Inhalation of molecular hydrogen prevents ischemia-reperfusion liver damage during major liver resection
Introduction
One of the key mechanisms in the development of liver dysfunction is ischemia-reperfusion damage, which consists of two phases, an initial phase, and a late phase. In the initial phase, which occurs during the first two hours after reperfusion, Kupffer’s cells, stimulated by ischemia, produce a number of reactive oxygen species (ROS) that lead to acute hepatocellular damage. In the late phase, which starts 6 hours after reperfusion, neutrophils, also a well-known source of ROS, accumulate in the liver, and further exacerbate liver damage. Furthermore, disruption of the respiratory chain in the mitochondria of hepatocytes and the endothelial cells of the sinusoids lead to additional formation of ROS in the initial phase of ischemia-reperfusion liver damage (1). In addition, prolonged warm liver ischemia exacerbates the development of oxidative stress during reperfusion; therefore, ischemic-reperfusion damage caused by oxidative stress has received considerable attention in both laboratory and clinical research (2-5).
Although the cause of ischemia-reperfusion damage is multifactorial and certainly involves hypoxia, the inflammatory response and free oxygen radical damage (more formally ROS–see below), and ROS (1,2,4-6).
ROS include both free radicals and substances that are not free radicals—therefore, the term “reactive oxygen species” is more accurate and correct than “free radical”. A free radical is any chemical entity, atom, molecule, or ion that has at least one unpaired electron in its outer electron shell, making it highly reactive and, in so doing, capable of independent existence.
The hydroxyl radical (HO•) is the most reactive ROS, which makes it extremely toxic. It originates in the presence of transition metal ions (Fe2+, Cu+), as a product of the reduction of H2O2 in the Fenton reaction, or from the Haber-Weiss reaction in which superoxide anion (O2•−) reacts with hydrogen peroxide (H2O2) (1). The search for scavengers, which selectively react with the hydroxyl radical, is very important since superoxide anion and hydrogen peroxide are eliminated by natural antioxidant mechanisms—superoxide dismutase, peroxidase, and glutathione peroxidase. Nevertheless, no enzyme can eliminate the hydroxyl radical.
Recent and extensive research in this direction has shown the potential of molecular hydrogen (H2) as a promising antioxidant. Although hydrogen has been known as a gaseous element for approximately 250 years, its ability to react with ROS (more precisely with hydroxyl radicals–HO•) in vivo and in vitro was only discovered in 2007 (7). Molecular hydrogen is able to react selectively with hydroxyl radicals to form water.
Molecular hydrogen is colorless, tasteless, and odorless and is highly explosive in mixtures with oxygen (at concentrations between 4–75% by volume) (8). Safe mixtures with oxygen exist below hydrogen concentrations of 4% by volume; in most experiments, half the concentration (2%) is generally used (7,9). In the body, hydrogen is completely inert and has no toxic effects, even during multi-hour exposure.
Since hydrogen is approximately four times more fat-soluble than water, it penetrates very quickly through all biological membranes, including subcellular organelles. This is its great advantage compared to other antioxidants. During continuous inhalation of a 2% H2 mixture, a concentration of about 8 µmol/L was established in intra- and extracellular fluid compartments after approximately 45 minutes. This concentration is maintained throughout the entire inhalation period. Hydrogen is then eliminated by exhalation with a half-life of 8–10 minutes (8,10-15). Inhalation appears to be the most appropriate method due to the rapid saturation of the organism and the easy maintenance of steady levels during surgical procedures.
From a surgical point of view, the protective effect during large liver resections and liver transplants is particularly important. Liver resection is a high-risk surgery that is associated with severe complications. The most serious is liver failure during the postoperative period, which can be fatal. One of the key mechanisms associated with liver dysfunction is ischemia-reperfusion damage. In light of new information regarding the positive effects of hydrogen in various clinical trials over the last 10 years, it is reasonable to evaluate its potential in a clinically relevant model of hemi-hepatectomy, where the positive effects could be particularly advantageous.
In the previously published studies, small laboratory animals were used almost exclusively, and only selected histological and biochemical parameters were assessed. To date, there have been no published studies using large laboratory animals followed by a comprehensive evaluation of ischemia-reperfusion liver damage using histological and biochemical markers, including markers of oxidative stress, which can be used in experimental research to assess oxidative damage at the molecular level.
Therefore, the aim of this work was to demonstrate the positive antioxidant, anti-inflammatory, and anti-apoptotic effect of inhaled hydrogen on domestic pigs during large liver resection, using histological and biochemical examinations and examination of oxidative damage markers.
Methods
Model of ischemia-reperfusion liver damage
The model used a total of 12 domestic female pigs (Sus scrofa f. domestica), weighing 30–35 kg. All animals received humane care according to the criteria outlined in the Guide for the Care and Use of Laboratory Animals prepared by the National Academy of Sciences and published by the National Institutes of Health (NIH publication 86-23 revised 1985). Animal health certificates were delivered, the animals were completely healthy, with no signs of infectious disease, and they were examined by a vet prior to inclusion in the study. The animals were kept under standard conditions. Before the experiments, the animals were first acclimated to reduce the influence of transport and handling stress on the results of the study.
The 12 animals were randomly divided into two groups, 6 animals underwent the surgical procedure, which included inhaled hydrogen during general anesthesia. The second group of 6 animals underwent the same procedure without inhaled hydrogen and served as the control group.
General anesthesia
The animals were pre-treated with an intramuscular mixture of ketamine (Narkamon, Spofa, Czech Republic) at a dose of 30 mg/kg of live weight, azaperone (Stresnil, Janssen Pharmaceutics, Belgium) at a dose of 40 mg/kg, and atropine (Atropin Biotics A.U. V, Biotics, Slovakia) at a dose of 0.05 mg/kg of live weight. The animals were then cannulated to the ear vein, and general anesthesia was initiated using propofol (Propofol, Fresenius Kabi, Bad Homburg, Germany) at a dose of 2 mg/kg until the onset of effect. Next, the animals were intubated with a tracheal cannula (size 7.5), and maintained in total inhalation anesthesia with a mixture of isoflurane (Aerrane, Baxter S.A., Lessines, Belgium) +40% O2 +58–60% N2 (+2% molecular hydrogen was added to the intervention group). In addition, and under ultrasound control, the femoral artery was cannulated (Arrow 18G, length 16 cm, Teleflex, Athlon, Co. Westmeath, Ireland) for sampling and peri-operative monitoring. During anesthesia, the animals received pancuronium (Arduan, Gedeon RichterPlc., Budapest, Hungary), as a muscle relaxant, at a dose of 0.05 mg/kg (initial bolus), and then maintained with 0.01 mg/kg/hour continuous drip. At the end of the experiment, the animals were painlessly euthanized (using intravenous T61, Intervet International B.V., Boxmeer, The Netherlands).
Hydrogen inhalation
The animals in the intervention group were given a well-defined mixture of oxygen, nitrogen, and hydrogen in pressure cylinders supplied by the SIAD Czech Inc. (Prague, Czech Republic) in a ratio of 40% O2 +58% N2 +2% H2, from induction of general anesthesia throughout the entire period of surgery and until the end of the experiment. The gas mixture was supplied to the anesthesia machine using a compressed air inlet, and the oxygen supply was switched off. The gas flow into the closed-circuit machine was set to 1 liter per minute. This mixture was safe and explosion-proof even in high-pressure bottles. This approach ensured a concentration of dissolved hydrogen of about 8–10 µmol/L in all body tissues according to previous research (Ono et al. 2012) (16).
Surgery
Intraoperatively, a middle laparotomy and preparation of the left branch of the hepatic portal vein and the left hepatic artery were carried out, and a tourniquet was applied to both vessels, thus achieving complete warm ischemia of the left lobes of the liver. Ischemia was verified visually by a change in the liver color to dark purple. The duration of warm ischemia was 120 minutes. Then the tourniquet was released, followed by reperfusion of the liver for another 120 minutes. Thereafter, samples of liver tissue from ischemic and non-ischemic halves of the liver were taken, and the surgery was terminated.
Sampling
In the course of the experiment, each animal had blood taken from the femoral artery four times, i.e., (I) during induction of general anesthesia before intubation; (II) before the hepatoduodenal ligament clamp; (III) after 2 hours of hepatic parenchyma ischemia; and (IV) after 2 hours of reperfusion. For each blood collection, 6 mL of blood was withdrawn into a lavender-topped tube (with EDTA) and 3.5 mL of blood into a gold-topped SST (serum-separating tube).
Blood sampling
The EDTA tube was spun at 4,150 rpm for 10 min on a HERMLE Z206A centrifuge (Hermle AG, Gosheim, Germany), and then, with the pipette, 0.25 mL of plasma was withdrawn to test for lactate, and 1.5 mL of plasma was used to test for markers of oxidative stress. The plasma was initially frozen at −18 °C. Some plasma was examined for lactate, and the remainder was stored in a freezer at −80 °C; markers of oxidative stress were later measured in bulk.
The blood-clotting tube was spun in the same way, cooled, and subsequently, the values of ALT, AST, ALP, GMT, LD, total protein, and albumin were determined. In addition, based on research by Vaubourdolle et al. [1993], we determined the level of creatine kinase, subtype BB. In the article, the authors state that sinusoid damage plays a key role in primary liver dysfunction after liver transplantation. However, since there is no simple biochemical marker for this damage, it is suggested that the levels of creatine kinase BB can be used, which is mainly found in the heart endothelium, and to a lesser extent in sinusoid endothelium, and Kupffer (stellate macrophages) and hepatic stellate (Ito) cells. BB creatine kinase is not present in hepatocytes, which contain mitochondrial creatine kinase (17).
Collection of liver tissue
At the end of reperfusion, liver samples from the ischemic (left) and non-ischemic (right) liver lobe were taken. Half of the samples from each lobe were immediately frozen in liquid nitrogen at a temperature of about −196 °C; subsequently, the samples were used to measure markers of oxidative stress. The other half of the samples were fixed in 10% formaldehyde solution and subsequently processed for histological analysis.
Histological examination
After fixation in formalin, the samples of liver tissue were embedded in paraffin. Paraffin cuts of 4 micrometers were colored with hematoxylin-eosin and observed under a light microscope. The degree of ischemia-reperfusion liver tissue damage was evaluated in individual sections using the Suzuki classification (18,19), in which the extent of sinusoidal congestion, vacuolation (balloon degeneration), and hepatocyte necrosis were expressed as a numerical score from 0 to 4, which allowed liver tissue damage to be assessed semi-quantitatively (see Table 1).
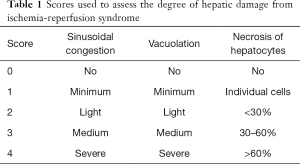
Full table
Examination of oxidative damage markers
Malondialdehyde (MDA) is a widely recognized marker of oxidative damage. It is an indicator of lipid peroxidation by hydroperoxyl and hydroxyl radicals. Additionally, 3-nitrotyrosine (3-NT) can be used as a marker of protein nitration by reactive forms of nitrogen. We were also able to measure oxidative damage indirectly by measuring the activity of antioxidant mechanisms in the organism and their products. Glutathione (GSH) and non-protein thiols (NP-SH) absorb ROS and are consumed in response to oxidative damage; therefore, their levels decreased with increased oxidative stress. On the other hand, the levels of their reactive by-products, glutathione-mixed disulfides (G-SS-NP) and non-protein disulfides (NP-SS-NP), increased during oxidative damage. The names of the markers are based on a study by Chen et al. [2008] (20) (see Table 2). Oxidative damage markers were measured both in serum and in liver tissue.
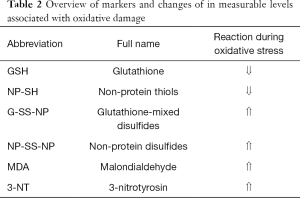
Full table
Hepatic homogenate
Liver homogenates were prepared by adding a 10 mmol of saline phosphate buffer solution (PBS tablet, Sigma-Aldrich, Saint Louis, USA), pH 7.4, in a ratio of 7 mL PBS to 1 g of liver tissue. The liver tissue in the PBS solution was first processed using a T 25 digital ULTRA-TURRAX dispersive device (IKA-Werke GmbH & Co. KG, Staufen, Germany) for 30 seconds and then ultrasonic homogenization using a UP50H Compact Lab Homogenizer (Hielscher Ultrasonics, Teltow, Germany) for 5 minutes. The homogenate formed was centrifuged using a Hettich UNIVERSAL 320/320 R centrifuge (Hielscher Ultrasonics, Teltow, Germany) at 10,000× g at 4 °C for 30 minutes and the resulting supernatant was processed using chromatographic analysis.
MDA and 3-NT were assessed using liquid chromatography-tandem mass spectrometry (LC-MS/MS), with 25 µL of serum/supernatant diluted with water (AquaOsmotic, Tišnov, Czech Republic) to 250 µL. Glutathione (GSH) NP-SH, glutathione-mixed (G-SS-NP), and non-protein disulfides (NP-SS-NP) were determined from 100 µL of serum/supernatant using HPLC-UV/VIS. The samples were further modified and analyzed under conditions described by Vanova et al. [2018] (21).
Statistical analysis
Data obtained from all measurements are expressed as medians (quartile Q0.25, quartile Q0.75) or mean ± standard deviation in international units. The data were evaluated using one-way or two-way variance analysis (ANOVA) using SigmaStat software (Systat Software, San Jose, CA, USA), and the level of statistical significance was set at P<0.05. Histological samples were evaluated semi-quantitatively using Suzuki scores, and all were assessed by the same pathologist.
Results
A total of 12 laboratory animals (domestic pigs) were included in this study, and the animals were randomly divided into two groups of 6 animals (intervention group and control group). The average weight of animals was 32.5±2.2 kg. The average surgery time from the cutaneous incision up to the tourniquet placed on the left branch of the portal vein and the left hepatic artery was 30±7.2 minutes.
Histological examination
The evaluation of histological changes caused by ischemia-reperfusion damage was based on the degree of sinusoidal congestion, vacuolation (balloon degeneration), and hepatocyte necrosis, followed by numerical expression of the severity of the damage according to the Suzuki score. A two-way analysis of variance (ANOVA) was calculated with the following factors, “intervention group” (n=6) vs. “control group” (n=6) and “left (ischemic) lobe” (n=6) vs. “right (non-ischemic) lobe” (n=6). The average Suzuki score for left lobes was 1.000±0.274 (left × hydrogen) in the intervention group, compared to 1.667±0.274 in the control group (left × no hydrogen), which corresponds to a difference in average values of 66.7%. In right (non-ischemic) lobes, the mean Suzuki score was 0.167±0.274 (right × hydrogen) in the intervention group versus 0.833±0.274 in the control group (right × no hydrogen), which corresponds to an average difference of 398% (see Figure 1).
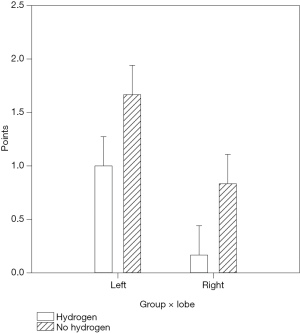
Therefore, liver samples in the intervention group using hydrogen showed statistically significantly fewer histological changes compared to the control group (hydrogen × no hydrogen, P=0.024). These differences are demonstrable both in the non-ischemic lobes and the ischemic lobes (left × right, P=0.006). Moreover, in the non-ischemic lobes of the intervention group, histological changes following prolonged ischemia and reperfusion were almost negligible (see Figure 2).
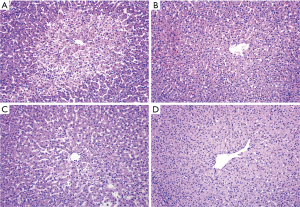
Biochemical examination
The measured values of the biochemical tests showed no statistically important difference in hepatic transaminases (AST, ALT), alkaline phosphatase (ALP), lactate dehydrogenase (LD), and lactate (see Table 3). Creatine kinase values (including subtype BB) seemed completely random and statistically non-significant, as such, we failed to confirm the suggestion by Vaubourdolle et al. [1993], and our conclusion is that creatine kinase BB cannot be used as a simple indicator of the extent of ischemia-reperfusion liver damage.
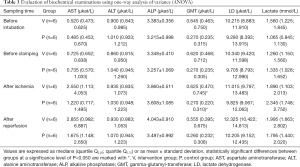
Full table
However, a surprising result was a statistically significant difference in gamma-glutamyl-transferase (GMT) between the intervention group and the control group (P<0.001). Its level fluctuated minimally over the course of the surgery; however, in the intervention group, the measured value was on average 2.5× higher than in the control group (see Figure 3).
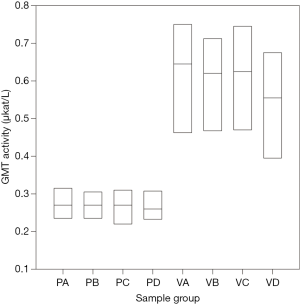
Oxidative damage markers
The levels of oxidative damage markers varied significantly, especially in plasma samples (see Table 4). The level of MDA and 3-NT was considerably lower in the intervention group using hydrogen than in the control group, indicating a lower degree of oxidative stress. Accordingly, a considerably higher level of GSH and NP-SH and lower levels of their oxidation products (non-protein disulfides; NP-SS-NP) were demonstrated in the intervention group. Significant differences were observed in hepatic tissue when comparing the non-ischemic (right) and ischemic (left) lobes of the liver (see Table 5). The difference in glutathione-mixed disulfides levels (G-SS-NP) was non-significant both in plasma and in hepatic tissue.
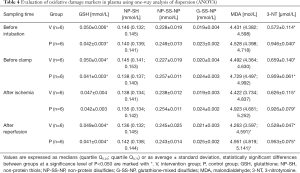
Full table
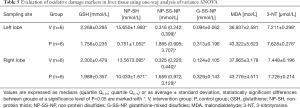
Full table
Discussion
Liver resection is a high-risk surgery that is often associated with severe complications, with liver failure in the postoperative period being one of the most severe and can easily be fatal. One of the key mechanisms involved in the development of liver dysfunction is ischemia-reperfusion damage. In this experimental study, we evaluated the antioxidant, anti-inflammatory, and antiapoptotic effects of inhaled hydrogen, on domestic pigs, in a clinically relevant model of ischemia-reperfusion liver damage. The effects were assessed using histological and biochemical examinations, including an examination of oxidative damage markers.
Inhaled hydrogen appears to be the most suitable form of administration due to its rapid tissue saturation and the ease of maintaining constant levels during surgery. Other possible administrations methods include the intravenous application of hydrogen-enriched physiological saline solution and consumption of drinking water enriched with hydrogen.
In 2001, the first study on the protective effect of hydrogen, under high pressure, on liver damage was published. In 2007, the therapeutic influence of hydrogen on liver ischemia was further explored (7). Studies dealing with the effect of hydrogen on hepatic tissue are relatively new, and the research methods and types of liver diseases vary widely.
In one of the first studies, Gharib et al. (22) examined the influence of molecular hydrogen on a mouse model with parasitic chronic liver disease (schistosomiasis infestation). Mice breathed a mixture of hydrogen and oxygen in a hyperbaric chamber for 14 days (87.5% hydrogen at 0.7 MPa). The authors observed liver function, oxidative damage to liver tissue, fibrosis, and signs of inflammation and concluded that continuous administration of high doses of hydrogen had significant protective effects relative to liver functions. This study was innovative since it demonstrated the anti-inflammatory and antioxidant effects of hydrogen. Because the hydrogen doses were so high, the study had limited transferability in terms of clinical use. In 2007, Ohsawa et al. (7) published another important work, showing that the same antioxidant effect can be achieved by inhaling a 2% hydrogen mixture for 35 minutes. These findings had much greater clinical potential. Then Fukuda et al. (1) published a study on the effects of hydrogen on ischemia-reperfusion liver damage using a rat model. The authors found, based on histological sections and the level of MDA and liver tests, that hydrogen had a significant effect on ischemic liver damage. In 2009, Kajiya et al. demonstrated the protective effect of hydrogen on concanavalin A-induced hepatitis (23). In the same year, Tsai et al. found that hydrogen-enriched water protects liver tissue in mice with carbon tetrachloride-induced hepatitis (24). Sun et al. demonstrated that hydrogen not only protects against liver damage, it also helped prevent three different models of liver cirrhosis, i.e., GalN/LPS, CCl4, and DEN (25). Liu et al. found that intraperitoneal application of hydrogen-enriched fluid could improve the antioxidant capacity of the liver and reduce the inflammatory response, which is of great clinical significance (26). Current studies show that long-term consumption of hydrogen-enriched water can improve the symptoms of non-alcoholic steatohepatitis by improving both liver function and liver morphology (in terms of preventing fibrosis) (27). In this same study, the authors demonstrate not only the long-term effects of hydrogen-enriched water to improve liver function, but also to reduce the risk of developing malignancies. This is strong evidence that hydrogen may also have application in the prevention of carcinogenesis. Kang et al. showed that daily use of hydrogen-enriched water improves the quality of life in patients who were irradiated as part of liver tumor treatment. The effects can be explained by a reduction in the oxidative stress and inflammation caused by radiotherapy (28). Another study showed the positive effect of hydrogen in obese diabetics in preventing the development of hepatic steatofibrosis and reducing insulin resistance (29). In 2013, Xia et al. published the result of a study in which 30 patients with chronic hepatitis B used hydrogen-enriched water for 6 weeks. These patients were found to have improved liver function and reduced levels of circulating HBV DNA compared to the control group of similar patients (30). In 2015 Zhang et al. published the results of a study examining rats who underwent a syngeneic orthotopic liver transplant. Before the transplant, they inhaled a mixture of 1–3% hydrogen. Rats who inhaled a 2% mixture of hydrogen showed considerably lower ischemia-reperfusion liver damage (31). In 2017 Lin et al. published a study confirming the antioxidant effects of hydrogen-enriched water in mice with ethanol-induced oxidative stress. The mice showed improved liver function, lower MDA levels, and increased function of intrinsic natural scavenger mechanisms (32). Recently, Uto et al. used UW solution enriched with dissolved hydrogen to store livers prior to syngeneic orthotopic liver transplant in rats. The liver transplants showed lower oxidative damage and a lower number of apoptotic hepatocytes compared to the control group (33). Lastly, Ge et al. showed the protective effect of hydrogen during laparoscopic hepatectomy in mini pigs. Post-operatively, the animals showed lower levels of inflammatory markers (interleukin IL-1, IL-6, TNF-α and CRP) and cortisol (34).
The selected studies mentioned show that hydrogen has significant potential to be an antioxidant in liver ischemia, hepatitis, steatosis, and other liver diseases.
Due to the complete anatomical separation of the vascular supply to the lobes of the liver, a tourniquet on the vena portae branch and the relevant hepatic artery will cause complete ischemia of the left or right liver lobe (in the case of domestic pigs, the two left and two right lobes). This form of hepatic ischemia is macroscopically noticeable with a near-instantaneous demarcation of ischemic and non-ischemic liver tissue. The subsequent transection only breaks the parenchyma and small vascular conjunctions and bile ducts, but does not further increase ischemia, and thus does not enhance oxidative stress. Preparation and the application of a hepatoduodenal ligament clamp in the area of the porta hepatis is, therefore, a key step in large liver resections (e.g., hemi-hepatectomy). The length of the clamp can also influence the severity of ischemia-reperfusion liver damage. In our study, we, therefore, limited the preparation and clamp of the relevant vascular branches without subsequent transection and removal of liver parenchyma. Completion of a hemi-hepatectomy would not provide additional meaningful observations regarding the effects of hydrogen during reperfusion. Due to the advantageous placement of the left branch of the vena portae in domestic pigs, we chose the left liver lobe for ischemia induction and subsequent reperfusion.
The length of ischemia and reperfusion was deliberately much longer than in normal liver resections in human medicine. As mentioned in previous studies by other authors (18,19,35) in our previous experiments, we had found that a shorter period of ischemia and reperfusion produced only minimal changes in the liver parenchyma of young, healthy animals, and therefore it would not be possible to assess the protective effect of inhaled hydrogen with shorter times.
The evaluation of histological changes due to ischemic-reperfusion damage was semi-quantitatively expressed using Suzuki scores. Liver samples from the intervention group (i.e., with inhaled hydrogen) showed statistically significantly fewer histological changes compared to the control group. In addition, the histological changes were all but negligible in the non-ischemic lobes in the intervention group. The results show that the use of hydrogen statistically significantly reduces the degree of ischemia-reperfusion liver damage at the histological level.
Biochemical test values showed no statistically important difference in hepatic transaminases (AST, ALT), ALP, LD, and lactates. Although peri-operative levels of AST increased, the increase was likely attributable to muscle tissue damage that occurred during the laparotomy. Moreover, the difference between the two groups was completely non-significant. Creatine kinase values (including subtype BB) seemed completely random and statistically non-significant; therefore, we failed to confirm the conclusion of Vaubourdolle et al. [1993] (17), and from our results, creatine kinase BB cannot be used as a simple indicator of the extent of ischemia-reperfusion liver injury. Likewise, we failed to show that routine liver function tests can serve as indicators of the severity of ischemia-reperfusion liver damage. However, a surprising result was a statistically significant difference in gamma-glutamyl transferase (GMT) levels. While levels fluctuated only minimally during the operation, the intervention group had values that were, on average, 2.5 times higher than in the control group. The elevated GMT value, in combination with elevated ALP, is generally considered to be a marker of hepatobiliary tract injury. However, all measured values in our study were within the physiological range; therefore, the different levels of GMT can probably be attributed to oxidative damage to the GMT molecule at higher levels of oxidative stress, which leads to a decrease in the level of GMT in the blood. GMT levels could thus serve as a predictive factor of the severity of ischemia-reperfusion damage in the early postoperative period. This assumption is in accordance with a study by Belcastro et al. [2017] (36), in which the authors observed the phenomenon of decreased levels of GSH, NP-SH (see Oxidative damage markers), and 3.5-fold decrease in GMT activity with increased oxidative stress, just as we observed in our study.
The markers of oxidative damage varied significantly in plasma samples. The level of MDA and 3-NT was significantly lower in the intervention group (with inhaled hydrogen) than in the control group, which indicates a lower degree of oxidative stress. Accordingly, the intervention group demonstrated substantially higher levels of GSH and NP-SH and lower levels of their oxidation products (non-protein disulfides; NP-SS-NP), which is in accordance with the assumption set out in the methodology. Significant differences were observed in hepatic tissues both in comparison with the non-ischemic (right) and ischemic (left) lobes of the liver. Differences in glutathione-mixed disulfides levels (G-SS-NP) was non-significant both in plasma and in hepatic tissues.
Although we failed to see a considerable difference in the tested oxidative damage markers in the plasma and liver tissue, perhaps due to the large dispersion of the measured values, the observed trends, both decreasing and increasing were in line with our expectations for almost in all selected markers. Our results strongly suggest that the administration of hydrogen during surgery greatly reduces the level of oxidative stress in the organism, and results in less severe ischemia-reperfusion damage.
We demonstrated the positive antioxidant effect of inhaled 2% molecular hydrogen on domestic pigs during large liver resection, using histological and biochemical examinations and examination of oxidative damage markers. We showed that this method is safe without any known side effects and easy to use and can be easily translated to human medicine. In liver surgery, it may be a safe approach to improve results of major liver resections and liver transplantation. Although “large hepatic resection” is not strictly defined, it usually means a left or right hepatectomy. More specifically, resection of four or more liver segments can be defined as large liver resection. We assume that this positive protective effect of inhaled molecular hydrogen during surgery will reduce the risk and incidence of acute liver failure in the postoperative period. It could also be suitable for urgent treatment of organ ischemia (e.g., acute coronary syndrome or mesenteric ischemia). Further research is necessary to show whether GMT levels can actually serve as a predictive factor for the severity of ischemia-reperfusion damage.
Conclusions
In this experimental study, we demonstrated that hydrogen inhalation during major resection of the liver considerably reduced the level of oxidative stress associated with ischemia-reperfusion liver damage and thus protected the hepatic parenchyma against its negative effects. We confirmed this phenomenon both with histological sections and by direct measurement of the level of oxidative stress in the organism. Furthermore, we suspect that the level of GMT could serve as a predictive factor of ischemia-reperfusion damage in the early postoperative period, and thus could predict the risk of hepatic failure in patients after resections and liver transplantation.
Acknowledgments
We thank Prof. Thomas Secrest, MSc. (Secrest Editing s.r.o.) for language editing and proofreading the article. Financial support for this study was provided by the grant of the Ministry of Defence of the Czech Republic called “Long-term organization development plan – Medical Aspects of Weapons of Mass Destruction”.
Footnote
Conflicts of Interest: The authors have no conflicts of interest to declare.
Ethical Statement: The authors are accountable for all aspects of the work in ensuring that questions related to the accuracy or integrity of any part of the work are appropriately investigated and resolved. All animals received humane care according to the criteria outlined in the Guide for the Care and Use of Laboratory Animals prepared by the National Academy of Sciences and published by the National Institutes of Health (NIH publication 86-23 revised 1985).
References
- Fukuda K, Asoh S, Ishikawa M, et al. Inhalation of hydrogen gas suppresses hepatic injury caused by ischemia/reperfusion through reducing oxidative stress. Biochem Biophys Res Commun 2007;361:670-4. [Crossref] [PubMed]
- Montalvo-Jave EE, Escalante-Tattersfield T, Ortega-Salgado JA, et al. Factors in the Pathophysiology of the Liver Ischemia-Reperfusion Injury. J Surg Res 2008;147:153-9. [Crossref] [PubMed]
- Shuh M, Bohorquez H, Loss GE, et al. Tumor Necrosis Factor-α: Life and Death of Hepatocytes During Liver Ischemia/Reperfusion Injury. Ochsner J 2013;13:119-30. [PubMed]
- Perry BC, Soltys D, Toledo AH, et al. Tumor Necrosis Factor-α in Liver Ischemia/Reperfusion Injury. J Invest Surg 2011;24:178-88. [Crossref] [PubMed]
- Ildefonso JÁ, Arias-Díaz J. Pathophysiology of liver ischemia—Reperfusion injury. Cirugía Española 2010;87:202-9. (English Ed). [Crossref] [PubMed]
- Cursio R, Colosetti P, Gugenheim J. Autophagy and liver ischemia-reperfusion injury. Biomed Res Int 2015;2015:417590. [Crossref] [PubMed]
- Ohsawa I, Ishikawa M, Takahashi K, et al. Hydrogen acts as a therapeutic antioxidant by selectively reducing cytotoxic oxygen radicals. Nat Med 2007;13:688-94. [Crossref] [PubMed]
- Huang CS, Kawamura T, Toyoda Y, et al. Recent advances in hydrogen research as a therapeutic medical gas. Free Radic Res 2010;44:971-82. [Crossref] [PubMed]
- Ohta S. Molecular hydrogen is a novel antioxidant to efficiently reduce oxidative stress with potential for the improvement of mitochondrial diseases. Biochim Biophys Acta 2012;1820:586-94. [Crossref] [PubMed]
- Ohta S. Molecular hydrogen as a preventive and therapeutic medical gas: initiation, development and potential of hydrogen medicine. Pharmacol Ther 2014;144:1-11. [Crossref] [PubMed]
- Iketani M, Ohsawa I. Molecular Hydrogen as a Neuroprotective Agent. Curr Neuropharmacol 2017;15:324-31. [Crossref] [PubMed]
- Huang L. Molecular hydrogen: a therapeutic antioxidant and beyond. Med Gas Res 2016;6:219. [Crossref] [PubMed]
- Ichihara M, Sobue S, Ito M, et al. Beneficial biological effects and the underlying mechanisms of molecular hydrogen - Comprehensive review of 321 original articles. Med Gas Res 2015;5:12. [Crossref] [PubMed]
- Shen M, Zhang H, Yu C, et al. A review of experimental studies of hydrogen as a new therapeutic agent in emergency and critical care medicine. Med Gas Res 2014;4:17. [Crossref] [PubMed]
- Sun X, Ohta S, Nakao A. Hydrogen Molecular Biology and Medicine. 1st edition. Dordrecht: Springer, 2015:117.
- Ono H, Nishijima Y, Adachi N, et al. A basic study on molecular hydrogen (H2) inhalation in acute cerebral ischemia patients for safety check with physiological parameters and measurement of blood H2 level. Med Gas Res 2012;2:21. [Crossref] [PubMed]
- Vaubourdolle M, Chazouilleres O, Poupon R, et al. Creatine kinase-BB: A marker of liver sinusoidal damage in ischemia-reperfusion. Hepatology 1993;17:423-8. [Crossref] [PubMed]
- Datta G, Fuller BJ, Davidson BR. Molecular mechanisms of liver ischemia reperfusion injury: Insights from transgenic knockout models. World J Gastroenterol 2013;19:1683-98. [Crossref] [PubMed]
- Arab HA, Sasani F, Rafiee MH, et al. Histological and biochemical alterations in early-stage lobar ischemia-reperfusion in rat liver. World J Gastroenterol 2009;15:1951-7. [Crossref] [PubMed]
- Chen W, Zhao Y, Seefeldt T, et al. Determination of thiols and disulfides via HPLC quantification of 5-thio-2-nitrobenzoic acid. J Pharm Biomed Anal 2008;48:1375-80. [Crossref] [PubMed]
- Vanova N, Muckova L, Schmidt M, et al. Simultaneous determination of malondialdehyde and 3-nitrotyrosine in cultured human hepatoma cells by liquid chromatography-mass spectrometry. Biomed Chromatogr 2018;32:e4349. [Crossref] [PubMed]
- Gharib B, Hanna S, Abdallahi OM, et al. Anti-inflammatory properties of molecular hydrogen: investigation on parasite-induced liver inflammation. C R Acad Sci III 2001;324:719-24. [Crossref] [PubMed]
- Kajiya M, Sato K, Silva MJB, et al. Hydrogen from intestinal bacteria is protective for Concanavalin A-induced hepatitis. Biochem Biophys Res Commun 2009;386:316-21. [Crossref] [PubMed]
- Tsai CF, Hsu YW, Chen WK, et al. Hepatoprotective effect of electrolyzed reduced water against carbon tetrachloride-induced liver damage in mice. Food Chem Toxicol 2009;47:2031-6. [Crossref] [PubMed]
- Sun H, Chen L, Zhou W, et al. The protective role of hydrogen-rich saline in experimental liver injury in mice. J Hepatol 2011;54:471-80. [Crossref] [PubMed]
- Liu Q, Shen WF, Sun HY, et al. Hydrogen-rich saline protects against liver injury in rats with obstructive jaundice. Liver Int 2010;30:958-68. [Crossref] [PubMed]
- Kawai D, Takaki A, Nakatsuka A, et al. Hydrogen-rich water prevents progression of nonalcoholic steatohepatitis and accompanying hepatocarcinogenesis in mice. Hepatology 2012;56:912-21. [Crossref] [PubMed]
- Kang KM, Kang YN, Choi IB, et al. Effects of drinking hydrogen-rich water on the quality of life of patients treated with radiotherapy for liver tumors. Med Gas Res 2011;1:11. [Crossref] [PubMed]
- Kamimura N, Nishimaki K, Ohsawa I, et al. Molecular Hydrogen Improves Obesity and Diabetes by Inducing Hepatic FGF21 and Stimulating Energy Metabolism in db/db Mice. Obesity 2011;19:1396-403. [Crossref] [PubMed]
- Xia C, Liu W, Zeng D, et al. Effect of hydrogen-rich water on oxidative stress, liver function, and viral load in patients with chronic hepatitis B. Clin Transl Sci 2013;6:372-5. [Crossref] [PubMed]
- Zhang CB, Tang YC, Xu XJ, et al. Hydrogen gas inhalation protects against liver ischemia/reperfusion injury by activating the NF-κB signaling pathway. Exp Ther Med 2015;9:2114-20. [Crossref] [PubMed]
- Lin CP, Chuang WC, Lu FJ, et al. Anti-oxidant and anti-inflammatory effects of hydrogen-rich water alleviate ethanol-induced fatty liver in mice. World J Gastroenterol 2017;23:4920-34. [Crossref] [PubMed]
- Uto K, Sakamoto S, Que W, et al. Hydrogen-rich solution attenuates cold ischemia-reperfusion injury in rat liver transplantation. BMC Gastroenterol 2019;19:25. [Crossref] [PubMed]
- Ge YS, Zhang QZ, Li H, et al. Hydrogen-rich saline protects against hepatic injury induced by ischemia-reperfusion and laparoscopic hepatectomy in swine. Hepatobiliary Pancreat Dis Int 2019;18:48-61. [Crossref] [PubMed]
- Xiang L, Tan JW, Huang LJ, et al. Inhalation of hydrogen gas reduces liver injury during major hepatotectomy in swine. World J Gastroenterol 2012;18:5197-204. [PubMed]
- Belcastro E, Wu W, Fries-Raeth I, et al. Oxidative stress enhances and modulates protein S-nitrosation in smooth muscle cells exposed to S-nitrosoglutathione. Nitric Oxide 2017;69:10-21. [Crossref] [PubMed]