In vivo gait kinematics of the knee after anatomical and non-anatomical single-bundle anterior cruciate ligament reconstruction—a prospective study
Introduction
Anterior cruciate ligament (ACL) maintains knee stability not only by controlling anterior translation of the tibia but also restricting knee rotation in the axial and transverse plane (1). The increased anterior translation of tibia and knee rotational imbalance accompanies ACL injuries (2). Moreover, ACL injuries alter the kinematics of knees with or without meniscal tears during level walking test (3). After ACL tears, the knee joint remains unstable and more prone to further injuries like the damage to menisci and articular cartilage which may lead to premature arthritis (4,5). Many studies have reported that anatomical reconstruction (AR) can restore superior rotational stability and clinical outcomes than non-anatomical reconstruction (non-AR) (6-11). However, few studies have been reported on the reasons for this discrepancy.
Gait kinematics, the most common daily activity, are closely related to knee functions and stability. The abnormal knee kinematics often accompanies ACL injuries (12-14). Zhang et al. (3) showed that the ACL deficient knees, with or without meniscal tears, exhibited significant less flexion and more femoral external rotation than ACL intact knees. The abnormal gait kinematics was found to be a risk factor for lower limb tendinopathy (15). Moreover, abnormal kinematics may be one of the possible reasons for subsequent joint degenerations after anterior cruciate ligament reconstruction (ACLR) (16,17). Imhauser et al. (16) performed a cadaveric study to simulate clinical tests of anterior and rotational stability after ACLR. They concluded that abnormal contact stress was associated with altered kinematics after ACLR, which may affect the progression of osteoarthritis. Andriacchi and Favre (17) summarized the in vivo mechanical signals influenced cartilage health and progression to knee osteoarthritis. So, kinematics analysis should be an important index to evaluate functional restoration of knees after ACLR.
Graft orientation caused by the placement of tunnel aperture has been reported to affect the knee functions and clinical outcomes (18-22). Musahl et al. (19) performed a cadaveric study with the cadaveric knees tested in response to a 134-N anterior load and a combined 10-N.m valgus and 5-N.m internal rotation load. They concluded a femoral tunnel position inside the anatomical footprint resulted in knee kinematics closer to the intact knee. Zantop et al. (22) also summarized anatomical tunnel placement of anteromedial (AM) and posterolateral bundles can restore intact knee kinematics based on a cadaveric study with the knees in response to a 134-N anterior tibial load and a combined rotatory load of 10 N.m valgus and 4 N.m internal tibial rotation using a robotic/universal force moment sensor testing system. Howell et al. (18,21) measured the MR signal intensity of unimpinged and impinged ACL grafts. They found impinged ACL grafts showed an increase in signal intensity in the distal two-thirds of the graft. While the unimpinged grafts with more vertical graft orientation on the coronal plane remained low signal intensity. Brophy et al. (20) reported that more vertical graft orientation may be a reason for ACL revision. These studies demonstrated that the ACL graft orientation may be a determinant for the function restoration after ACLR. However, the effect of graft orientations on in vivo gait kinematics has rarely been reported.
To the best of our knowledge, few studies have been performed to compare gait kinematics between ACLR knees and contralateral normal knees after anatomical and non-anatomical single-bundle ACLR, separately. The purpose of this study was to evaluate the gait kinematics and clinical outcomes of knees after anatomical and non-anatomical single-bundle ACLR during level walking. A portable optical tracking system was used to measure the 6 degrees of freedom (DOF) kinematics of the single-bundle ACLR knees, the ACL-intact (ACLI) contralateral normal knees and the knees of normal people during treadmill gait. This study was expected to provide references for the placement of tunnel aperture and graft orientation during ACLR.
Methods
Study design
After approval from the Institutional Review Board of Nanjing Drum Tower Hospital (2019-024-01) and obtaining informed consent, 34 patients (28 males, 6 females; age range, 18–46 years; BMI range, 19.3–37.7 kg/m2) were recruited. The inclusion criteria included unilateral primary single-bundle ACLR, closed epiphyseal plate and healthy volunteers. The exclusion criteria included multi-ligament injuries, lower extremity or spine deformities, positive anterior draw test or Lachman test. Subgroups were determined according to the femoral and tibial tunnel placement, including anatomical reconstruction group (AR group) and non-anatomical reconstruction group (Non-AR group). The International Knee Documentation Committee (IKDC) evaluation form and Lysholm score were used to assess the subjective knee function restoration. The objective laxity test was performed by using the KT-2000 arthrometer (MEDmetric Corp, San Diego, CA, USA) and the side to side difference in displacement was recorded under manual maximum laxity test (23). In order to compare gait kinematics between left and right knees of healthy people, 18 healthy people were also recruited. A portable optical tracking system (3,13) was used to measure the 6 DOF kinematics of the single-bundle ACLR knees, the ACLI contralateral normal knees and the knees of healthy people during treadmill gait (Figure 1). The range of motion (ROM) of each DOF was also calculated. Then, the comparison of 6 DOF kinematics and ROM of 6 DOF kinematics were performed between ACLR knees and ACLI contralateral normal knees. The comparison of 6 DOF kinematics between left and right knees of healthy people was also performed.
Surgical procedure and postoperative rehabilitation
The surgical procedures were completed by Dr. DYC, Dr. ZHX and Dr. XQX. The arthroscopic exploration and debridement were performed through the AM and anterolateral (AL) portal. Simultaneously, meniscal tear was diagnosed and treated either by partial resection or suture. The autologous peroneus longus tendon was harvested and knitted to serve as the ACL graft. The femoral tunnel aperture and tibial tunnel aperture were created separately. The tibial tunnel aperture was drilled with use of tibial tunnel guide, based on the ACL anatomical tibial footprint. Then the femoral tunnel aperture was drilled with the AM portal technique. The interference screw was used for the femoral side and tibial side autograft fixation. Finally, the wounds would be closed if the knee stability and graft tension met the surgeon’s satisfaction.
In order to alleviating knee swelling and pain, the ice compress was applied after surgery, immediately. The knee was immobilized in full extension with a brace in the early phase. Within 4 weeks, patients were encouraged to perform ankle pump exercise, isometric quadriceps and hamstring contractions, straight and side leg raising exercises. The non-weight bearing knee flexion exercise was performed to improve the ROM from the second week. The gradual-increasing weight-bearing exercise was allowed by at least 4 weeks and full weight-bearing exercise was permitted from 6 weeks. Running and swimming was permitted until 3 months, but contact sports were not suggested until 12 months after operation.
Tunnel placement determination and distribution
All patients were scanned by a CT scanner (GE Discovery CT 750 HD, GE Medical Systems) in the supine position with knees extended and thighs horizontal and parallel. Axial images (120 kV, tube voltage; 185 mAs/slice, tube current; 0.426, pitch factor; 512×512, matrix; 0.625 mm, reconstruction thickness) of the knee were obtained. The 3D model of distal femur and proximal tibia were reconstructed by RadiAnt DICOM ViewerTM (Medixant, Poznán, Poland). For femoral tunnel placement, on the distal view, the medial femoral condyle was cut off along the highest point of intercondylar notch, then the model was rotated to show the medial side of lateral condyle. The Bernard quadrant method (24) was used to measure the femoral tunnel position (Figure 2A). For tibial tunnel placement, the method described by Amis et al. (25) (Figure 2B) was used. The distribution of the centers of femoral tunnel aperture was displayed in Figure 2C. Xu et al. (26) described the standard area of anatomical ACL femoral footprint center as a circle (x, y; 27.53%±4.58%, 35.85%±9.2%). In this study, the centers of femoral tunnel aperture within the standard area were defined as anatomical reconstruction group (AR group, n=13), while the centers outside the standard area were defined as Non-anatomical reconstruction group (Non-AR group, n=21). The position of the centers of tibial tunnel aperture was 35.0%±4.6% (95% CI: 33.3–36.6%). In this study, the centers of all included tibial tunnel aperture were within the anatomical ACL tibial footprint (Figure 2D).
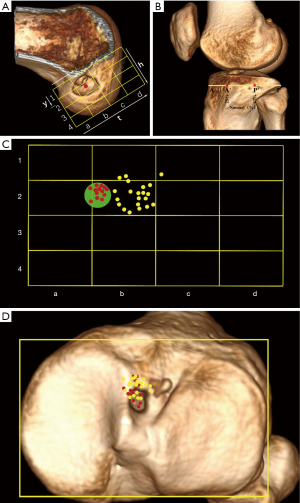
Graft orientation measurement
For ACL graft orientation measurement, the 3D model of distal femur and proximal tibia were reconstructed by the Mimics 17.0 software (Materialise N.V., Heverlee, Belgium). The geometric center of femoral and tibia tunnel aperture was determined. Then, a cylinder connecting the femoral tunnel center and the tibial tunnel center was constructed to simulate the ACL graft. The long axis of cylinder was used to measure the graft orientation relative to the tibial plateau on coronal and sagittal plane as described by Scanlan et al. (27) (Figure 3).
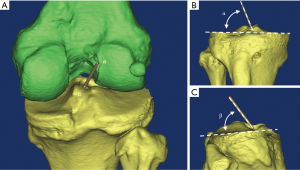
Gait kinematics acquisition
The gait kinematics was acquired by an optical tracking system (Opti-Knee, Innomotion Inc., Shanghai, China) while walking on a treadmill at the speed of 3.0 km/h (Figure 4). The lower limb anatomic bone markers and infrared-light reflecting rigid bodies were prepared following a previously published protocol (3). Two rigid bodies, with each body comprising four infrared light-reflecting markers (OK_Marquer; Innomotion Inc), were separately fixed in the thigh and shank with bandages. The femoral and tibial anatomical landmarks (i.e., greater trochanter, lateral epicondyle, medial epicondyle, lateral tibia plateau, medial tibia plateau, medial malleolus, lateral malleolus) were identified by a handled digitizing probe with four infrared light-reflecting markers. After a 5-min treadmill gait warm-up, fifteen seconds of kinematics data were obtained by an integrated two-head stereo-infrared camera at 60 Hz, while an integrated synchronous high-speed camera was used to capture the walking video for further gait cycle segmentation. Raw kinematics data was smoothed by a low-pass filter at a frequency of 6 Hz. The rotational and translational parameters (6 DOF) of knee kinematics were calculated based on the coordinate system of the tibia relative to the femur (Figure 5). The translation parameter was defined as the displacement of the origin of tibial coordinate system relative to the femoral coordinate system, including anterior (+)/posterior translation, proximal (+)/distal translation and medial/lateral (+) translation. Similarly, the rotational parameter was defined as the tibial coordinate system relative to the femoral coordinate system along the anterior-posterior, medial-lateral and proximal-distal axis in the Euler angle sequence, including varus/valgus (+), internal/external (+) rotation, flexion (+)/extension. The ensemble average curve of each DOF was generated by using GraphPad Prism 8 (GraphPad Software, Inc., USA). Gait cycle segmentation was defined by a kinematic approach (28) mainly including a stance phase and a swing phase.
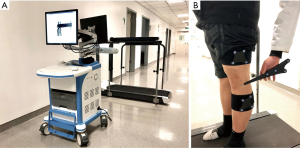
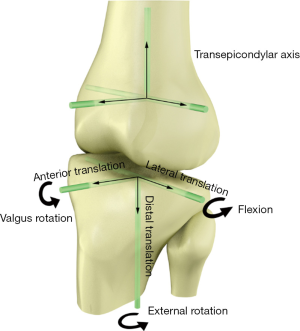
Statistical analysis
Descriptive statistics was used to summarize the data. All data were described as Means and standard deviations, excepting for the manual maximum laxity, IKDC and Lysholm scores using Means and 95% CI. Before statistical analysis, all data were tested for normality distribution and homogeneity of variance. Normality distribution was checked with Shapiro-Wilk test, and the homogeneity of variance was tested by Levene statistic. Then the unpaired t-test, Welch’s t-test and Mann-Whitney U test were applied according to the result of normality distribution and homogeneity of variance. Statistical analysis was performed with IBM SPSS Statistics 16 (IBM Corporation, NY, USA). Baseline characteristics of two groups were tested with Chi-square (χ2) test. A value of P<0.05 was considered to be statistically significant for all tests.
The statistical parametric mapping (1d nonparametric unpaired t-test) was used to examine the difference of kinematics between ACLR knees and contralateral normal knees and the left and right knees of normal populations were compared with the same method. SPM1D package available for Matlab (v.0.4,
Results
Demographics, surgical findings and clinical evaluations of two groups
In total, 34 patients (AR group, n=13; Non-AR group, n=21) and 18 healthy people were recruited. The two groups were comparable in terms of sex, age, height, weight, BMI and follow-up time (Table 1). Moreover, the initial status of knee injuries and treatment to meniscal injuries were also comparable between the two groups (Table 1). No statistically significant differences were observed between two groups in terms of post-operative IKDC subjective score, Lysholm score (Figure 6A) and manual maximum laxity test by KT-2000 (Figure 6B) at follow up time.
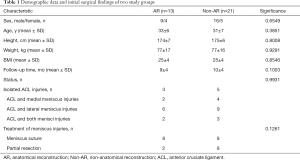
Full table
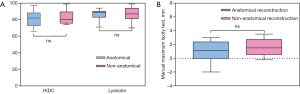
Graft orientation
For AR group, the mean graft angle on coronal plane was 71.4°±7.2°, and the mean graft angle on sagittal plane was 60.4°±7.5°. For Non-AR group, the mean graft angle on coronal plane was 75.6°±8.0°, and the mean graft angle on sagittal plane was 56.8°±7.5° (Table 2).
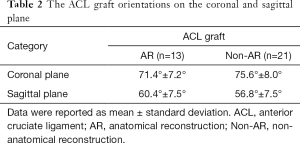
Full table
Gait kinematics and ROM
During the entire gait cycle, no statistically significant differences were observed in kinematics between left and right knees of healthy people (Figure 7), confirmed by SPM1D analysis. Moreover, neither the AR group (Figure 8) nor the Non-AR group (Figure 9) exhibited statistically significant differences in kinematics between the ACLR knees and the corresponding contralateral normal knees. For AR group, there were no statistically significant differences between the contralateral normal knees and ACLR knees in terms of ROM of 6 DOF (Table 3). For Non-AR group, the ROM of anterior-posterior translation of the ACLR knees was significantly larger than that of the corresponding contralateral normal knees (1.8±0.6 vs. 1.3±0.5 cm; P=0.0080). No statistically significant differences were observed in ROM of the other 5 DOF between the ACLR knees and the corresponding contralateral normal knees (Table 4).
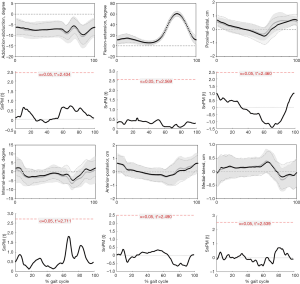
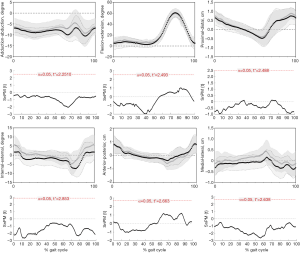
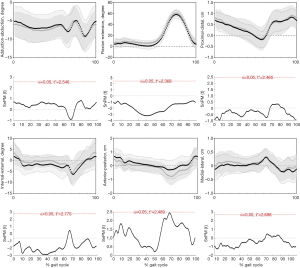
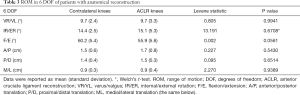
Full table
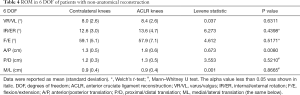
Full table
Discussion
In our study, there were no statistically significant differences between left and right knees of healthy people in terms of gait kinematics. So, the gait kinematics between ACLR knees and contralateral normal knees of patients were comparable. Our results showed that the AR group exhibited no statistically significant differences in terms of kinematics and ROM of 6 DOF between the ACLR knees and contralateral normal knees during level walking. For Non-AR group, the ACLR knees exhibited significant ROM of anterior-posterior translation by approximately 0.5 cm than contralateral knees.
The results observed in our study were consistent with some other reports (30-33), exhibiting improved locomotion and function restoration after ACLR. Papageorgiou et al. (30) examined kinematics of ten cadaveric knees using a robotic/universal force-moment sensor testing system. They found that the varus rotation and internal tibial rotation were restored compared with intact knees after ACLR under a combined 134-N anterior and 200-N axial compressive tibial load. Georgoulis et al. (33) tested 13 patients with ACL deficient knees, twenty one patients with ACLR knees and ten control subjects with uninjured knees during walking using a 3D optoelectronic gait analysis system. They found that the ACLR knees can maintain tibial rotational stability. Iliopoulos et al. (32) summarized that nearly normalized locomotion economy was observed in anatomical ACLR with either hamstrings tendon or bone-patellar tendon-bone graft during flat, uphill, and downhill walking. Akpinar et al. (34) concluded no significant difference of knee kinematics was observed between anatomic ACLR knees and native knees at 24-month follow-up during downhill running. As was consistent with previous studies (32,34), our results also showed that anatomical ACLR can restore knee kinematics during level walking. However, as was demonstrated by Figure 9, the non-anatomical ACLR in our study can also restore knee kinematics during level walking. This phenomenon may be potentially explained by the following reasons. As was displayed in Figure 2C, the centers of non-anatomical tunnel aperture located closely to the standard anatomical area indicating minor discrepancy of placement. Williams et al. (35) tested the pre-surgery to post-surgery ipsilateral comparisons of neuromuscular function after ACLR. They found that the improvement of voluntary muscle control occurred in most muscles after ACLR. The anterolateral ligament (ALL) of knee has been considered to play a potential role in maintaining the knee rotational stability (36) together with ACL and protective effect on ACLR (37). Thus, the compensation from improved voluntary muscle control and native ALL may counteract the minor discrepancy of tunnel location. In our study, the majority of tunnel apertures were located within the area of 25–50% (x) of distance t and 25–50% (y) of distance h (Figure 2C). This area can be assumed as an acceptable region for femoral tunnel aperture in gait kinematics perspective. Of course, more cases should be further included to verify this assumption.
Compared with non-anatomical reconstruction group, the ACL graft orientation of anatomical reconstruction group was closer to the reported native ACL orientation (38) either in coronal plane or sagittal plane, thus better simulating the native ACL fibers to function in terms of graft orientation. Vignos et al. (39) reported that the non-anatomic graft geometry was associated with the asymmetric knee kinematics and cartilage contact pattern. As shown in our results, the AR group can restore ROM of 6 DOF, while, in Non-AR group, the ROM of anterior-posterior translation of ACLR knees was significantly larger than that of contralateral knees by approximately 0.5 cm, thus potentially leading to the alterations of cartilage contact pattern and joint congruence. The in vitro and animal studies have demonstrated that cartilage degeneration can be initiated by the alterations of cartilage mechanics (40,41). Furthermore, Marchant et al. (6) reported that the non-anatomic tunnel placement prevailed among the knees of failed or revision ACLR.
However, some studies have reported that kinematics alterations may be one of the possible reasons for subsequent joint degenerations after ACLR, even though the static anterior stability was maintained (17,42,43). There were some studies on kinematics of knees after ACLR, under various loading conditions. Yoo et al. (44) tested the effect of ACLR on knee kinematics, using eight human cadaveric knees on a robotic testing system. Their results showed that rotational stability may not be restored under simulated physiological loading conditions, even though anterior stability achieved clinical satisfaction. Another study evaluated the kinematics of ACLR knees and contralateral normal knees for six patients during downhill running. More external rotation and adduction were observed in ACLR knees, compared with contralateral knees (43). Furthermore, some other studies have also shown the ACLR knees may not maintain normal rotational stability under high demanding activities, such as a combined descending and pivoting activity (45) or single leg weight-bearing lunge (42). However, most of those studies were performed with cadaveric knees, software simulation or under high demanding activities that may lead to the difference of results between our study and their reports.
Some limitations still exist in this study. We used the reported standard area for anatomical ACL footprint rather than the contralateral normal ACL footprint for the determination of tunnel placement. Second, this study was limited to gait kinematics testing at the speed of 3.0 km/h. Advanced kinematics testing under different states of motion should be further evaluated, because the function demanding for ACL fibers may be different. Third, the results in our study can only reflect the early stage gait kinematics after ACLR, with mean follow-up time of nine months for AR group and ten months for Non-AR group. Further studies should be performed to evaluate the gait kinematics after ACLR during different follow-up times. Fourth, different types of meniscal injuries or treatments may result in gait alterations. But more detailed subgroups were not performed due to the limited number of patients in our study. For advanced comprehension of the effect of meniscal injuries or treatments accompanied ACLR on gait kinematics, further studies are required. Finally, the accuracy of acquired data may be limited by this skin marker-based optoelectronic gait analysis system.
Conclusions
The anatomical ACLR can restore close to normal gait kinematics and ROM of 6 DOF kinematics compared with non-anatomical ACLR. The ACL graft after anatomical ACLR simulated native ACL fibers to function in terms of graft orientation.
Acknowledgments
Funding: We thank the volunteers who participated in this study. This work was supported by National Key R&D Program of China (2018YFC1105904), Natural Science Foundation of Jiangsu Province, China (BK20180127), Key Program of NSFC (81730067), Excellent Young Scholars NSFC (81622033), National Science Foundation of China (81802196), Social Development Project of Jiangsu Provincial Science and Technology Department (BE2016609).
Footnote
Conflicts of Interest: The authors have no conflicts of interest to declare.
Ethical Statement: The authors are accountable for all aspects of the work in ensuring that questions related to the accuracy or integrity of any part of the work are appropriately investigated and resolved. The study was approved by the Institutional Review Board of Nanjing Drum Tower Hospital (2019-024-01) and Written informed consent was obtained from the patient for publication of this manuscript and any accompanying images.
References
- Andersen HN, Dyhre-Poulsen P. The anterior cruciate ligament does play a role in controlling axial rotation in the knee. Knee Surg Sports Traumatol Arthrosc 1997;5:145-9. [Crossref] [PubMed]
- Siegel L, Vandenakker-Albanese C, Siegel D. Anterior cruciate ligament injuries: anatomy, physiology, biomechanics, and management. Clin J Sport Med 2012;22:349-55. [Crossref] [PubMed]
- Zhang Y, Huang W, Yao Z, et al. Anterior Cruciate Ligament Injuries Alter the Kinematics of Knees With or Without Meniscal Deficiency. Am J Sports Med 2016;44:3132-9. [Crossref] [PubMed]
- Levy BA. Is early reconstruction necessary for all anterior cruciate ligament tears? N Engl J Med 2010;363:386-8. [Crossref] [PubMed]
- Fithian DC, Paxton EW, Stone ML, et al. Prospective trial of a treatment algorithm for the management of the anterior cruciate ligament-injured knee. Am J Sports Med 2005;33:335-46. [Crossref] [PubMed]
- Marchant BG, Noyes FR, Barber-Westin SD, et al. Prevalence of nonanatomical graft placement in a series of failed anterior cruciate ligament reconstructions. Am J Sports Med 2010;38:1987-96. [Crossref] [PubMed]
- Steiner ME, Battaglia TC, Heming JF, et al. Independent drilling outperforms conventional transtibial drilling in anterior cruciate ligament reconstruction. Am J Sports Med 2009;37:1912-9. [Crossref] [PubMed]
- Alentorn-Geli E, Samitier G, Alvarez P, et al. Anteromedial portal versus transtibial drilling techniques in ACL reconstruction: a blinded cross-sectional study at two- to five-year follow-up. Int Orthop 2010;34:747-54. [Crossref] [PubMed]
- Kim MK, Lee BC, Park JH. Anatomic single bundle anterior cruciate ligament reconstruction by the two anteromedial portal method: the comparison of transportal and transtibial techniques. Knee Surg Relat Res 2011;23:213-9. [Crossref] [PubMed]
- Mardani-Kivi M, Madadi F, Keyhani S, et al. Antero-medial portal vs. transtibial techniques for drilling femoral tunnel in ACL reconstruction using 4-strand hamstring tendon: a cross-sectional study with 1-year follow-up. Med Sci Monit 2012;18:CR674-9. [Crossref] [PubMed]
- Tudisco C, Bisicchia S. Drilling the femoral tunnel during ACL reconstruction: transtibial versus anteromedial portal techniques. Orthopedics 2012;35:e1166-72. [Crossref] [PubMed]
- Waite JC, Beard DJ, Dodd CA, et al. In vivo kinematics of the ACL-deficient limb during running and cutting. Knee Surg Sports Traumatol Arthrosc 2005;13:377-84. [Crossref] [PubMed]
- Yeung MY, Fu SC, Chua EN, et al. Use of a portable motion analysis system for knee dynamic stability assessment in anterior cruciate ligament deficiency during single-legged hop landing. Asia Pac J Sports Med Arthrosc Rehabil Technol 2016;5:6-12. [PubMed]
- Lam MH, Fong DT, Yung P, et al. Knee stability assessment on anterior cruciate ligament injury: Clinical and biomechanical approaches. Sports Med Arthrosc Rehabil Ther Technol 2009;1:20. [PubMed]
- Mousavi SH, Hijmans JM, Rajabi R, et al. Kinematic risk factors for lower limb tendinopathy in distance runners: A systematic review and meta-analysis. Gait Posture 2019;69:13-24. [Crossref] [PubMed]
- Imhauser C, Mauro C, Choi D, et al. Abnormal tibiofemoral contact stress and its association with altered kinematics after center-center anterior cruciate ligament reconstruction: an in vitro study. Am J Sports Med 2013;41:815-25. [Crossref] [PubMed]
- Andriacchi TP, Favre J. The nature of in vivo mechanical signals that influence cartilage health and progression to knee osteoarthritis. Curr Rheumatol Rep 2014;16:463. [Crossref] [PubMed]
- Howell SM, Berns GS, Farley TE. Unimpinged and impinged anterior cruciate ligament grafts: MR signal intensity measurements. Radiology 1991;179:639-43. [Crossref] [PubMed]
- Musahl V, Plakseychuk A, VanScyoc A, et al. Varying femoral tunnels between the anatomical footprint and isometric positions: effect on kinematics of the anterior cruciate ligament-reconstructed knee. Am J Sports Med 2005;33:712-8. [Crossref] [PubMed]
- Brophy RH, Selby RM, Altchek DW. Anterior cruciate ligament revision: double-bundle augmentation of primary vertical graft. Arthroscopy 2006;22:683.e1-5. [Crossref] [PubMed]
- Howell SM, Clark JA. Tibial tunnel placement in anterior cruciate ligament reconstructions and graft impingement. Clin Orthop Relat Res 1992.187-95. [PubMed]
- Zantop T, Diermann N, Schumacher T, et al. Anatomical and nonanatomical double-bundle anterior cruciate ligament reconstruction: importance of femoral tunnel location on knee kinematics. Am J Sports Med 2008;36:678-85. [Crossref] [PubMed]
- Lubowitz JH, Bernardini BJ, Reid JB 3rd. Current concepts review: comprehensive physical examination for instability of the knee. Am J Sports Med 2008;36:577-94. [Crossref] [PubMed]
- Bernard M, Hertel P, Hornung H, et al. Femoral insertion of the ACL. Radiographic quadrant method. Am J Knee Surg 1997;10:14-21; discussion 21-2. [PubMed]
- Amis AA, Jakob RP. Anterior cruciate ligament graft positioning, tensioning and twisting. Knee Surg Sports Traumatol Arthrosc 1998;6 Suppl 1:S2-12. [Crossref] [PubMed]
- Xu H, Zhang C, Zhang Q, et al. A Systematic Review of Anterior Cruciate Ligament Femoral Footprint Location Evaluated by Quadrant Method for Single-Bundle and Double-Bundle Anatomic Reconstruction. Arthroscopy 2016;32:1724-34. [Crossref] [PubMed]
- Scanlan SF, Blazek K, Chaudhari AM, et al. Graft orientation influences the knee flexion moment during walking in patients with anterior cruciate ligament reconstruction. Am J Sports Med 2009;37:2173-8. [Crossref] [PubMed]
- Benedetti MG, Catani F, Leardini A, et al. Data management in gait analysis for clinical applications. Clin Biomech (Bristol, Avon) 1998;13:204-15. [Crossref] [PubMed]
- Pataky TC. One-dimensional statistical parametric mapping in Python. Comput Methods Biomech Biomed Engin 2012;15:295-301. [Crossref] [PubMed]
- Papageorgiou CD, Gil JE, Kanamori A, et al. The biomechanical interdependence between the anterior cruciate ligament replacement graft and the medial meniscus. Am J Sports Med 2001;29:226-31. [Crossref] [PubMed]
- Hall M, Stevermer CA, Gillette JC. Gait analysis post anterior cruciate ligament reconstruction: knee osteoarthritis perspective. Gait Posture 2012;36:56-60. [Crossref] [PubMed]
- Iliopoulos E, Galanis N, Zafeiridis A, et al. Anatomic single-bundle anterior cruciate ligament reconstruction improves walking economy: hamstrings tendon versus patellar tendon grafts. Knee Surg Sports Traumatol Arthrosc 2017;25:3155-62. [Crossref] [PubMed]
- Georgoulis AD, Papadonikolakis A, Papageorgiou CD, et al. Three-dimensional tibiofemoral kinematics of the anterior cruciate ligament-deficient and reconstructed knee during walking. Am J Sports Med 2003;31:75-9. [Crossref] [PubMed]
- Akpinar B, Thorhauer E, Irrgang JJ, et al. Alteration of Knee Kinematics After Anatomic Anterior Cruciate Ligament Reconstruction Is Dependent on Associated Meniscal Injury. Am J Sports Med 2018;46:1158-65. [Crossref] [PubMed]
- Williams GN, Snyder-Mackler L, Barrance PJ, et al. Neuromuscular function after anterior cruciate ligament reconstruction with autologous semitendinosus-gracilis graft. J Electromyogr Kinesiol 2005;15:170-80. [Crossref] [PubMed]
- Patel RM, Brophy RH. Anterolateral Ligament of the Knee: Anatomy, Function, Imaging, and Treatment. Am J Sports Med 2018;46:217-23. [Crossref] [PubMed]
- Delaloye JR, Murar J, Vieira TD, et al. Combined Anterior Cruciate Ligament Repair and Anterolateral Ligament Reconstruction. Arthrosc Tech 2018;8:e23-9. [Crossref] [PubMed]
- Ahn JH, Lee SH, Yoo JC, et al. Measurement of the graft angles for the anterior cruciate ligament reconstruction with transtibial technique using postoperative magnetic resonance imaging in comparative study. Knee Surg Sports Traumatol Arthrosc 2007;15:1293-300. [Crossref] [PubMed]
- Vignos MF, Kaiser JM, Baer GS, et al. American Society of Biomechanics Clinical Biomechanics Award 2017: Non-anatomic graft geometry is linked with asymmetric tibiofemoral kinematics and cartilage contact following anterior cruciate ligament reconstruction. Clin Biomech (Bristol, Avon) 2018;56:75-83. [Crossref] [PubMed]
- Griffin TM, Guilak F. The role of mechanical loading in the onset and progression of osteoarthritis. Exerc Sport Sci Rev 2005;33:195-200. [Crossref] [PubMed]
- Beveridge JE, Heard BJ, Shrive NG, et al. Tibiofemoral centroid velocity correlates more consistently with cartilage damage than does contact path length in two ovine models of stifle injury. J Orthop Res 2013;31:1745-56. [PubMed]
- Papannagari R, Gill TJ, Defrate LE, et al. In vivo kinematics of the knee after anterior cruciate ligament reconstruction: a clinical and functional evaluation. Am J Sports Med 2006;34:2006-12. [Crossref] [PubMed]
- Tashman S, Collon D, Anderson K, et al. Abnormal rotational knee motion during running after anterior cruciate ligament reconstruction. Am J Sports Med 2004;32:975-83. [Crossref] [PubMed]
- Yoo JD, Papannagari R, Park SE, et al. The effect of anterior cruciate ligament reconstruction on knee joint kinematics under simulated muscle loads. Am J Sports Med 2005;33:240-6. [Crossref] [PubMed]
- Ristanis S, Giakas G, Papageorgiou CD, et al. The effects of anterior cruciate ligament reconstruction on tibial rotation during pivoting after descending stairs. Knee Surg Sports Traumatol Arthrosc 2003;11:360-5. [Crossref] [PubMed]