first synthesis and from literature data that (I) subtle structural modulation on both acceptor and donor may affect the behavior of the glycosylation process; and (II) a 4,6-benzylidene ring on both the donor and the acceptor is still the best protection to efficiently provide β-(1→3)-Glucanic chain (17,38-40). This inspired us for the following synthesis of the laminaribiosyl-(1→3)-mannose 2 and its reduced counterpart 4. Consequently, we have revisited the iterative synthesis of linear β-(1→3)-Glucans (8) since only the 2 position of the reducing end of the targets differ from linear β-(1→3)-Glucans. However, the approach is valid provided that the OH-2 of the reducing glucosyl residue bears an orthogonal protecting group compared to that of OH-2 of the other glucosyl entities. For that purpose, we opted for a levulinoyl protection on OH-2a and benzoyl ester for OH-2b and OH-2c (Figure 4). Consequently, the synthetic scheme first began with known compound 14 that was a key building block for the preparation of both 15 by standard levulynoylation followed by anomeric protection by a benzyl group, and benzoylation that provided donor 17. Regioselective oxido-reductive deprotection of the 3 position by dicyanodichloroquinone (DDQ) in methanol into 15 yielded the acceptor 16 that was couple to 17 to give the disaccharide 18 in excellent 94% yield. Chain elongation further followed the two-step procedure: DDQ assisted deprotection, and glycosylation step starting with the resulting nucleophile 19 and the same key donor 17. At this stage of the synthesis, the iterative strategy differed from that previously described by selective deprotection of the 2a hydroxyl group by hydrazine acetate in 82% yield. After experimentation, the best oxidative reagent was the Dess-Martin periodinan that allowed production of the ketone 22 in a quantitative yield. Its conversion into the mannosyl derivative 23 was carried out quantitatively thanks to the hydride L-Selectride at –80 °C. Further Zemplen transesterfication and pallado-catalyzed debenzylation afforded the desired laminaribiosyl-(1→3)-mannose 4, that was finally reduced by sodium borohydride to yield the laminaribiosyl-(1→3)-mannitol 2. This iterative startegy complemented well the uloside approach previously describe starting from pure oligo-β-(1→3)-Glucans (41-43).
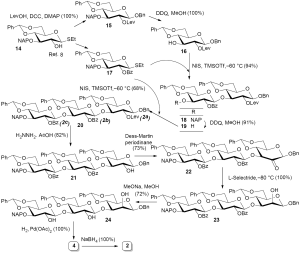
On an analytical point of view, small structural modulations on the reducing end induced significant differences in NMR data, i.e., on the mannose or mannitol entity. Indeed, coupling constants between H-1 and H-2 for the glucosyl residues b and c range between 7.5 and 8.0 Hz, thus corroborating the desired β linkages. However the most interesting information has been provided from 13C NMR since glycosylation on a specific oxygen atom should cause a significant shift of the signal corresponding to the involved carbon centre towards the lower fields. By comparing the data, the C-6a in 1 and 3 appears at 72.2 and 69.3 ppm, respectively, while the same signal appear at only 63.4 and 61.0 in 2 and 4, respectively. A similar observation is possible considering now the signals of the C-3a centres: 69.4 ppm for 1 and 66.7 ppm for 3, but 77.7 ppm for 2 and 78.5 ppm for 4.
Conclusions
The synthesis of two mannitol derivatives, grafted by laminaribiose—a precursor of longer β-(1→3)-Glucan chain—was performed targeting the primary and the three positions that are found in nature. We have shown that the synthetic approaches were efficient by using a mannoside as acceptor, but that they must be adapted to the nature of the desired glycosidic linkage. Thus, the (1→6)-bound required direct connection of a disaccharidyl donor since further elongation was disrupted by the presence of the mannosyl residue itself. The (1→3)-linkage was efficiently achieved by revisiting the iterative strategy that was previously developed for the preparation of linear oligo-β-(1→3)-Glucans. This study resulted in the synthesis of two non-natural laminaribiosyl-mannose derivatives and two laminaribiosyl-mannitol ones, whose analytical data will be valuable for further characterization of extracted β-(1→3)-Glucans.
Analytical data of compounds 1-4
Trisaccahride 3 (α/β =1.0:0.3)
1H RMN (D2O, 400 MHz) δ (ppm): 5.08 (s, 1H, H-1aα), 4.83 (m, 0,3H, H-1aβ), 4,66 (d, 1,3H, H-1c, JH1-H2 =7.7 Hz), 4.46 (d, 0,3H, H-1bβ, JH1-H2 =7.7 Hz), 4.45 (d, 1H, H-1bα, JH1-H2 =7.8 Hz), 4.13-4.06 (m, 1,3H, H-6a), 3.91-3.72 (m, 7.8H, H-2a, H-4a, H-5a, H-6’a, H-6b, H-6c), 3.71-3.52 (m, 5,2H, H-3a, H-3b, H-6’b, H-6’c), 3.50-3.22 (m, 9.1H, H-2b, H-4b, H-5b, H-2c, H-3c, H-4c, H-5c); 13C RMN (D2O, 100 MHz) δ (ppm): 103.1 (C-1c), 102.8 (C-1b), 94.4 (C-1aα), 94.1 (C-1aβ), 84.5 (C-3b), 76.3 (C-5c), 75.8 (C-3c, C-5b), 73.8 (C-2c), 73.2 (C-2b), 71.7 (C-4aα), 71.5 (C-4aβ), 70.9 (C-2aα), 70.6 (C-2aβ), 70.4 (C-5aα, C-5aβ), 69.9 (C-4c), 69.3 (C-6a), 68.5 (C-4b), 66.9 (C-3aα), 66.7 (C-3aβ), 61.0 (C-6b, C-6c). HRMS (ESI+): calcd m/z for [M+Na]+ C18H32NaO16 527.1588, found m/z 527.1580; calcd for [M+K]+ C18H32KO16 543.1327, found m/z 543.1334.
Laminaribiosyl-β-(1→6)-mannitol 1
1H RMN (D2O, 400 MHz) δ (ppm): 4.67 (d, 1H, H-1c, JH1-H2 =7.8 Hz), 4.46 (d, 1H, H-1b, JH1-H2 =8.0 Hz), 4.11 (dd, 1H, H-6a, JH5-H6 =1.8 Hz, JH6-H6’ =10.6 Hz), 3.88-3.56 (m, 12H, H-1a, H-1’a, H-2a, H-3a, H-3b, H-4a, H-5a, H-6b, H-6c, H-6’a, H-6’b, H-6’c), 3.48-3.37 (m, 5H, H-2b, H-3c, H-4b, H-5b, H-5c), 3.32 (t, 1H, H-4c, JH3-H4 = JH4-H5 =9.0 Hz), 3.27 (dd, 1H, H-2c, JH2-H3 =9.3 Hz); 13C RMN (D2O, 100 MHz) δ (ppm): 103.1 (C-1c), 103.0 (C-1b), 84.6 (C-3b), 76.3 (C-5c), 75.9, 75.8 (C-3c, C-5b), 73.8 (C-2c), 73.3 (C-2b), 72.2 (C-6a), 71.1 (C-2a), 70.0 (C-5a), 69.9 (C-4c), 69.4 (C-3a), 69.3 (C-4a), 68.5 (C-4b), 63.5 (C-1a), 61.0 (C-6b, C-6c); HRMS (ESI+): [M+Na]+ C18H34NaO16, calcd m/z 529.1745, measured m/z 529.1739; [M+K]+ C18H34KO16, calcd m/z 545.1484, measured m/z 545.1476; [M-H+2Na]+ C18H33Na2O1, calcd m/z 551.1564, measured m/z 551.1570.
Trisaccharide 4
1H RMN (D2O, 400 MHz) δ (ppm): 5.13 (d, 1H, H-1aα, JH1a-H2a =1.8 Hz), 4.67 (d, 1H, H-1c, JH1c-H2c =7.7 Hz), 4.53 (d, 1H, H-1b, JH1b-H2b =8.0 Hz), 4.03 (dd, 1H, H-2a, JH2-H3 =3.1 Hz), 3.96 (dd, 1H, H-3a, JH3-H4 =9.5 Hz), 3.90-3.80 (m, 3H, H-6a, H-6b, H-6c), 3.80-3.73 (m, 1H, H-4a), 3.72-3.55 (m, 5H, H-5a, H-6’a, H-3b, H-6’b, H-6’c), 3.53-3.36 (m, 5H, H-2b, H-64b, H-5b, H-3c, H-5c), 3.32 (t, 1H, H-4c, JH3-H4 = JH4-H5 =9.7 Hz), 3.27 (dd, 1H, H-2c, JH2-H3 =9.3 Hz); 13C RMN (D2O, 100 MHz) δ (ppm): 103.1 (C-1c), 100.5 (C-1b), 94.1 (C-1aα), 84.5 (C-3b), 78.5 (C-3a), 76.3 (C-5c), 75.9 (C-3c, C-5b), 75.8 (C-2c), 73.1 (C-2b), 72.7 (C-4a), 69.9 (C-4c), 68.6 (C-2a), 68.4 (C-4b), 65.5 (C-5a), 61.2, 61.0 (C-6a, C-6b, C-6c); HRMS (ESI+): [M+Na]+ C18H32NaO16, calcd m/z 527.1588, measured m/z 527.1587; [M+K]+ C18H32KO16, calcd m/z 543.1327, measured m/z 543.1343.
Laminaribiosyl-β-(1→3)-mannitol 2
1H RMN (D2O, 400 MHz) δ (ppm): 4.82 (d, 1H, H-1c, JH1-H2 =8.0 Hz), 4.65 (d, 1H, H-1b, JH1-H2 =8.1 Hz), 4.09-3.90 (m, 7H, H-1a, H-2a, H-3a, H-5a, H-6a, H-6b, H-6c), 3.86-3.66 (m, 6H, H-1’a, H-4a, H-6’a, H-3b, H-6’b, H-6’c), 3.64-3.38 (m, 7H, H-2b, H-4b, H-5b, H-2c, H-3c, H-4c, H-5c); 13C RMN (D2O, 100 MHz) δ (ppm): 103.1 (C-1c), 102.5 (C-1b), 84.4 (C-3b), 77.3 (C-3a), 76.3 (C-5c), 75.8 (C-3c), 75.5 (C-5b), 73.7 (C-2c), 73.4 (C-2b), 70.9, 70.8 (C-2a, C-5a), 69.9 (C-4c), 69.6 (C-4a), 68.8 (C-4b), 63.4 (C-6a), 62.6 (C-1a), 61.2, 61.0 (C-6b, C-6c); HRMS (ESI+): calcd m/z for [M+Na]+ C18H34NaO16: 529.1745, found 529.1743; calcd m/z for [M+K]+ C18H34KO16: 545.1484, found 545.1480; calcd m/z for [M-H+2Na]+ C18H33Na2O16 551.1564, found 551.1561.
Acknowledgements
K.D. acknowledges Goemar Laboratories for a PhD grant. The authors are also grateful to Cancéropole Grand Ouest, and more especially the axis Produits de la Mer en Cancérologie, for complementary financial supports.
Disclosure: This original work is exclusively proposed for publication in Annals of Translational Medicine and was not submitted elsewhere. The company Goemar has partly supported this work, so that people scientifically involved in this work are obviously coauthors of the paper.
Supplementary material
Benzyl 2,3,4-tri-O-benzoyl-α-D-mannopyranoside 7b: to a solution of 6 (3.48 g, 6.79 mmol) in pyridine (50 mL) was added benzoyl chloride (4.71 mL, 40.76 mmol). The reaction was stirred for 2 d at RT before the solvent was removed under reduced pressure. The crude was then diluted in AcOEt (50 mL) and washed with a 10% aq sol HCl, a sat aq sol of NaHCO3, and brine. The organic layer was dried over MgSO4 and concentrated. The resulting crude was further diluted in anhydride acetic (80 mL), warmed at 100 °C for 20 min and added of water (20 mL). After stirring for 30 min, the media was cooled to 0 °C under reduced pressure. The desired compound 7b was finally purified by chromatography [light petroleum/AcOEt (7:3; v/v)] and isolated in a 72% yield for two steps (2.47 g). Rf (light petroleum/AcOEt, 7:3) 0.3. [α]20D +73.5 (c =2.0, CHCl3). 1H RMN (CDCl3, 400 MHz) δ (ppm): 8.05-8.00 (m, 2H, H arom), 7.88-7.82 (m, 2H, H arom), 7.72-7.76 (m, 2H, H arom), 7.55-7.10 (m, 14H, H arom), 5.95 (dd, 1H, H-3, JH2-H3 =3.6 Hz, JH3-H4 =10.2 Hz), 5.77 (t, 1H, H-4, JH4-H5 =10.2 Hz), 5.66 (dd, 1H, H-2, JH1-H2 =1.5 Hz), 5.13 (d, 1H, H-1), 4.75 (d, 1H, CH2Ph, J =11.9 Hz), 4.62 (d, 1H, CH2Ph), 4.00 (ddd, 1H, H-5, JH5-H6 =2.3 Hz, JH5-H6’ =3.8 Hz), 3.72 (dd, 1H, H-6, JH6-H6’ =13.0 Hz), 3.67 (dd, 1H, H-6’). 13C RMN (CDCl3, 100 MHz) δ (ppm): 165.6, 165.5, 165.5 (OCOPh), 136.4, 133.7, 133.5, 133.2 (C quat arom), 130.1, 129.9, 129.7, 129.2, 128.6, 128.5, 128.4, 128.3, 128.2, 127.9, 127.8 (C arom), 97.1 (C-1), 71.1 (C-5), 70.6 (C-2), 70.1 (CH2Ph), 69.6 (C-3), 67.3 (C-4), 61.2 (C-6).
Ethyl 2,4,6-tri-O-acetyl-3-O-(2-methylnaphtyl)-1-thio-β-D-glucopyranoside 8: to a solution of ethyl 3-O-(2-methylnaphtyl)-1-thio-β-D-glucopyranoside (1 g, 2.46 mmol) in pyridine (10 mL) was added dropewise acetic anhydride (1.2 mL, 12.73 mmol) and the reaction was stirred at RT for 20 h. After removal of the solvent under reduced pressure, the crude was diluted in CH2Cl2, washed with 10% aq sol HCl, sat aq sol of NaHCO3, and brine. The organic layer was dried (MgSO4), concentrated, and purified by chromatography [light petroleum/AcOEt (7:3; v/v)] to yield 8 as a white solid (1.08 g, 90%). mp 134 °C. Rf (light petroleum/AcOEt, 7:3) 0.3. [α]20D –19.6 (c =1.0, CH2Cl2). 1H RMN (CDCl3, 400 MHz) δ (ppm): 7.83-7.33 (m, 7H, H arom), 5.14 (t, 1H, H-2, JH1-H2 = JH2-H3 =9.9 Hz), 5.12 (t, 1H, H-4, JH3-H4 = JH4-H5 =9.9 Hz), 4.80 (d, 1H, CH2Ar, J =12.0 Hz), 4.75 (d, 1H, CH2Ar), 4.40 (d, 1H, H-1), 4.19 (dd, 1H, H-6, JH5-H6 =5.4 Hz, JH6-H6’ =12.5 Hz), 4.11 (dd, 1H, H-6’, JH5-H6’ =2.3 Hz), 3.76 (t, 1H, H-3), 3.60 (ddd, 1H, H-5), 2.76-2.64 (m, 2H, CH2CH3), 2.07, 1.98, 1.92 (3s, 9H, COCH3), 1.26 (t, 3H, CH3, 2J =7.4 Hz). 13C RMN (CDCl3, 100 MHz) δ (ppm): 170.8, 169.4, 169.3 (3C, COCH3), 135.2, 133.1, 132.9 (C quat arom), 128.2, 127.8, 127.6, 126.4, 126.2, 126.0, 125.6 (C arom), 83.6 (C-1), 81.4 (C-3), 76.1 (C-5), 74.2 (C-7), 71.2 (C-2), 69.6 (C-4), 62.4 (C-6), 23.9 (CH2CH3), 20.9, 20.8, 20.8 (COCH3), 14.7 (CH2CH3). Elemental analysis: calcd for C25H30O8S: C 61.21%, H 6.16%, S 6.54%; measured: C 61.64%, H 6.30%, S 6.20%.
Benzyl 2,4,6-tri-O-acetyl-β-D-glucopyranosyl-(1→6)-2,3,4-tri-O-benzyl-α-D-mannopyranoside 9: to a solution of donor 8 (100 mg, 0.204 mmol) and acceptor 7a (92 mg, 0.170 mmol) in CH2Cl2 were successively added N-iodosuccinimide (NIS, 58 mg, 0.258 mmol) and trimethylsilyl triflate (TMSOTf, 1.5 µL, 8 µmol). After 30 min at RT, the reaction was quenched by adding few drops of NEt3. The mixture was then filtered, concentrated and submitted to chromatographic purification [light petroleum/AcOEt (7:3, v/v)]. This afforded a disaccharide (91 mg, 94 µmol) isolated in 55% yield and as a colorless oil. Rf (light petroleum/AcOEt, 7:3) 0.3. The product was further dissolved in a 4:1 mixture of CH2Cl2, CH3OH (10 mL) before adding 2,3-dichloro-5,6-dicyano-1,4-benzoquinone (DDQ, 64 mg, 0.282 mmol). After stirring overnight at RT, dilution with CH2Cl2was performed (40 mL). The resulting organic layer was washed with sat aq NaHCO3, water, dried (MgSO4), and concentrated. The residue was purified by chromatography [light petroleum/AcOEt (10:7; v/v)] to give 9 (53 mg) as a colorless oil and in 68% yield. Rf (light petroleum/AcOEt, 10:7) 0.1. 1H RMN (CDCl3, 400 MHz) δ (ppm): 7.29-7.16 (m, 20H, H arom), 4.88 (t, 1H, H-4b, JH3-H4 = JH4-H5 =9.7 Hz), 4.87 (d, 1H, OCH2Ph, 2J =11.2 Hz), 4.83 (d, 1H, H-1a, JH1-H2 =1.6 Hz), 4.82 (dd, 1H, H-2b, JH1-H2 =7.6 Hz, JH2-H3 =9.7 Hz), 4.62 (d, 1H, C-1OCH2Ph, J =11.4 Hz), 4.62 (s, 2H, OCH2Ph), 4.51 (s, 2H, OCH2Ph), 4.51 (d, 1H, OCH2Ph), 4.45 (d, 1H, H-1b), 4.35 (d, 1H, C-1OCH2Ph), 4.16 (dd, 1H, H-6b, JH5-H6 =4.8 Hz, JH6-H6’ =12.2 Hz), 4.04 (dd, 1H, H-6’b, JH5-H6’ =2.3 Hz), 4.04 (dd, 1H, H-6a, JH5-H6 =4.0 Hz, JH6-H6’ =11.0 Hz), 3.87 (dd, 1H, H-3a, JH2-H3 =3.0 Hz, JH3-H4 =8.9 Hz), 3.81-3.73 (m, 2H, H-4a, H-5a), 3.73 (dd, 1H, H-2a), 3.67 (dd, 1H, H-6’a, JH5-H6’ =5.6 Hz), 3.59 (dt, 1H, H-3b, JH3-OH3 =7.1 Hz), 3.48 (ddd, 1H, H-5b), 2.65 (d, 1H, OH-3b), 2.02, 1.96, 1.91 (3 s, 9H, COCH3). 13C RMN (CDCl3, 100 MHz) δ (ppm): 170.8, 170.6, 170.4 (OCOCH3), 138.3, 138.0, 137.0 (C quat arom), 128.4, 128.3, 128.1, 127.9, 127.7, 127.6, 127.5 (C arom), 100.7 (C-1b), 96.8 (C-1a), 80.1 (C-3a), 74.9 (OCH2Ph), 74.8 (C-4a), 74.3 (C-2a), 74.1 (C-2b), 73.9 (C-3b), 72.6 (OCH2Ph), 72.0 (OCH2Ph), 71.7 (C-5a), 71.6 (C-5b), 70.9 (C-4b), 68.6 (C-6a), 68.5 (C-1OCH2Ph), 62.1 (C-6b), 20.8, 20.7 (OCOCH3).
Benzyl 2,4,6-tri-O-acetyl-3-O-(2-methylnaphtyl)-α-D-glucopyranosyl-(1→3)-2,4,6-tri-O-acetyl-β-D-glucopyranosyl-(1→6)-2,3,4-tri-O-benzyl-α-D-mannopyranoside 10: to a solution of 8 (38 mg, 0.077 mmol) and 9 (53 mg, 0.064 mmol) in CH2Cl2 cooled at –10 °C were successively added NIS (22 mg, 0.098 mmol) and TMSOTf (0.6 µL, 3 μmol). After 45 min, few drops of NEt3 were added, the mixture was filtered, the filtrate concentrated under reduced pressure, and the crude was subjected to column chromatography [light petroleum/AcOEt (3:2, v/v)]. The trisaccharide (48 mg) was isolated in 60% yield. Rf (light petroleum/AcOEt, 3:2) 0.3. 1H RMN (CDCl3, 400 MHz) δ (ppm): 7.76-7.70 (m, 3H, H arom), 7.62 (s, 1H, H arom), 7.38-7.35 (m, 2H, H arom), 7.30-7.17 (m, 22H, H arom), 5.19 (d, 1H, H-1c, JH1-H2 =3.6 Hz), 5.10 (t, 1H, H-4c, JH3-H4 = JH4-H5 =9.7 Hz), 5.04 (dd, 1H, H-2b, JH1-H2 =7.9 Hz, JH2-H3 =9.7 Hz), 5.00 (t, 1H, H-4b, JH3-H4 = JH4-H5 =9.9 Hz), 4.87 (d, 1H, CH2 NAP, J =10.9 Hz), 4.81 (d, 1H, H-1a, JH1-H2 =1.5 Hz), 4.75 (d, 1H, OCH2Ph, 2J =12.0 Hz), 4.70 (dd, 1H, H-2c, JH2-H3 =10.2 Hz), 4.64 (d, 1H, OCH2Ph), 4.63 (s, 2H, OCH2Ph), 4.61 (d, 1H, C-1OCH2Ph, J =11.4 Hz), 4.51 (s, 2H, OCH2Ph), 4.49 (d, 1H, CH2 NAP), 4.33 (d, 1H, H-1b), 4.32 (d, 1H, C-1OCH2Ph), 4.12 (dd, 1H, H-6b, JH5-H6 =4.6 Hz, JH6-H6’ =12.5 Hz), 4.08 (dd, 1H, H-6a, JH5-H6 =1.8 Hz, JH6-H6’ =10.4 Hz), 4.08 (dd, 1H, H-6c, JH5-H6 =5.0 Hz, JH6-H6’ =10.7 Hz), 3.99 (dd, 1H, H-6’b, JH5-H6’ =2.5 Hz), 3.96 (dd, 1H, H-6’c, JH5-H6’ =2.0 Hz), 3.88 (dd, 1H, H-3a, JH2-H3 =3.1 Hz, JH3-H4 =9.7 Hz), 3.87 (dd, 1H, H-3c), 3.86 (ddd, 1H, H-5c), 3.81 (t, 1H, H-3b), 3.79 (ddd, 1H, H-5a, JH4-H5 =9.7 Hz, JH5-H6’ =7.2 Hz), 3.74 (dd, 1H, H-2a), 3.67 (t, 1H, H-4a), 3.58 (dd, 1H, H-6’a), 3.43 (ddd, 1H, H-5b), 1.99, 1.96, 1.86, 1.68 (4 s, 18H, COCH3). 13C RMN (CDCl3, 100 MHz) δ (ppm): 170.7, 169.4, 169.2, 168.9 (OCOCH3), 138.3, 138.2, 138.0, 137.1, 135.5, 133.2, 132.8 (C quat arom), 128.4, 128.3, 128.1, 127.9, 127.8, 127.7, 127.6, 127.5, 126.1, 125.9, 125.5 (C arom), 101.1 (C-1b), 96.8 (C-1a), 95.8 (C-1c), 80.2 (C-3a), 76.7 (C-3c), 76.2 (C-3b), 75.2 (CH2 NAP), 75.0 (C-4a, OCH2Ph), 74.3 (C-2a), 73.2 (C-2c), 72.8 (OCH2Ph), 72.1 (OCH2Ph), 71.7 (C-5b, C-2b), 71.5 (C-5a), 70.2 (C-4c), 69.0 (C-6a, C-4b), 68.5 (C-1OCH2Ph), 68.4 (C-5c), 61.8 (C-6b), 61.4 (C-6c), 20.9, 20.8, 20.7, 20.6 (OCOCH3). HRMS (ESI+): [M+Na]+ C69H76NaO22 calcd m/z 1,279.4726, found m/z 1279.4705; [M+K]+ C69H76KO22 calcd m/z 1,295.4465, found m/z 1,295.4537.
2,3,4,6-tetra-O-acetyl-β-D-glucopyranosyl-(1→3)-2,4,6-tri-O-acetyl-α-D-glucopyranosyl trichloroacetimidate 11c: to a solution of peracetylated laminaribiose (2.2 g, 3.25 mmol in CH2Cl2 (25 mL) was added morpholine (1.4 mL, 16.25 mmol). After stirring under reflux for 4 h, the mixture was cooled at RT, diluted with CH2Cl2 (25 mL), washed with sat aq NaHCO3, and water. The organic layer was then dried (MgSO4) and concentrated to yield the desired hemiacetal. The latter (2.07 g, 3.25 mmol) was dissolved in CH2Cl2 (25 mL) and 1,8-diazobicyclo[5,4,0]undec-7-ene (DBU, 100 μL, 0.65 mmol) and trichloroacetonitrile (3.3 mL, 32.5 mmol) were added at RT. After 1 h, the reaction media was concentrated and the resulting crude purified by chromatography [light petroleum/AcOEt (3:2, v/v)] to afford 11c (1.86 g, 74 over 2 steps). Rf (light petroleum/AcOEt, 3:2) 0.25. [α]20D +41.6 (c =1.0, CH2Cl2). 1H RMN (CDCl3, 400 MHz) δ (ppm): 8.70 (s, 1H, NH), 6.44 (d, 1H, H-1a, JH1-H2 =3.8 Hz), 5.15 (dd, 1H, H-3b, JH2-H3 = JH3b-H4b =9.5 Hz), 5.11 (dd, 1H, H-4a, JH3-H4 = JH4-H5=9.7 Hz), 5.08 (dd, 1H, H-2a, JH2-H3 =9.9 Hz), 5.06 (dd, 1H, H-4b, JH4-H5 =9.5 Hz), 4.90 (dd, 1H, H-2b, JH1-H2 =8.1 Hz), 4.65 (d, 1H, H-1b), 4.35 (dd, 1H, H-6b, JH5-H6 =4.6 Hz, JH6-H6’ =12.4 Hz), 4.21-4.07 (m, 5H, H-3a, H-5a, H-6a, H-6’a, H-6’b), 3.73 (ddd, 1H, H-5b, JH5-H6’ =2.3 Hz), 2.10, 2.08, 2.06, 2.05, 2.00, 1.97 (6s, 21H, COCH3). 13C RMN (CDCl3, 100 MHz) δ (ppm): 170.7, 170.5, 170.3, 169.6, 169.3, 169.1 (COCH3), 160.5 (CNHCCl3), 100.9 (C-1b), 93.1 (C-1a), 90,7 (CCl3), 76.4 (C-3a), 72.8 (C-3b), 71.7 (C-5b), 71.6 (C-2a), 71.3 (C-2b), 70.2 (C-5a), 68.0 (C-4b), 67.1 (C-4a), 61.6 (C-6b), 61.5 (C-6a), 20.7, 20.6, 20.5, 20.3 (COCH3). HRMS (ESI+): [M+Na]+ C28H36NaNO18Cl3 calcd m/z 802.0896, found m/z 802.0899.
Benzyl 2,3,4,6-tetra-O-acetyl-β-D-glucopyranosyl-(1→3)-2,4,6-tri-O-acetyl-β-D-glucopyranosyl-(1→6)-2,3,4-tri-O-benzyl-α-D-mannopyranoside 12: a solution of 11c (1.85 g, 2.369 mmol) and 7a (1.17 g, 2.164 mmol) in CH2Cl2 was cooled at -50 °C before adding TMSOTf (15 µL, 0.083 mmol). The reaction was quenched after stirring for 15 min by NEt3, concentrated and purified by chromatography [light petroleum/AcOEt (1:1, v/v)] to afford an orthoester (1.98 g) which was rearranged after dissolution (CH2Cl2, 10 mL) thanks to TMSOTf (20 μL, 0.111 mmol) at 0 °C. After neutralization by NEt3, concentration and chromatography [light petroleum/AcOEt (1:1, v/v)], the expected trisaccharide 12 (1.83 g) was obtained as a white solid in 73% yield. mp 70 °C. Rf (light petroleum/AcOEt, 1:1) 0.4. [α]20D +11.6 (c =1.0, CH2Cl2). 1H RMN (CDCl3, 400 MHz) δ (ppm): 7.30-7.20 (m, 20H, H arom), 5.06 (t, 1H, H-3c, JH2-H3 = JH3-H4=9.4 Hz), 5.00 (t, 1H, H-4c, JH4-H5 =9.4 Hz), 4.99 (dd, 1H, H-2b, JH1-H2 =8.2 Hz, JH2-H3 =9.7 Hz), 4.88 (t, 1H, H-4b, JH3-H4 = JH4-H5 =9.7 Hz), 4.87 (d, 1H, OCH2Ph, 2J =10.9 Hz), 4.83 (dd, 1H, H-2c, JH1-H2 =7.9 Hz), 4.82 (d, 1H, H-1a, JH1-H2 =1.7 Hz), 4.64 (s, 2H, OCH2Ph), 4.60 (d, 1H, OCH2Ph, J =12.0 Hz), 4.53 (s, 2H, OCH2Ph), 4.51 (d, 1H, OCH2Ph), 4.50 (d, 1H, H1c), 4.36 (d, 1H, H1b), 4.34 (d, 1H, C-1OCH2Ph), 4.32 (dd, 1H, H-6c, JH5-H6 =4.3 Hz, JH6-H6’ =12.7 Hz), 4.14-4.04 (m, 3H, H-6a, H-6b, H-6’b), 3.98 (dd, 1H, H-6’c, JH5-H6’ =2.3 Hz), 3.88 (dd, 1H, H-3a, JH2-H3 =3.0 Hz, JH3-H4 =9.2 Hz), 3.82-3.77 (m, 2H, H-3b, H-5a), 3.76 (dd, 1H, H-2a), 3.68 (t, 1H, H-4a, JH4-H5 =9.2 Hz), 3.65-3.52 (m, 3H, H-5b, H-5c, H-6’a), 2.08, 2.03, 2.00, 1.99, 1.97 (5s, 21H, COCH3). RMN 13C (CDCl3, 100 MHz) δ (ppm): 170.8, 170.5, 170.3, 169.4, 169.2, 169.1, 168.4 (COCH3), 138.3, 138.2, 138.0, 137.1 (C quat arom), 128.4, 128.3, 127.9, 127.8, 127.7, 127.6, 127.5 (C arom), 100.9 (C-1b, C-1c), 96.7 (C-1a), 80.2 (C-3a), 78.9 (C-3b), 74.9 (C-4a, OCH2Ph), 74.4 (C-2a), 72.9 (C-3c), 72.8 (OCH2Ph), 72.4 (C-2b), 72.1 (OCH2Ph), 71.7, 71.6, 71.5 (C-5a, C-5b, C-5c), 70.9 (C-2c), 68.7 (C-6a), 68.4 (C-1OCH2Ph), 68.2 (C-4b), 67.9 (C-4c), 62.0 (C-6b), 61.6 (C-6c), 20.8, 20.7, 20.6, 20.5, 20.3 (COCH3). Elemental analysis calcd for C60H70O23: C 62.17%, H 6.09%; found C 62.09%, H 6.20%.
Benzyl β-D-glucopyranosyl-(1→3)-β-D-glucopyranosyl-(1→6)-2,3,4-tri-O-benzyl-α-D-mannopyranoside 13: deacylation of 12 (883 mg, 0.761 mmol) was performed in 5:1:1 CH3OH/NEt3/H2O mixture (5 mL). After stirring overnight, the solvent was evaporated under reduced pressure to give 13 (655 mg) in a quantitative yield as a whithe solid. mp 95 °C. Rf (CH2Cl2/MeOH, 9:1) 0.2. [α]20D +13.8 (c =1.0, CH2Cl2). 1H RMN (CDCl3, 400 MHz) δ (ppm): 7.32-7.19 (m, 20H, H arom), 4.85 (d, 1H, OCH2Ph, 2J =11.2 Hz), 4.83 (d, 1H, H-1a, JH1-H2 <1.0 Hz), 4.63 (d, 1H, C-1OCH2Ph, J =12.2 Hz), 4.60 (s, 2H, OCH2Ph), 4.59 (d, 1H, OCH2Ph), 4.52 (d, 1H, OCH2Ph, 2J =11.4 Hz), 4.51 (d, 1H, OCH2Ph), 4.44 (d, 1H, H-1c, JH1-H2 =7.6 Hz), 4.38 (d, 1H, C-1OCH2Ph), 4.28 (d, 1H, H-1b, JH1-H2 =7.1 Hz), 4.05-4.02 (m, 1H, H-6a), 3.90-3.86 (m, 2H, H-3a, H-4a), 3.83-3.70 (m, 5H, H-2a, H-5a, H-6’a, H-6b, H-6c), 3.67 (dd, 1H, H-6’b, JH5-H6 =4.8 Hz, JH6-H6’ =12.2 Hz), 3.61 (dd, 1H, H-6’c, JH5-H6 =5.3 Hz, JH6-H6’ =11.7 Hz), 3.47-3.40 (m, 3H, H-2b, H-3b, H-4b), 3.38-3.35 (m, 1H, H-3c), 3.33-3.24 (m, 3H, H-2c, H-4c, H-5c), 3.24-3.20 (m, 1H, H-5b). 13C RMN (CDCl3, 100 MHz) δ (ppm): 138.0, 137.9, 137.3, 136.7 (C quat arom), 128.2, 128.1, 127.9, 127.8, 127.7, 127.6, 127.5, 127.4, 127.3 (C arom), 103.9 (C-1c), 102.9 (C-1b), 96.8 (C-1a), 87.0 (C-3b), 79.6 (C-3a), 76.3 (C-5c), 76.0 (C-3c), 75.4 (C-5b), 74.9 (OCH2Ph), 74.3 (C-4a, C-5a), 73.8 (C-2c), 72.6 (OCH2Ph), 72.4 (C-2b), 72.0 (OCH2Ph), 71.3 (C-2a), 69.6 (C-4c), 68.7 (C-1OCH2Ph), 68.6 (C-4b), 68.2 (C-6a), 61.5 (C-6b), 61.2 (C-6c). Elemental analysis: calcd for C46H56O16 C 63.88%, H 6.53%; found C 63.33%, H 6.42%.
β-D-glucopyranosyl-(1→3)-β-D-glucopyranosyl-(1→6)-(α,β)-D-mannopyranose 3: to a solution of 13 (200 mg, 0.231 mmol) in 1:1 ethanol/water (15 mL) were successively added acetic acid (100 μL) and palladium acetate (40 mg). After vigorous stirring under hydrogen atmosphere for 48 h, the media was filtered over celite, concentrated and coevaporated with absolute ethanol. This afforded 3 (120 mg, 100%) as a white foam and as an anomeric mixture (α/β =3:1). 1H RMN (D2O, 400 MHz) δ (ppm): 5.08 (s, 1H, H-1aα), 4.83 (m, 0.3H, H-1aβ), 4.66 (d, 1.3H, H-1c, JH1-H2 =7.7 Hz), 4.46 (d, 0.3H, H-1bβ, JH1-H2 =7.7 Hz), 4.45 (d, 1H, H-1bα, JH1-H2 =7.8 Hz), 4.13-4.06 (m, 1.3H, H-6a), 3.91-3.72 (m, 7.8H, H-2a, H-4a, H-5a, H-6’a, H-6b, H-6c), 3.71-3.52 (m, 5.2H, H-3a, H-3b, H-6’b, H-6’c), 3.50-3.22 (m, 9.1H, H-2b, H-4b, H-5b, H-2c, H-3c, H-4c, H-5c). 13C RMN (D2O, 100 MHz) δ (ppm): 103.1 (C-1c), 102.8 (C-1b), 94.4 (C-1aα), 94.1 (C-1aβ), 84.5 (C-3b), 76.3 (C-5c), 75.8 (2C, C-3c, C-5b), 73.8 (C-2c), 73.2 (C-2b), 71.7 (C-4aα), 71.5 (C-4aβ), 70.9 (C-2aα), 70.6 (C-2aβ), 70.4 (2C, C-5aα, C-5aβ), 69.9 (C-4c), 69.3 (C-6a), 68.5 (C-4b), 66.9 (C-3aα), 66.7 (C-3aβ), 61.0 (2C, C-6b, C-6c). HRMS (ESI+): calcd m/z for [M+Na]+ C18H32NaO16 527.1588, found m/z 527.1580; calcd for [M+K]+ C18H32KO16 543.1327, found m/z 543.1334.
β-D-glucopyranosyl-(1→3)-β-D-glucopyranosyl-(1→1)-D-mannitol 1: to a solution of 3 (35.5 mg, 0.070 mmol) in 5:1 CH3OH/H2O (5 mL) was added sodium borohydride (27 mg, 0.714 mmol). After stirring for 6 d at RT, adding few drops of acetic acid allowed neutralization. The resulting mixture was concentrated under reduced pressure and successively coevaoprated with 5:1 CH3OH/acetic acid, and CH3OH. The resulting compound 1 was chemically purified after acetylation (acetic anhydride: 5 mL, 0.053 mmol, pyridine: 5 mL, 48 h, RT). At the end of the reaction, the solvent was removed under reduced pressure and the crude oil diluted in CH2Cl2 (20 mL). This organic layer was washed with 10% HCl aq sol, and sat aq NaHCO3, the dried over MgSO4, and finally concentrated. The peracetylated compound was chromatographied [light petroleum/AcOEt (2:3, v/v)]. The deprotection step involved dissolution of the resulting derivative in 5:1:1 CH3OH/NEt3/H2O mixture (7 mL), stirring for 20 h at RT, and freeze drying, so that 1 was isolated as a white foam (34 mg, 100%). 1H RMN (D2O, 400 MHz) δ (ppm): 4.67 (d, 1H, H-1c, JH1-H2 =7.8 Hz), 4.46 (d, 1H, H-1b, JH1-H2 =8.0 Hz), 4.11 (dd, 1H, H-6a, JH5-H6 =1.8 Hz, JH6-H6’ =10.6 Hz), 3.88-3.56 (m, 12H, H-1a, H-1’a, H-2a, H-3a, H-3b, H-4a, H-5a, H-6b, H-6c, H-6’a, H-6’b, H-6’c), 3.48-3.37 (m, 5H, H-2b, H-3c, H-4b, H-5b, H-5c), 3.32 (t, 1H, H-4c, JH3-H4 = JH4-H5 =9.0 Hz), 3.27 (dd, 1H, H-2c, JH2-H3 =9.3 Hz). 13C RMN (D2O, 100 MHz) δ (ppm): 103.1 (C-1c), 103.0 (C-1b), 84.6 (C-3b), 76.3 (C-5c), 75.9, 75.8 (C-3c, C-5b), 73.8 (C-2c), 73.3 (C-2b), 72.2 (C-6a), 71.1 (C-2a), 70.0 (C-5a), 69.9 (C-4c), 69.4 (C-3a), 69.3 (C-4a), 68.5 (C-4b), 63.5 (C-1a), 61.0 (C-6b, C-6c). HRMS (ESI+): calcd m/z for [M+Na]+ C18H34NaO16: 529.1745, found 529.1739; calcd m/z for [M+K]+ C18H34KO16: 545.1484, found 545.1476; calcd m/z for [M-H+2Na]+ C18H33Na2O16 551.1564, found 551.1570.
Benzyl 4,6-O-benzylidene-2-O-levulinoyl-β-D-glucopyranoside 16: to a solution of 14 (1.1 g, 1.844 mmol) in CH2Cl2/CH3OH (4:1, v/v) at RT was added DDQ (810 mg, 3.568 mmol). After stirring for 5 h, the media was diluted with CH2Cl2 (50 mL) and washed twice by sat aq NaHCO3. The organic layer was then dried (MgSO4), the solvent removed under reduced pressure and the resulting crude subjected to chromatography [light petroleum/AcOEt (3:1, v/v)] to yield the desired acceptor 16 (690 mg, 82%) as a white solid. mp 122 °C. Rf (light petroleum/AcOEt, 3:1) 0.2. [α]20D –62.2 (c =1.0, CH2Cl2). 1H RMN (CDCl3, 400 MHz) δ (ppm): 7.50-7.48 (m, 2H, H arom), 7.38-7.28 (m, 8H, H arom), 5.55 (s, 1H, CHO2Ph), 4.99 (dd, 1H, H-2, JH1-H2 =8.0 Hz, JH2-H3 =9.1 Hz), 4.89 (d, 1H, C-1OCH2Ph, J =12.3Hz), 4.62 (d, 1H, C-1OCH2Ph), 4.56 (d, 1H, H-1), 4.36 (dd, 1H, H-6a, JH5-H6a =4.8 Hz, JH6a-H6b =10.4 Hz), 3.86 (t, 1H, H-4, JH3-H4 = JH4-H5 =8.0 Hz), 3.80 (t, 1H, H-6b, JH5-H6b =10.4 Hz), 3.61 (t, 1H, H-3), 3.42 (dt, 1H, H-5), 3.05 (s, 1H, OH-3), 2.79-2.73 (m, 2H, CH2COCH3), 2.59-2.51 (m, 2H, CH2CO2), 2,16 (s, 3H, CH3). 13C RMN (CDCl3, 100 MHz) δ (ppm): 207.1 (COCH3), 172.0 (CO2), 136.9 (C quat arom), 129.2, 128.4, 128.3, 127.9, 127.7, 126.3 (C arom), 101.9 (CHO2Ph), 99.8 (C-1), 80.5 (C-4), 74.5 (C-2), 72.0 (C-3), 70.8 (CH2Ph), 68.5 (C-6), 66.2 (C-5), 38.1 (CH2COCH3), 29.8 (CH3), 28.0 (CH2CO2). Elemental analysis: calcd for C25H28O8: C 65.78%, H 6.18%; found C 66.04%, H 6.23%.
Benzyl 2-O-benzoyl-4,6-O-benzylidene-3-O-(2-methylnaphtyl)-β-D-glucopyranosyl-(1→3)-4,6-O-benzylidene-2-O-levulinoyl-β-D-glucopyranoside 18: to a suspension of molecular sieves 4Å (100 mg) in CH2Cl2 cooled at –60 °C were successively added the donor 17 (1.73 g, 3.108 mmol), NIS (763 mg, 3.390 mmol), and TMSOTf (50 µL, 0.283 mmol). The reaction was stirred for 3 h before neutralizing by few drops of NEt3. After filtration and removal of the solvent, a chromatographic purification [toluene/AcOEt (9:1, v/v)] gave 18 as a white solid (2.51 g, 94%). mp 178-179 °C. [α]20D –21.0 (c =1.0, CH2Cl2). Rf (light petroleum/AcOEt, 3:1) 0.2. 1H RMN (CDCl3, 400 MHz) δ (ppm): 7.90-7.86 (m, 2H, H arom), 7.70-7.67 (m, 1H, H arom), 7.60-7.21 (m, 24H, H arom), 5.53 (s, 1H, CHbO2Ph), 5.28 (s, 1H, CHaO2Ph), 5.26 (t, 1H, H-2b, JH1-H2 = JH2-H3 =7.1 Hz), 5.06 (dd, 1H, H-2a, JH1-H2 =7.8 Hz, JH2-H3 =8.9 Hz), 4.94 (d, 1H, CH2 NAP, J =12.1 Hz), 4.93 (d, 1H, H-1b), 4.84 (d, 1H, CH2 NAP), 4.80 (d, 1H, C-1OCH2Ph, J =12.2 Hz), 4.52 (d, 1H, C-1OCH2Ph), 4.45 (d, 1H, H-1a), 4.35 (dd, 1H, H-6a, JH5-H6 =4.9 Hz, JH6-H6’ =10.4 Hz), 4.13 (dd, 1H, H-6b, JH5-H6 =4.9 Hz, JH6-H6’ =10.4 Hz), 3.93 (t, 1H, H-3a, JH3-H4 =8.9 Hz), 3.95 (t, 1H, H-4b, JH3-H4 = JH4-H5 =9.1 Hz), 3.85 (dd, 1H, H-3b), 3.81 (t, 1H, H-6’a), 3.80 (t, 1H, H-4a, JH4-H5 =8.9 Hz), 3.44 (dd, 1H, H-5a), 3.43 (ddd, 1H, H-5b, JH5-H6’ =10.4 Hz), 3.68 (t, 1H, H-6’a), 2.66-2.46 (m, 2H, CH2CO2), 2.40-2.27 (m, 2H, CH2COCH3), 2.08 (s, 3H, CH3). 13C RMN (CDCl3, 100 MHz) δ (ppm): 206.4 (COCH3), 171.1 (CO2CH2), 165.0 (OCOPh), 137.3, 137.0, 136.7, 135.3, 133.1, 133.0 (C quat arom), 132.8, 129.7, 129.4, 128.9, 128.3, 128.2, 127.9, 127.8, 127.7, 127.5, 126.7, 126.1, 126.0, 125.8, 125.6 (C arom), 101.7 (CbHO2Ph), 100.9 (CaHO2Ph), 100.1 (C-1b), 99.8 (C-1a), 80.7 (C-4b), 79.7 (C-4a), 78.1 (C-3b), 77.5 (C-3a), 74.0 (C-2b), 73.4 (CH2 NAP), 72.9 (C-2a), 70.6 (C-1OCH2Ph), 68.6 (C-6a, C-6b), 66.3 (C-5a), 65.9 (C-5b), 37.8 (CH2COCH3), 29.7 (CH3), 27.5 (CH2CO2). Elemental analysis: calcd for C56H54O14: C 70.72%, H 5.72%; found C 70.89%, H 5.72%.
Benzyl 2-O-benzoyl-4,6-O-benzylidene-β-D-glucopyranosyl-(1→3)-4,6-O-benzylidene-2-O-levulinoyl-β-D-glucopyranoside 19: DDQ (820 mg, 3.6 mmol) was added to a solution of 18 (1.71 g, 1.8 mmol) in a 4:1 CH2Cl2/CH3OH mixture. After stirring for 16 h at RT, the media was diluted in CH2Cl2, washed with sat aq NaHCO3, and water. The organic layer was then dried (MgSO4) and concentrated under reduced pressure. A chromatographic purification afforded 19 as a white solid. mp 214 °C. Rf (light petroleum/AcOEt, 2:1) 0.3. [α]20D –43.0 (c =1.0, CH2Cl2). 1H RMN (CDCl3, 400 MHz) δ (ppm): 8.06-8.01 (m, 2H, H arom), 7.63-7.23 (m, 19H, H arom), 5.57 (s, 1H, CHbO2Ph), 5.31 (s, 1H, CHaO2Ph), 5.14 (dd, 1H, H-2b, JH1-H2 =6.8 Hz, JH2-H3 =7.1 Hz), 5.09 (dd, 1H, H-2a, JH1-H2 =7.5 Hz, JH2-H3 =8.4 Hz), 5.02 (d, 1H, H-1b), 4.83 (d, 1H, C-1OCH2Ph, J =12.2 Hz), 4.55 (d, 1H, C-1OCH2Ph), 4.49 (d, 1H, H-1a), 4.39 (dd, 1H, H-6a, JH5-H6 =4.8 Hz, JH6-H6’ =10.6 Hz), 4.18 (dd, 1H, H-6b, JH5-H6 =5.1 Hz, JH6-H6’ =10.6 Hz), 4.04 (dd, 1H, H-3a, JH3-H4 =9.1 Hz), 3.99 (dd, 1H, H-3b, JH3-H4 =9.3 Hz), 3.89 (t, 1H, H-6’a, JH5-H6’ =10.6 Hz), 3.78 (t, 1H, H-4a, JH4-H5 =9.1 Hz), 3.75 (t, 1H, H-4b, JH4-H5 =9.3 Hz), 3.70 (t, 1H, H-6’b, JH5-H6’ =10.6 Hz), 3.49 (ddd, 1H, H-5a), 3.46 (ddd, 1H, H-5b), 2.72 (s, 1H, OH-3b), 2.65-2.49 (m, 2H, CH2CO2), 2.37-2.25 (m, 2H, CH2COCH3), 2.12 (s, 3H, CH3). 13C RMN (CDCl3, 100 MHz) δ (ppm): 206.4 (COCH3), 171.1 (CO2CH2), 165.7 (OCOPh), 137.0, 136.9, 136.7 (C quat arom), 133.3, 129.8 (C arom), 129.5 (C quat arom), 129.3, 129.1, 128.4, 128.3, 128.2, 128.1, 127.8, 127.6, 126.2. 126,0 (C arom), 101.6 (CbHO2Ph), 101.5 (CaHO2Ph), 100.0 (C-1b), 99.7 (C-1a), 80.5 (C-4b), 79.6 (C-4a), 77.6 (C-3a), 75.6 (C-2b), 72.9 (C-2a), 70.6 (C-1OCH2Ph), 68.6 (C-6a, C-6b), 66.2 (C-5a), 65.8 (C-5b), 37.7 (CH2COCH3), 29.7 (CH3), 27.5 (CH2CO2). Elemental analysis: calcd for C45H46O14: C =66.66%, H =5.72%; found C =66.53%, H =5.80%.
Benzyl 2-O-benzoyl-4,6-O-benzylidene-3-O-(2-methylnaphtyl)-β-D-glucopyranosyl-(1→3)-2-O-benzoyl-4,6-O-benzylidene-β-D-glucopyranosyl-(1→3)-4,6-O-benzylidene-2-O-levulinoyl-β-D-glucopyranoside 20: to a solution of donor 17 (226 mg, 0.406 mmol) and acceptor 19 (300 mg, 0.370 mmol) in CH2Cl2 cooled at –80 °C, were successively added molecular sieves 4Å, NIS (100 mg, 0.444 mmol) and TMSOTf (6.7 µL, 0.037 mmol). The Lewis acid was neutrlized after stirring for 2 h by adding few drops of NEt3. The media was filtered, concentrated and chromatographied eluting with toluene/AcOEt (9:1, v/v) to give 20 (328 mg, 68%) as a white solid. mp 115 °C. Rf (light petroleum/AcOEt, 2:1) 0.5. [α]20D –4.7 (c =1.0, CH2Cl2). 1H RMN (CDCl3, 400 MHz) δ (ppm): 7.95-7.86 (m, 4H, H arom), 7.70-7.13 (m, 33H, H arom), 5.51 (s, 1H, CHO2Ph), 5.43 (s, 1H, CHO2Ph), 4.94 (d, 1H, CH2 NAP, J =12.2 Hz), 4.83 (d, 1H, CH2 NAP), 4.78 (d, 1H, C-1OCH2Ph, J =12.4 Hz), 4.50 (s, 1H, CHO2Ph), 4.49 (d, 1H, C-1OCH2Ph), 2.69-2.47 (m, 2H, CH2CO2), 2.40-2.21 (m, 2H, CH2COCH3), 1.95 (s, 3H, CH3) and Tables S1 and S2. RMN 13C (CDCl3, 100 MHz) δ (ppm): 206.6 (COCH3), 171.1 (CO2CH2), 165.0, 164.8 (OCOPh), 137.3, 137.1, 137.0, 135.4 (C quat arom), 133.3, 133.1 (C arom), 133.0, 132.8 (C quat arom), 129.8, 129.7 (C arom), 129.4 (C quat arom), 129.4, 128.9, 128.7, 128.6, 128.3, 128.2, 128.1, 127.9, 127.8, 127.7, 127.6, 127.5, 126.6, 126.4, 126.1, 126.0, 125.7, 125.6 (C arom), 102.1 (CHO2Ph), 101.1 (CHO2Ph), 100.9 (CHO2Ph), 73.8 (CH2 NAP), 70.4 (C-1OCH2Ph), 37.7 (CH2COCH3), 29.3 (CH3), 27.6 (CH2CO2) and Table S3. Elemental analysis: cacld for C76H72O20: C =69.93%, H =5.56%; found C =69.89%, H =5.63%.
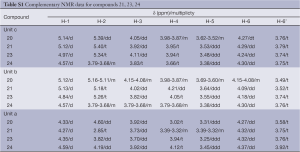
Full table
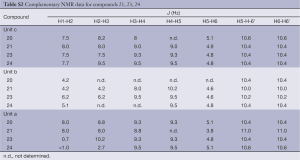
Full table
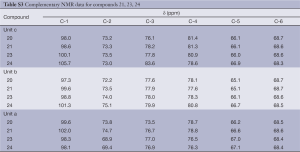
Full table
Benzyl 2-O-benzoyl-4,6-O-benzylidene-3-O-(2-methylnaphtyl)-β-D-glucopyranosyl-(1→3)-2-O-benzoyl-4,6-O-benzylidene-β-D-glucopyranosyl-(1→3)-4,6-O-benzylidene-β-D-glucopyranoside 21: to a solution of 20 (500 mg, 0.383 mmol) in pyridine (7.6 mL) was added 1M hydrazine in pyridine/acetic acid (7.6 mL, 3:2, v/v). After stirring at RT for 3 h, 2,4-pentanedione (2.1 mL) was added and the solvent removed under reduced pressure. The crude was then diluted in CH2Cl2 (50 mL) and washed with 10% aq sol HCl, sat aq NaHCO3, and brine. The organic layer was finally dried (MgSO4), concentrated, and the desired compound 21 purified by chromatography eluting with [light petroleum/AcOEt (2:1, v/v)] to afford a white solid (377 mg, 82%). mp 136 °C. Rf (light petroleum/AcOEt, 2:1) 0.5. [α]20D +13.4 (c =1.0, CH2Cl2). 1H RMN (CDCl3, 400 MHz) δ (ppm): 7.94-7.90 (m, 4H, H arom), 7.68-7.66 (m, 1H, H arom), 7.58-7.19 (m, 32H, H arom), 5.54 (s, 1H, CHO2Ph), 5.52 (s, 1H, CHO2Ph), 4.95 (d, 1H, CH2 NAP, J =12.4 Hz), 4.88 (d, 1H, C-1OCH2Ph, J =11.6 Hz), 4,83 (d, 1H, CH2 NAP), 4.77 (s, 1H, C-1OCH2Ph), 4.53 (d, 1H, C-1OCH2Ph) and Tables S1 and S2. 13C RMN (CDCl3, 100 MHz) δ (ppm): 165.1, 164.7 (2C, OCOPh), 137.3, 137.2, 136.9, 135.3, 133.0, 132.8, 129.2 (8C, C quat arom), 133.4, 133.0, 129.8, 129.7, 129.3 (C arom), 129.2 (C quat arom), 129.0, 128.7, 128.5, 128.4, 128.3, 128.2, 128.0, 127.9, 127.8, 127.5, 126.7, 126.4, 126.1, 126.0, 125.7, 125.6 (C arom), 102.0 (CHO2Ph), 101.2 (CHO2Ph), 100.5 (CHO2Ph), 73.8 (CH2 NAP), 71.2 (CH2 NAP) and Table S3. Elemental analysis: calcd for C71H66O18: C =70.64%, H =5.51%; found C =70.49%, H =5.54%.
Benzyl 2-O-benzoyl-4,6-O-benzylidene-3-O-(2-methylnaphtyl)-β-D-glucopyranosyl-(1→3)-2-O-benzoyl-4,6-O-benzylidene-β-D-glucopyranosyl-(1→3)-4,6-O-benzylidene-β-D-glucopyranos-2-uloside 22: compound 21 (330 mg, 0.273 mmol) was diluted in DMSO/acetic acid (9 mL, 2:1, v/v). After stirring for 48 h at RT, the media was added of CH2Cl2 (50 mL) and then washed with sat aq NaHCO3, and water. After removal of the solvent and coevaopration with toluene, the crude was subjected to column chromatography [light petroleum/AcOEt (3:1, v/v)] to give the expected trisaccharide 22 (180 mg, 55%). Rf (light petroleum/AcOEt, 2:1) 0.3. 1H RMN (CDCl3, 400 MHz) δ (ppm): 7.95-7.91 (m, 4H, H arom), 7.67-7.60 (m, 1H, H arom), 7.57-7.10 (m, 32H, H arom), 5.53 (s, 1H, CHO2Ph), 5.41 (s, 1H, CHO2Ph), 5.40 (dd, 1H, H-2c, JH1-H2 =7.8 Hz, JH2-H3 =8.4 Hz), 5.32 (d, 1H, H-1c), 5.27 (dd, 1H, H-2b, JH1-H2 =2.4 Hz, JH2-H3 =1.6 Hz), 5.19 (d, 1H, H-1b), 4.94 (d, 1H, CH2 NAP, J =11.7 Hz), 4.82 (d, 1H, CH2 NAP), 4.82 (d, 1H, C-1OCH2Ph, J =12.0 Hz), 4.64 (s, 1H, H-1a), 4.61 (d, 1H, C-1OCH2Ph), 4.42 (s, 1H, CHO2Ph), 4.35 (d, 1H, H-3a, JH3-H4 =10.4 Hz), 4.35 (t, 1H, H-6a, JH5-H6 = JH6-H6’ =5.5 Hz), 4.28 (dd, 1H, H-6c, JH5-H6 =4.9 Hz, JH6-H6’ =10.4 Hz), 4.24 (dd, 1H, H-4b, JH3-H4 =7.5 Hz, JH4-H5 =10.6 Hz), 4.11 (dd, 1H, H-6b, JH5-H6 =4.8 Hz, JH6-H6’ =10.2 Hz), 4.01 (dd, 1H, H-3b), 3.95 (t, 1H, H-3c, JH3-H4 =8.4 Hz), 3.91 (t, 1H, H-4c, JH4-H5 =8.4 Hz), 3.77 (t, 1H, H-6’c, JH5-H6’ =10.4 Hz), 3.72 (ddd, 1H, H-5b, JH5-H6’ =10.2 Hz), 3.63 (ddd, 1H, H-5c), 3.56 (t, 1H, H-6’a, JH5-H6’ =5.5 Hz), 3.55 (dt, 1H, H-5a, JH4-H5 =9.0 Hz), 3.41 (t, 1H, H-6’b), 2.98 (dd, 1H, H-4a). 13C RMN (CDCl3, 100 MHz) δ (ppm): 165.2, 165.0 (OCOPh), 137.3, 137.2, 136.8, 135.9, 135.4 (C quat arom), 133.5, 133.3 (C arom), 133.0, 132.8 (C quat arom), 129.8, 129.7, 129.3, 129.2 (C arom), 129.0 (C quat arom), 129.0, 128.9, 128.7, 128.5, 128.4, 128.3, 128.2, 128.1, 128.0, 127.9, 127.8, 127.5, 126.7, 126.4, 126.2, 126.1, 126.0, 125.7, 125.6 (C arom), 101.6 (CHO2Ph), 101.2 (CHO2Ph), 100.6 (CHO2Ph), 98.6 (C-1a), 97.6 (C-1c), 96.1 (C-1b), 81.6 (C-4c), 79.9 (C-4a), 78.1 (C-3c), 77.4 (C-4b), 75.9 (C-3a), 75.8 (C-3b), 73.9 (CH2 NAP), 73.1 (C-2c), 71.8 (C-2b), 70.3 (1OCH2Ph), 68.9 (C-6b), 68.7 (C-6c), 68.3 (C-6a), 66.4 (C-5a), 66.2 (C-5c), 64.9 (C-5b). HRMS (ESI+): calcd m/z for [M+Na]+ C71H64NaO18 1,227.3990, found m/z 1,227.3988; calcd m/z for [M+Na+CH3OH]+ C72H68NaO19 1,259.4252, found m/z 1,259.4245.
Benzyl 2-O-benzoyl-4,6-O-benzylidene-3-O-(2-methylnaphtyl)-β-D-glucopyranosyl-(1→3)-2-O-benzoyl-4,6-O-benzylidene-β-D-glucopyranosyl-(1→3)-4,6-O-benzylidene-β-D-mannopyranoside 23: to a solution of uloside 22 (180 mg, 0.149 mmol) in CH2Cl2/THF (1:1, v/v) cooled at –78 °C was added L-Selectride (1 M in THF, 150 μL, 0.15 mmol). After stirring for 45 min, few drops of acetic acid were added to neutralize the media. At RT, the mixture was diluted CH2Cl2 (30 mL), washed with 10% aq HCl, sat aq NaHCO3, and brine. The resulting layer was finally dried over MgSO4 and concentrated under reduced pressure to afford the mannoside 23 as a white solid (180 mg, 100%). mp 180 °C. Rf (light petroleum/AcOEt, 2:1) 0.2. [α]20D –4.4 (c =1.0, CH2Cl2). 1H RMN (CDCl3, 400 MHz) δ (ppm): 7.85-7.83 (m, 2H, H arom), 7.73-7.67 (m, 3H, H arom), 7.62-7.17 (m, 32H, H arom), 5.50 (s, 1H, CHO2Ph), 5.42 (s, 1H, CHO2Ph), 5.21 (s, 1H, CHO2Ph), 4.90 (d, 1H, CH2 NAP, J =12.4 Hz), 4.87 (d, 1H, C-1OCH2Ph, J =12.2 Hz), 4.80 (d, 1H, CH2 NAP), 4.59 (d, 1H, C-1OCH2Ph) and Tables S1 and S2. RMN 13C (CDCl3, 100 MHz) δ (ppm): 164.8, 164.8 (OCOPh), 137.2, 137.1, 136.5, 135.2 (C quat arom), 133.2 (C arom), 132.9, 132.8 (C quat arom), 132.8, 129.7, 129.6 (C arom), 129.4, 129.1 (C quat arom), 129.0, 128.9, 128.7, 128.5, 128.4, 128.3, 128.2, 128.1, 128.0, 127.8, 127.5, 126.7, 126.4, 126.3, 126.1, 126.0, 125.7, 125.6 (C arom), 101.5 (CHO2Ph), 101.1 (CHO2Ph). 73.5 (CH2 NAP), 70.4 (C-1OCH2Ph) and Table S3.
Benzyl 4,6-O-benzylidene-3-O-(2-methylnaphtyl)-β-D-glucopyranosyl-(1→3)-4,6-O-benzylidene-β-D-glucopyranosyl-(1→3)-4,6-O-benzylidene-β-D-mannopyranoside 24: to a solution of 23 (170 mg, 0.141 mmol) in CH3OH/CH2Cl2 (2:1, v/v, 15 mL) was added sodium methylate in methanol (0.1 M, 3 mL, 0.3 mmol). After stirring for 6 h at RT, neutralization by Amberlite IR-120 (H+-form) afforded a suspension which was then filtered and concentrated. Purification by chromatography [CH2Cl2/C (99:1, v/v)] gave the desired mannoside 24 as a white solid (126 mg, 72%). mp >230 °C. Rf (CH2Cl2/MeOH, 97:3) 0.4. [α]20D –43.5 (c =1.0, CH2Cl2). 1H RMN (CDCl3, 400 MHz) δ (ppm): 7.70-7.19 (m, 27H, H arom), 5.47 (s, 1H, CHO2Ph), 5.44 (s, 1H, CHO2Ph), 5.42 (s, 1H, CHO2Ph), 4.96 (d, 1H, CH2 NAP, J =12.0 Hz), 4.88 (d, 1H, CH2 NAP), 4.84 (d, 1H, C-1OCH2Ph, J =11.9 Hz), 4.56 (d, 1H, C-1OCH2Ph) and Tables S1 and S2. RMN 13C (CDCl3, 100 MHz) δ (ppm): 137.1, 137.0, 136.9, 136.2, 135.8, 133.1, 132.8 (7C, C quat arom), 129.0, 128.9, 128.8, 128.5, 128.4, 128.3, 128.2, 128.1, 128.0, 127.9, 127.8, 127.6, 127.5, 127.4, 126.8, 126.5, 126.2, 126.1, 126.0, 125.9, 125.8, 125.7 (C arom), 101.6 (CHO2Ph), 101.2 (CHO2Ph), 100.7 (CHO2Ph), 74.4 (CH2 NAP), 70.7 (C-1OCH2Ph) and Table S3. HRMS (ESI+): calcd m/z for [M+Na]+ C57H58NaO16 1,021.3623, found m/z 1,021.3624; calcd m/z for [M+K]+ C57H58KO16 1,037.3362, found m/z 1,037.3370; calcd m/z for [M-H+2Na]+ C57H57Na2O16 1,043.3442, found m/z 1,043.3463.
β-D-glucopyranosyl-(1→3)-β-D-glucopyranosyl-(1→3)-(α,β)-D-mannopyranose 4: compound 24 (90 mg, 0.090 mmol)was first dissolved in AcOEt/CH3OH/CH2Cl2 (2:2:1, v/v/v, 10 mL) before adding palladium acetate (60 mg, 0.267 mmol). The suspension was vigorously stirred at RT under hydrogen atmosphere for 7 d, and then filtered over a pad of celite. The hydroorganic layer was extracted with CH2Cl2, concentrated, coevaoprated with ethanol, and subjected to size exclusion chromatography (Sephadex G-10, water) to give 4 in a quantitative yield as a white foam. 1H RMN (D2O, 400 MHz) δ (ppm): 5.13 (d, 1H, H-1aα, JH1-H2 =1.8 Hz), 4.67 (d, 1H, H-1c, JH1-H2 =7.7 Hz), 4.53 (d, 1H, H-1b, JH1-H2 =8.0 Hz), 4.03 (dd, 1H, H-2a, JH2-H3 =3.1 Hz), 3.96 (dd, 1H, H-3a, JH3-H4 =9.5 Hz), 3.90-3.80 (m, 3H, H-6a, H-6b, H-6c), 3.80-3.73 (m, 1H, H-4a), 3.72-3.55 (m, 5H, H-5a, H-6’a, H-3b, H-6’b, H-6’c), 3.53-3.36 (m, 5H, H-2b, H-4b, H-5b, H-3c, H-5c), 3.32 (t, 1H, H-4c, JH3-H4 = JH4-H5 =9.7 Hz), 3.27 (dd, 1H, H-2c, JH2-H3 =9.3 Hz). 13C RMN (D2O, 100 MHz) δ (ppm): 103.1 (C-1c), 100.5 (C-1b), 94.1 (C-1aα), 84.5 (C-3b), 78.5 (C-3a), 76.3 (C-5c), 75.9 (C-3c, C-5b), 75.8 (C-2c), 73.1 (C-2b), 72.7 (C-4a), 69.9 (C-4c), 68.6 (C-2a), 68.4 (C-4b), 65.5 (C-5a), 61.2, 61.0 (C-6a, C-6b, C-6c). HRMS (ESI+): calcd m/z for [M+Na]+ C18H32NaO16 527.1588, found m/z 527.1587; calcd for [M+K]+ C18H32KO16 543.1327, found m/z 543.1343.
β-D-glucopyranosyl-(1→3)-β-D-glucopyranosyl-(1→3)-D-mannitol 2: to a solution of trisaccharide 4 (25 mg, 0.049 mmol) in CH3OH/H2O (4:1, v/v, 10 mL) was added the reducing agent sodium borohydride (19 mg, 0.502 mmol). After stirring at RT for 10 d, neutralization was performed with few drop of acetic acid. The resulting solution was concentrated under reduced pressure, coevaoprated successively with CH3OH/ aceti acid, and CH3OH, and finally freeze-dried. Size exclusion chromatography eluting with water (Sephadex G-10) gave 2 (23 mg) as a white foam. 1H RMN (D2O, 400 MHz) δ (ppm): 4.82 (d, 1H, H-1c, JH1-H2 =8.0 Hz), 4.65 (d, 1H, H-1b, JH1-H2 =8.1 Hz), 4.09-3.90 (m, 7H, H-1a, H-2a, H-3a, H-5a, H-6a, H-6b, H-6c), 3.86-3.66 (m, 6H, H-1’a, H-4a, H-6’a, H-3b, H-6’b, H-6’c), 3.64-3.38 (m, 7H, H-2b, H-4b, H-5b, H-2c, H-3c, H-4c, H-5c). 13C RMN (D2O, 100 MHz) δ (ppm): 103.1 (C-1c), 102.5 (C-1b), 84.4 (C-3b), 77.3 (C-3a), 76.3 (C-5c), 75.8 (C-3c), 75.5 (C-5b), 73.7 (C-2c), 73.4 (C-2b), 70.9, 70.8 (C-2a, C-5a), 69.9 (C-4c), 69.6 (C-4a), 68.8 (C-4b), 63.4 (C-6a), 62.6 (C-1a), 61.2, 61.0 (C-6b, C-6c). HRMS (ESI+): calcd m/z for [M+Na]+ C18H34NaO16: 529.1745, found 529.1743; calcd m/z for [M+K]+ C18H34KO16: 545.1484, found 545.1480; calcd m/z for [M-H+2Na]+ C18H33Na2O16 551.1564, found 551.1561.
References
- Chen J, Seviour R. Medicinal importance of fungal beta-(1→3), (1→6)-Glucans. Mycol Res 2007;111:635-52. [PubMed]
- Bhanja SK, Rout D, Patra P, et al. Structural studies of an immunoenhancing glucan of an ectomycorrhizal fungus Ramaria botrytis. Carbohydr Res 2013;374:59-66. [PubMed]
- Sarkar R, Nandan CK, Bhunia SK, et al. Glucans from alkaline extract of a hybrid mushroom (backcross mating between PfloVv12 and Volvariella volvacea): structural characterization and study of immunoenhancing and antioxidant properties. Carbohydr Res 2012;347:107-13. [PubMed]
- Patra P, Bhanja SK, Sen IK, et al. Structural and immunological studies of heteropolysaccharide isolated from the alkaline extract of Tricholoma crassum (Berk.) Sacc. Carbohydr Res 2012;362:1-7. [PubMed]
- Adams EL, Rice PJ, Graves B, et al. Differential high-affinity interaction of dectin-1 with natural or synthetic glucans is dependent upon primary structure and is influenced by polymer chain length and side-chain branching. J Pharmacol Exp Ther 2008;325:115-23. [PubMed]
- Sletmoen M, Stokke BT. Higher order structure of (1,3)-beta-D-glucans and its influence on their biological activities and comlexation abilities. Biopolymers 2008;89:310-21. [PubMed]
- Takeda Y, Seko A, Sakono M, et al. Parallel quantification of lectin-glycan interaction using ultrafiltration. Carbohydr Res 2013;375:112-7. [PubMed]
- Jamois F, Ferrières V, Guégan JP, et al. Glucan-like synthetic oligosaccharides: iterative synthesis of linear oligo-beta-(1,3)-glucans and immunostimulatory effects. Glycobiology 2005;15:393-407. [PubMed]
- Palma AS, Feizi T, Zhang Y, et al. Ligands for the bata-glucan receptor, dectin-1, assigned using “designer” microarrays of oligosaccharide probes (neoglycolipids) generated from glucan polysaccharides. J Biol Chem 2006;281:5771-9. [PubMed]
- Tanaka H, Kawai T, Adachi Y, et al. Synthesis of β(1,3) oligoglucans exhibiting a Dectin-1 binding affinity and their biological evaluation. Bioorg Med Chem 2012;20:3898-914. [PubMed]
- Józefowski S, Yang Z, Marcinkiewicz J, et al. Scavenger receptors and β-glucan receptors participate in the recognition of yeasts by murine macrophages. Inflamm Res 2012;61:113-26. [PubMed]
- Xiang D, Sharma VR, Freter CE, et al. Anti-tumor monoclonal antibodies in conjunction with β-glucans: a novel anti-cancer immunotherapy. Curr Med Chem 2012;19:4298-305. [PubMed]
- Figueiredo RT, Bittencourt VC, Lopes LC, et al. Toll-like receptors (TLR2 and TLR4) recognize polysaccharides of Pseudallescheria boydii cell wall. Carbohydr Res 2012;356:260-4. [PubMed]
- Vu-Quang H, Muthiah M, Lee HJ, et al. Immune cell-specific delivery of β-glucan-coated iron oxide nanoparticles for diagnosing liver metastasis by MR imaging. Carbohydr Polym 2012;87:1159-68.
- Sylla B, Guégan JP, Wieruszeski JM, et al. Probing β-(1→3)-D-glucans interactions with recombinant human receptors using high resolution NMR studies. Carbohydr Res 2011;346:1490-4. [PubMed]
- Pietrella D, Rachini A, Torosantucci A, et al. A beta-glucan-conjugate vaccine and anti-beta-glucan antibodies are effective against murine vaginal candidiasis as assessed by a novel in vivo imaging technique. Vaccine 2010;28:1717-25. [PubMed]
- Tanaka H, Kawai T, Adachi Y, et al. β(1,3)-Branched heptadeca- and linear hexadeca-saccharides possessing an aminoalkyl group as a strong ligand to dectin-1. Chem Commun (Camb) 2010;46:8249-51. [PubMed]
- LeBlanc BW, Albina JE, Reichner JS. The effect of PGG-beta-glucan on neutrophil chemotaxis in vivo. J Leukoc Biol 2006;79:667-75. [PubMed]
- Li B, Allendorf DJ, Hansen R, et al. Yeast beta-glucan amplifies phagocyte killing of iC3b-opsonized tumor cells via CR3-Syk-PI3-kinase pathway. J Immunol 2006;177:1661-9. [PubMed]
- Tsikitis VL, Albina JE, Reichner JS. Beta-glucan affects leukocyte navigation in a complex chemotactic gradient. Surgery 2004;136:384-9. [PubMed]
- Hong F, Hansen RD, Yan J, et al. Beta-glucan functions as an adjuvant for monoclonal antibody immunotherapy by recruiting tumoricidal granulocytes as killer cells. Cancer Res 2003;63:9023-31. [PubMed]
- Xia Y, Borland G, Huang J, et al. Function of the lectin domain of Mac-1/complement receptor type 3 (CD11b/CD18) in regulating neutrophil adhesion. J Immunol 2002;169:6417-26. [PubMed]
- Xia Y, Vetvicka V, Yan Y, et al. The beta-glucan-binding lectin site of mouse CR3 (CD11b/CD18) and its functionin generating a primed state of the receptor that mediates cytotoxic activation in response to iC3b-opsonized target cells. J Immunol 1999;162:2281-90. [PubMed]
- Thornton BP, Vĕtvicka V, Pitman M, et al. Analysis of the sugar specificity and molecular location of the β-glucan-binding lectin site of complement receptor type 3 (CD11b/CD18). J Immunol 1996;156:1235-46. [PubMed]
- Peat S, Whelan WJ, Lawley HG. The structure of laminarin. Part II. The minor structural features. J Chem Soc 1958;729-37.
- Annan WD, Hirst E, Manners DJ. The constitution of laminarin. Part IV. The minor component sugars. J Chem Soc 1965;220-6.
- Lee RT, Lee YC. Synthesis of some O-D-xylosyl-D-mannoses and their derivatives. Carbohydr Res 1978;67:389-97.
- Shaban MA, Ary IE, Jeanloz DA, et al. The synthesis of 2-acetamido-2-deoxy-6-O-beta-D-mannopyranosyl-D-glucose. Carbohydr Res 1975;45:105-14. [PubMed]
- Ogawa T, Yamamoto H. Synthetic studies on cell-surface glycans. Part XXXIII. Synthesis of a model linear mannohexaose for the backbone structure of fruit body polysaccharide of Tremella fuciformis and Dictyophora indusiata Fisch. Agric Biol Chem 1985;49:475-82.
- Dziewiszek K, Zamojski A. New syntheses of D- and L-glycero-D-manno-heptoses. Carbohydr Res 1986;150:163-72.
- Arnarp J, Lönngren J, Ottosson H. Synthesis of O-β-D-galactopyranosyl-(1,4)-O-(2-acetamido-2-deoxy-β-D-glucopyranosyl)-(1,6)-D-mannopyranose. Carbohydr Res 1981;98:154-6.
- Alais J, Veyrières A. Blood-group li-active oligosaccharides. Synthesis of O-β-D-galactopyranosyl-(1,4)-O-(2-acetamido-2-deoxy-β-D-glucopyranosyl)-(1,6)-D-mannose. Carbohydr Res 1981;92:310-3.
- Lipták A, Jodál I, Nánási P. Stereoselective ring-cleavage of 3-O-benzyl- and 2,3-di-O-benzyl-4,6-O-benzylidenehexopyranoside derivatives with the LiAlH4.AlCl3 reagent. Carbohydr Res 1975;44:1-11.
- Sylla B, Descroix K, Pain C, et al. Double diastereoselection explains limitations in synthesizing mannose-containing beta-(1,3)-glucans. Carbohydr Res 2010;345:1366-70. [PubMed]
- Zeng Y, Ning J, Kong F. Pure alpha-linked products can be obtained in high yields in glycosylation with glucosyl trichloroacetimidate donors with a C2 ester capable of neighboring group participation. Tetrahedron Lett 2002;43:3729-33.
- Moreau V, Viladot JL, Samain E, et al. Design and chemoenzymatic synthesis of thiooligosaccharide inhibitors of 1,3:1,4-beta-D-glucanases. Bioorg Med Chem 1996;4:1849-55. [PubMed]
- Sakairi N, Okazaki Y, Furukawa J, et al. Interglycosidic acetals IV. Preparation and regioselective cleavage of phenyl 2,2':4,6:4',6'-Tri-O-benzylidene-1-thio-β-laminaribiosides. Bull Chem Soc Jpn 1998;71:679-84.
- Ning J, Zhang W, Yi Y, et al. Synthesis of beta-(1→6)-branched beta-(1→3) glucohexaose and its analogues containing an alpha-(1→3) linked bond with antitumor activity. Bioorg Med Chem 2003;11:2193-203. [PubMed]
- Takeo K, Maki K, Wada Y, et al. Synthesis of the laminara-oligosacchairde methyl β-glycosides of dp 3-8. Carbohydr Res 1993;245:81-96. [PubMed]
- Yu H, Williams DL, Ensley HE. 4-Acetoxy-2, 2-dimethylbutanoate: a useful carbohydrate protecting group for the selective formation of β-(1,3)-D-glucans. Tetrahedron Lett 2005;46:3417-21.
- Vetvicka V, Saraswat-Ohri S, Vashishta A, et al. New 4-deoxy-(1→3)-β-D-glucan-based oligosaccharides and their immunostimulating potential. Carbohydr Res 2011;346:2213-21. [PubMed]
- Descroix K, Vetvicka V, Laurent I, et al. New oligo-beta-(1,3)-glucan derivatives as immunostimulating agents. Bioorg Med Chem 2010;18:348-57. [PubMed]
- Saraswat-Ohri S, Vashishta A, Vetvicka V, et al. Biological properties of (1→3)-β-D-glucan-based synthetic oligosaccharides. J Med Food 2011;14:369-76. [PubMed]