The effect of human adipose-derived stem cells on lipopolysaccharide-induced acute respiratory distress syndrome in mice
Introduction
Acute respiratory distress syndrome (ARDS) is an acute respiratory failure in critically ill patients (1). ARDS is characterized by bilateral diffuse alveolar injury, profound inflammation, increased vascular permeability, and alveolar flooding after exposure to a rapid inflammatory reaction that has various causes (1). In the early stages, endogenous pro-inflammatory cytokines, coagulation pathway hyperactivity, or their imbalance could lead to mortality if multi organ failure or circulatory collapse occurs. The extent and duration of these dysfunctions are related to the morbidity and mortality of ARDS (2,3).
ARDS is known to progress pathologically in three stages. The initial exudation stage involves edema and inflammation that develop about a week after lung injury. The subsequent fibroplasia stage lasts for about 4 weeks, and the final fibrosis stage involves the restoration of the lung, accumulation of mature collagen, and progression of pulmonary fibrosis (4). However, recent studies have shown that vigorous collagen synthesis occurs from the beginning of ARDS (5), and a correlation exists between the presence of procollagen peptide III and a fatal outcome (6). Therefore, the processing of fibrosis is considered the early period of ARDS.
Over the past several decades, significant advances have been made in understanding the various causes, mechanisms, and pathophysiology of ARDS owing to several randomized clinical trials (7-9). In particular, low tidal volume ventilation (7), the use of positive end expiratory pressure (8), and conservative fluid management have contributed to the reduction of mortality (9). However, the mortality rate of patients with ARDS is still high at 30–50% (10,11); hence, new therapeutic strategies are needed to reduce the mortality rate. Mesenchymal stem cells (MSCs) are non-hematopoietic stromal cells with low immunogenicity that secrete multiple paracrine factors, including endothelial and epithelial growth factors, anti-inflammatory cytokines, and antimicrobial peptides (12-16). Studies have indicated that the use of MSCs could be a therapeutic modality for ARDS. However, harvesting bone marrow is a painful procedure, and low numbers of MSCs are present in the harvested bone marrow, which can be imposed a load on some patients with severe complications (17).
For clinical application, an alternative source of MSCs is the adipose tissue that is abundant and easily accessible, and yields large numbers of adipose-derived MSCs (ASCs), which are easier to isolate via less-invasive procedures. Compared to other tissues, ASCs can be obtained from a large amount of adipose tissue lipoaspirates and can easily be increased in number, thereby helping secure a large number of MSCs that can produce clinically effective doses (18). In addition, studies have shown that ASCs share immune-enhancing properties with bone marrow-derived stem cells (17-19). However, only a few studies reported adipose-derived stem cells (ASCs), which are easier to isolate, are currently emerging as an attractive treatment option in ARDS and studied acute short term effect only.
The purpose of this study was to investigate whether human ASCs have a therapeutic effect in the short term (day 2) and in long term (day 7) of lipopolysaccharide (LPS)-induced ARDS in a mouse model.
Methods
Mice
C57BL/6 mice (7-week-old males weighing 21–23 g; OrientBio, Gyeonggi-do, Korea) were used in this study. All mouse care and animal experiments were performed in accordance with the protocols approved by the Laboratory of Animal Research of Asan Medical Center (Seoul, Korea, IACUC No. 14-01-027). Monitoring was performed every 24 h (n=5 in all groups).
Purchase and cultivation of stem cells
Human ASCs (StemPRO® Human Adipose-Derived Stem Cells; Thermo Fisher, MA, USA) were purchased and subcultured according to the manufacturer’s manual. Four to five passages of human ASCs were harvested and administered.
ARDS mouse model and ASC transplantation
We divided the mice into four groups: control group; LPS (Escherichia coli, serotype 055:B5; Sigma-Aldrich, MO, USA)-induced ARDS (LPS group); ASCs only (ASC group); and human ASCs following LPS-induced ARDS (LPS/ASC group). Ketamine (70 mg/kg; Yuhan Pharma, Seoul, Korea; Narcotics Handling permission: Seoul 574) and xylazine (9 mg/kg; Bayer Korea Ltd., Seoul, Korea) were used to anesthetize the mice.
In the LPS/ASC group, after the mice were anesthetized, LPS was injected into the trachea at a dose of 5 mg/kg in saline to induce ARDS, and human ASCs (2×105 cells in 0.1 mL of the medium; GibcoTM, MA, USA) were intravenously injected into the tail vein 4 h later. The LPS group received the medium instead of human ASCs. The ASC group was injected with ASCs (2×105 cells in 0.1 mL of the medium) after saline intratracheal (IT) injection IT. The control group was intravenously injected with the medium after saline IT injection.
The short term ARDS model mice were sacrificed 2 days after LPS injection. The long term ARDS fibrosis model mice were injected the same dose of LPS repeatedly on days 0 and 3 (20). They were sacrificed after 7 days.
Tissue and bronchoalveolar lavage fluid (BALF) collection
For tissue harvesting, the lungs were perfused with normal saline. The right hilum was identified, tied off, and removed with the separate lobes, which were frozen immediately in liquid nitrogen and stored at −80 °C. The trachea was then isolated, and using a blunt-tip needle and syringe, the remaining left lung was inflated with 4% paraformaldehyde by using a 25-cm pressure column. The trachea was then tied off and the lung was removed for fixation overnight in formalin, followed by embedding in paraffin. We collected the BALF for cell analysis and cytokine measurement and lung tissue for histological analysis.
Neutrophil count and protein content measurement in the BALF
The protein content of the BALF was measured using the bicinchoninate quantification method. After Diff-Quick staining (Richard-Allan ScientificTM, CA, USA), the number of neutrophils was counted using the method described by Everhart et al. (21). We counted 500 cells in a ×400 field of view under an optical microscope, thereby calculating the cell numbers and neutrophil percentage.
Measurement variable
Tumor necrosis factor (TNF-α), interleukin (IL)-1β, IL-6, and IL-17 were measured using an enzyme-linked immunosorbent assay (ELISA) kit. Myeloperoxidase (MPO) was measured using the DuoSet® ELISA kit. Vascular endothelial growth factor (VEGF), hepatocyte growth factor (HGF), and insulin-like growth factor (IGF)-1 were measured using a Luminex reading program with a Luminex Screening and Performance assay kit (all kits from R&D Systems Inc., MN, USA).
Histological analysis
The fixed lungs were randomly cut, cleaned, dehydrated, embedded in paraffin, cut into 5-µm-thick sections, and examined. The severity of lung injury was determined using these paraffin-embedded lung sections stained with hematoxylin and eosin, and the connective tissue around the cells was observed using Masson’s trichrome staining. Lung injury was assessed by one pathologist who was blinded to the grouping of the mice. Histological variables associated with ARDS were examined, and each variable was given a grade from 0 to 3. The following variables were assessed: (I) intra-alveolar infiltration of neutrophils; (II) interstitial infiltration of neutrophils; (III) perivenous infiltration of neutrophils; (IV) pulmonary congestion; and (V) alveolar hemorrhage (total score, 0–15) (22). Lung fibrosis was evaluated using trichrome-stained lung sections by determining the degree of increased thickness of the interalveolar septa and the presence of fibrotic lesions with total or subtotal distortion of the parenchymal architecture compared with that of the normal architecture (23).
Data processing and analysis
All results are expressed as mean ± standard error of the mean. Differences among groups were assessed using one-way analysis of variance or the Kruskal-Wallis rank analysis of variance. Differences between pairs were assessed using Student’s t-test or the Mann-Whitney test. Statistical analyses were performed using IBM SPSS Statistics for Windows, Version 21.0 (IBM Corp., Armonk, NY, USA), with the significance level set at P<0.05.
Results
ASC treatment attenuated neutrophil infiltration in the lung tissue of the LPS-induced ARDS model at day 2
The lung tissue of the control and ASC groups had normal structures such as alveolar septa, alveolar lumen, and capillary vessels (Figure 1A,B); however, the lung structures were not preserved in the LPS group (Figure 1C). In contrast, the LPS/ASC group showed less neutrophil infiltration and less alveolar hemorrhage or congestion than did the LPS group (Figure 1D). The lung injury scores of the LPS/ASC group were lower than those of the LPS group (3.8±0.9 vs. 6.8±1.1; P=0.03) (Figure 1E).

In the LPS/ASC and LPS groups, the total cell count increased in the BALF at day 2 [(116.3±9.3)×104vs. (133.7±15.5)×104/mL; P=0.22]. Although ASC administration resulted in a reduced total cell count at day 2 compared to those in the LPS group, this did not reach statistical significance. The neutrophil percentage and count in the BALF of the LPS/ASC group showed a decreasing tendency compared to those in the LPS group [66.2%±3.8% vs. 75.3%±2.9%; P=0.05 and (75.7±8.7)×104vs. (101.1±13.8)×104/mL; P=0.09, respectively] (Figure 2).
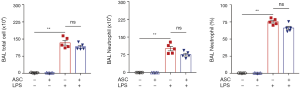
The increase in cell count in the BALF was accompanied by a significant increase in the levels of inflammatory mediators at day 2. Cytokine levels tended to decrease slightly but did not show any statistical significance between the LPS/ASC and LPS groups: TNF-α (73.7±21.7 vs. 102±15.3 pg/mL; P=0.22); IL-1β (55.3±14.5 vs. 47.2±5.4 pg/mL; P=0.55); IL-6 (566.5±253.8 vs. 877±211.5 pg/mL; P=0.15); IGF-1 (2.5±0.8 vs. 3.6±0.8 ng/mL; P=0.39); VEGF (474.4±170.2 vs. 607.7±77.7 pg/mL; P=0.69); HGF (689.9±183.6 vs. 785.9±74 pg/mL; P=0.84); and MPO (1±0.1 vs. 1.2±0.3 ng/mL; P=0.84) (Figure 3).
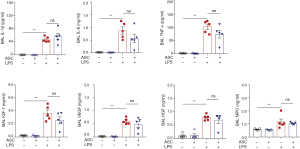
ASC treatment attenuated interstitial fibrosis in the LPS-induced ARDS model at day 7
At day 7, the LPS group showed inflammatory cell infiltration, mild alveolar septa, and interstitial thickening (Figure 4A). However, in the LPS/ASC group, the alveolar septa and alveolar lumen were relatively well preserved, and inflammatory cell infiltration was observed in the alveolar space and interstitium. Interstitial thickening was not observed (Figure 4).
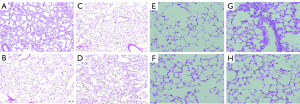
Masson’s trichrome staining showed inflammatory cell infiltration and mild thickening in the alveolar septa and interstitium of the LPS group, with prominent perivascular collagen and suspicion of fibrosis (Figure 5A). However compared to the LPS group, the LPS/ASC group showed reduced collagen deposition around the vessels and fibrosis accompanied by alveolar septal or interstitial thickening (Figure 5).
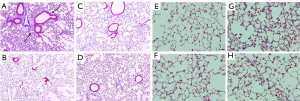
The total cell count in the ASC group was also higher than those in the control group at day 7. The total cell count in the BALF was not different between the LPS/ASC and LPS groups [(24.3±3.7)×104vs. (21±0.6)×104/mL; P=0.13]. The percentage and count of neutrophils were also not different between the LPS/ASC and LPS groups [19.6%±3.4% vs. 19.2%±4.8%; P=0.69 and (4.6±0.8)×104vs. (3.7±1)×104/mL; P=0.31, respectively].
The LPS/ASC group had lower MPO levels than did the LPS group (453.2±26.2 vs. 670.2±65.9 pg/mL; P<0.01). However, the concentration of IL-17 was not significantly different between the LPS/ASC and LPS groups (6.0±0.1 vs. 6.5±0.2 pg/mL; P=0.60) (Figure 6).
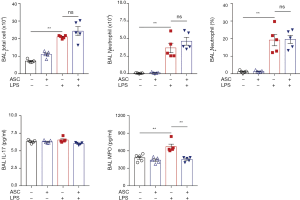
Discussion
In this study, we investigated the effects of human ASCs in an LPS-induced ARDS mouse model, as well as the relevant inflammatory factors and histological changes. The injection of ASCs resulted in a tendency of decrease in the percentage of neutrophils in the BALF and an improvement in histological assessment of lung injury at the short term. In addition, a decrease in MPO was observed in the long term of acute lung injury, as was a decrease in fibrosis on histological analysis. However, the decrease in inflammatory cytokine levels was not significant and the treatment effect was minimal.
The pathogenesis of ARDS involves lung endothelial injury, alveolar epithelial injury, and the accumulation of protein-rich fluid and cellular debris in the alveolar space (1). Among these, LPS-induced acute lung injury is caused by the interactions of IL-1β, IL-6, and TNF-α, which are inflammatory cytokines secreted by LPS-activated neutrophils, alveolar macrophages, and vascular endothelial cells, that are mainly involved in this mechanism (1,24). The mechanism of acute lung injury caused by neutrophils is important, and in this experiment, we observed that the epithelial and vascular barriers were destroyed to induce alveolar flooding. The most important factors in the LPS-induced ARDS model are neutrophils (25,26), which secrete a large amount of reactive oxygen species (27). ARDS reduced when LPS or TNF was administered to animal models with artificially removed neutrophils (25,28,29).
We observed a decrease in neutrophil infiltration in the short term injury model after ASC treatment (day 2); moreover, the slight thickening of the alveolar septum and interstitium was reduced and the deposition of collagen around the veins was less pronounced. In addition, ASC treatment resulted in a tendency of decrease in the neutrophil percentage in the BALF at the initial stage. ASC treatment substantially reduced LPS-induced lung injury and neutrophil infiltration as shown by hematoxylin and eosin staining. Because neutrophil activation and transmigration into the alveolar space and lung interstitium play crucial roles in the development of ARDS (30), reducing neutrophil infiltration by administering ASCs may have a clinically relevant therapeutic potential. Several studies mainly for bone marrow derived stem cell, a few ASC, as summarized in Table 1, have reported the ability of human ASC to reduce inflammation and lung injury. Previous studies for ARDS were done mainly for bone marrow derived stem cells not for ASC and studied only acute effect. There are few studies for effects of ASC and subacute effects of ASC in ARDS model. However, our study did not show any significant effects of human ASCs on cytokines and growth factors, such as IL-1β, IL-6, or TNF-α, and showed only partial effects on acute lung injury in the mouse model of lung injury.
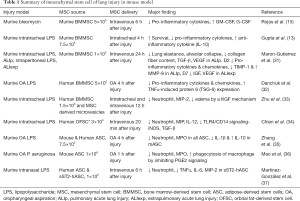
Full table
Zhang et al. showed that the administration of ASCs by oropharyngeal aspiration from mice in the acute lung injury mouse model improved lung injury and inflammatory response, and that anti-inflammatory effects mediated by IL-6 were useful in acute lung injury (35,38). We used human ASCs instead of mouse ASCs; hence, we suggest the use of heterologous ASCs and the different route of ASCs contributed to this discrepancy in our study.
Traditionally, ARDS has been known to progress over time with distinctly different features of inflammation, fibroplasia, and fibrosis, but recent research has shown that these concepts have changed significantly. Fibrosing alveolitis starts from the fibroplasia stage even before the occurrence of ARDS fibrosis (39,40), and the genes involved in inflammation and repair are co-expressed in the very early stage of ARDS (41); moreover, several common mediators are involved in these processes. We observed that MPO concentration in the BALF was significantly lower in the ASC group than in the LPS group in the long term (day 7), where the fibroplasia stage became. In addition, the fibrosis associated with peripheral collagen deposition and the alveolar septa or interstitium was reduced in the ASC group at the time of histological fibroplasia. The reduction in the levels of fibrogenic mediators resulted in improved lung remodeling. This might have an effect on fibrosis.
Fortunately, no significant adverse effects were found in the alveolar tissues. Neither the ASC group nor the control group showed any inflammatory reaction or swelling in the alveoli. However, this does not show an obvious effect of ASCs. This finding suggests that the number of cells required for treatment is unknown, or that pretreatment is required to activate the function of the administered heterologous ASCs (37).
A recent systematic meta-analysis evaluated studies on the efficacy of stem cell administration in animal models of ARDS with preclinical-stage acute lung injury (42). This analysis found that death due to acute lung injury was associated with an odds ratio of 0.24 (95% confidence interval, 0.18–0.34), thereby showing the positive effects of stem cell therapy. However, studies on the administration of heterologous ASCs in acute lung injury models are relatively scarce, and these studies did not yield significant results in the meta-analysis (42). Chien et al. showed that human orbital fat-derived stem cells showed a decrease in lung injury and cytokine levels, which may be associated with their better dividing potential because they are stem cells derived from orbital fat and not subcutaneous adipose tissue (34,43).
Although the several parameters and histology of lung injury and fibrosis show trends in attenuation, there are some limitations in this study. It failed to detect the significant effects of ASCs on inflammatory cytokines and growth factors in mouse lung injury. It might be related to the different MSC delivery by oropharyngeal aspiration, or inadequate MSC dosage. More basically, the relative lack of human MSC-mediated process might be likely to be due to limitations of their immune privilege and differential priming of human MSC in mice.
In terms of the evaluation of lung fibrosis, collagen deposition in this study was not clear, it is needed the detailed and quantitative measurement including total collagen fiber in lung tissue.
However, our study suggests the possibility of human ASC treatment in lung fibrosis including inflammation in ARDS mice model. Further studies are needed to overcome these limitations of interspecies stem cell treatment. Moreover, the current study findings are meaningful in that human ASCs were injected into an LPS-induced acute lung injury mouse model to observe the fibrosis and exudative phases. Although this study did not reveal the role of fibrosis-related mediators, it showed a decrease in fibrosis histologically. Therefore, further studies are warranted to understand the effects of ASCs in ARDS.
Conclusions
Human ASC therapy may inhibit neutrophil recruitment, weaken early lung injury, and even affect fibrosis. However, the effectiveness of the treatment is minimal, and further studies are warranted to understand the mechanism and improve the therapeutic effect of ASCs.
Acknowledgments
Funding: This study was supported by a grant 2014-595 from the Asan Institute for Life Science, Asan Medical Center, Seoul, Korea, and Samyang 2015-643 from the Asan Institute for Life Sciences and Corporate Relations of Asan Medical Center, Seoul, Korea.
Footnote
Conflicts of Interest: The authors have no conflicts of interest to declare.
Ethical Statement: The authors are accountable for all aspects of the work in ensuring that questions related to the accuracy or integrity of any part of the work are appropriately investigated and resolved. The study was approved by the Laboratory of Animal Research of Asan Medical Center (Seoul, Korea, IACUC No. 14-01-027).
References
- Ware LB, Matthay MA. The acute respiratory distress syndrome. N Engl J Med 2000;342:1334-49. [Crossref] [PubMed]
- Osuchowski MF, Welch K, Siddiqui J, et al. Circulating cytokine/inhibitor profiles reshape the understanding of the sirs/cars continuum in sepsis and predict mortality. J Immunol 2006;177:1967-74. [Crossref] [PubMed]
- Monneret G, Venet F, Pachot A, et al. Monitoring Immune Dysfunction in the Septic Patient: A New for the Old Ceremony. Mol Med 2008;14:64-78. [Crossref] [PubMed]
- Tomashefski JF Jr. Pulmonary pathology of acute respiratory distress syndrome. Clin Chest Med 2000;21:435-66. [Crossref] [PubMed]
- Clark JG, Milberg JA, Steinberg KP, et al. Type III procollagen peptide in the adult respiratory distress syndrome. Association of increased peptide levels in bronchoalveolar lavage fluid with increased risk for death. Ann Intern Med 1995;122:17-23. [Crossref] [PubMed]
- Meduri GU, Tolley EA, Chinn A, et al. Procollagen types I and III aminoterminal propeptide levels during acute respiratory distress syndrome and in response to methylprednisolone treatment. Am J Respir Crit Care Med 1998;158:1432-41. [Crossref] [PubMed]
- Acute Respiratory Distress Syndrome Network, Brower RG, Matthay MA, et al. Ventilation with lower tidal volumes as compared with traditional tidal volumes for acute lung injury and the acute respiratory distress syndrome. N Engl J Med 2000;342:1301-8. [Crossref] [PubMed]
- Mercat A, Richard JC, Vielle B, et al. Positive end-expiratory pressure setting in adults with acute lung injury and acute respiratory distress syndrome: a randomized controlled trial. JAMA 2008;299:646-55. [Crossref] [PubMed]
- National Heart, Lung, and Blood Institute Acute Respiratory Distress Syndrome (ARDS) Clinical Trials Network, Wiedemann HP, et al. Comparison of two fluid-management strategies in acute lung injury. N Engl J Med 2006;354:2564-75.
- Rubenfeld GD, Caldwell E, Peabody E, et al. Incidence and outcomes of acute lung injury. New Engl J Med 2005;353:1685-93. [Crossref] [PubMed]
- Villar J, Blanco J, Anon JM, et al. The ALIEN study: incidence and outcome of acute respiratory distress syndrome in the era of lung protective ventilation. Intensive Care Med 2011;37:1932-41. [Crossref] [PubMed]
- Fang X, Neyrinck AP, Matthay MA, et al. Allogeneic Human Mesenchymal Stem Cells Restore Epithelial Protein Permeability in Cultured Human Alveolar Type II Cells by Secretion of Angiopoietin-1. J Biol Chem 2010;285:26211-22. [Crossref] [PubMed]
- Gupta N, Su X, Popov B, et al. Intrapulmonary delivery of bone marrow-derived mesenchymal stem cells improves survival and attenuates endotoxin-induced acute lung injury in mice. J Immunol 2007;179:1855-63. [Crossref] [PubMed]
- Ortiz LA, Gambelli F, McBride C, et al. Mesenchymal stem cell engraftment in lung is enhanced in response to bleomycin exposure and ameliorates its fibrotic effects. Proc Natl Acad Sci U S A 2003;100:8407-11. [Crossref] [PubMed]
- Rojas M, Xu J, Woods CR, et al. Bone marrow-derived mesenchymal stem cells in repair of the injured lung. Am J Respir Cell Mol Biol 2005;33:145-52. [Crossref] [PubMed]
- Krasnodembskaya A, Song YL, Fang XH, et al. Antibacterial Effect of Human Mesenchymal Stem Cells Is Mediated in Part from Secretion of the Antimicrobial Peptide LL-37. Stem Cells 2010;28:2229-38. [Crossref] [PubMed]
- Zuk PA, Zhu M, Mizuno H, et al. Multilineage cells from human adipose tissue: implications for cell-based therapies. Tissue Eng 2001;7:211-28. [Crossref] [PubMed]
- Yañez R, Lamana ML, García-Castro J, et al. Adipose tissue-derived mesenchymal stem cells have in vivo immunosuppressive properties applicable for the control of the graft-versus-host disease. Stem Cells 2006;24:2582-91. [Crossref] [PubMed]
- Dicker A, Le Blanc K, Aström G, et al. Functional studies of mesenchymal stem cells derived from adult human adipose tissue. Exp Cell Res 2005;308:283-90. [Crossref] [PubMed]
- Vernooy JH, Denterner MA, van Suylen RJ, et al. Long-term intratracheal lipopolysaccharide exposure in mice results in chronic lung inflammation and persistent pathology. Am J Respir Cell Mol Biol 2002;26:152-9. [Crossref] [PubMed]
- Everhart MB, Wei H, Sherrill TP, et al. Duration and intensity of NF-kappa B activity determine the severity of endotoxin-induced acute lung injury. J Immunol 2006;176:4995-5005. [Crossref] [PubMed]
- Hong SB, Koh Y, Lee IC, et al. Induced hypothermia as a new approach to lung rest for the acutely injured lung. Crit Care Med 2005;33:2049-55. [Crossref] [PubMed]
- Degryse AL, Tanjore H, Xu XCC, et al. Repetitive intratracheal bleomycin models several features of idiopathic pulmonary fibrosis. Am J Physiol Lung Cell Mol Physiol 2010;299:L442-52. [Crossref] [PubMed]
- Pugin J, Ricou B, Steinberg KP, et al. Proinflammatory activity in bronchoalveolar lavage fluids from patients with ARDS, a prominent role for interleukin-1. Am J Respir Crit Care Med 1996;153:1850-6. [Crossref] [PubMed]
- Abraham E. Neutrophils and acute lung injury. Crit Care Med 2003;31:S195-9. [Crossref] [PubMed]
- Perkins GD, Nathani N, McAuley DF, et al. In vitro and in vivo effects of salbutamol on neutrophil function in acute lung injury. Thorax 2007;62:36-42. [Crossref] [PubMed]
- Iyer SS, Torres-Gonzalez E, Neujahr DC, et al. Effect of Bone Marrow-Derived Mesenchymal Stem Cells on Endotoxin-Induced Oxidation of Plasma Cysteine and Glutathione in Mice. Stem Cells Int 2010;2010:868076. [Crossref] [PubMed]
- Ismail G, Morganroth ML, Todd RF 3rd, et al. Prevention of pulmonary injury in isolated perfused rat lungs by activated human neutrophils preincubated with anti-Mo1 monoclonal antibody. Blood 1987;69:1167-74. [PubMed]
- Stephens KE, Ishizaka A, Wu ZH, et al. Granulocyte Depletion Prevents Tumor Necrosis Factor-Mediated Acute Lung Injury in Guinea-Pigs. Am Rev Respir Dis 1988;138:1300-7. [Crossref] [PubMed]
- Yan C, Wu M, Cao J, et al. Critical Role for CCAAT/Enhancer-Binding Protein in Immune Complex-Induced Acute Lung Injury. J Immunol 2012;189:1480-90. [Crossref] [PubMed]
- Maron-Gutierrez T, Silva JD, Asensi KD, et al. Effects of Mesenchymal Stem Cell Therapy on the Time Course of Pulmonary Remodeling Depend on the Etiology of Lung Injury in Mice. Crit Care Med 2013;41:e319-33. [Crossref] [PubMed]
- Danchuk S, Ylostalo JH, Hossain F, et al. Human multipotent stromal cells attenuate lipopolysaccharide-induced acute lung injury in mice via secretion of tumor necrosis factor-alpha-induced protein 6. Stem Cell Res Ther 2011;2:27. [Crossref] [PubMed]
- Zhu YG, Feng XM, Abbott J, et al. Human mesenchymal stem cell microvesicles for treatment of Escherichia coli endotoxin-induced acute lung injury in mice. Stem Cells 2014;32:116-25. [Crossref] [PubMed]
- Chien MH, Bien MY, Ku CC, et al. Systemic human orbital fat-derived stem/stromal cell transplantation ameliorates acute inflammation in lipopolysaccharide-induced acute lung injury. Crit Care Med 2012;40:1245-53. [Crossref] [PubMed]
- Zhang S, Danchuk SD, Imhof KM, et al. Comparison of the therapeutic effects of human and mouse adipose-derived stem cells in a murine model of lipopolysaccharide-induced acute lung injury. Stem Cell Res Ther 2013;4:13. [Crossref] [PubMed]
- Mao YX, Xu JF, Seeley EJ, et al. Adipose Tissue-Derived Mesenchymal Stem Cells Attenuate Pulmonary Infection Caused by Pseudomonas aeruginosa via Inhibiting Overproduction of Prostaglandin E2. Stem Cells 2015;33:2331-42. [Crossref] [PubMed]
- Martínez-González I, Roca O, Masclans JR, et al. Human Mesenchymal Stem Cells Overexpressing the IL-33 Antagonist Soluble IL-1 Receptor-Like-1 Attenuate Endotoxin-Induced Acute Lung Injury. Am J Respir Cell Mol Biol 2013;49:552-62. [Crossref] [PubMed]
- Zhang S, Danchuk SD, Bonvillain RW, et al. Interleukin 6 mediates the therapeutic effects of adipose derived stromal/stem cells in lipopolysaccharide-induced acute lung injury. Stem Cells 2014;32:1616-28. [Crossref] [PubMed]
- Marshall RP, Bellingan G, Webb S, et al. Fibroproliferation occurs early in the acute respiratory distress syndrome and impacts on outcome. Am J Respir Crit Care Med 2000;162:1783-88. [Crossref] [PubMed]
- Chesnutt AN, Matthay MA, Tibayan FA, et al. Early detection of type III procollagen peptide in acute lung injury. Pathogenetic and prognostic significance. Am J Respir Crit Care Med 1997;156:840-5. [Crossref] [PubMed]
- Wesselkamper SC, Case LM, Henning LN, et al. Gene expression changes during the development of acute lung injury: role of transforming growth factor beta. Am J Respir Crit Care Med 2005;172:1399-411. [Crossref] [PubMed]
- McIntyre LA, Moher D, Fergusson DA, et al. Efficacy of Mesenchymal Stromal Cell Therapy for Acute Lung Injury in Preclinical Animal Models: A Systematic Review. PLoS One 2016;11:e0147170. [Crossref] [PubMed]
- Ho JH, Ma WH, Tseng TC, et al. Isolation and characterization of multi-potent stem cells from human orbital fat tissues. Tissue Eng Part A 2011;17:255-66. [Crossref] [PubMed]