Microcirculation and mean arterial pressure: friends or foes?
Since the landmark paper by De Backer et al. (1), numerous studies have confirmed the presence of microcirculatory dysfunction in septic shock, and established its pathophysiological mechanisms and prognostic impact (2-4). A controversial issue is the potential dissociation between microcirculation and macrohemodynamics in shock states (5). This is a fundamental issue with potential implications in the selection of the most appropriate resuscitation target (6). Sublingual microcirculation, which is easy to access with video-microscopic techniques (7), is one of the most frequently explored territory in humans. However, these techniques are performed for research purposes only. Recently, much emphasis has been put on assessment of peripheral perfusion (8) using skin perfusion markers such as mottling score (9) or capillary refill time recently proposed to guide resuscitation in early septic shock (10,11).
Kazune et al. (12) also proposed to assess skin perfusion of the knee by using the hyperspectral imaging (HSI) technique, which is derived from the characteristic discoloration in mottling, created by the pattern of light reflected from the skin surface. This discoloration reflects the relative concentration and distribution of chromophores, including oxy- and deoxyhemoglobin contained in the microcirculation. As the light absorption properties of these chromophores are known, measurements of diffuse reflectance spectra can be used to determine their relative concentrations. The HSI uses a camera combining digital photography with measurement of reflected light intensity at multiple spectral bands for each pixel and enabling precise mapping of oxy- and deoxyhemoglobin distribution in the dermal layer of the skin. It is admitted that when skin microcirculation is heterogeneous, hyperspectral images allow to identify poorly oxygenated areas.
Using HIS, Kazune et al. aimed to assess the effects of NE-induced increase in mean arterial pressure (MAP) on microcirculatory oxygen saturation in hypoxic skin areas in patients with sepsis or septic shock (12). The optimal MAP target during septic shock is still a matter of debate. Although there is a general agreement to initially target at least 65 mmHg (13), there is no consensus regarding a maximal value ofMAP not to exceed and above which a further increase in MAP would be harmful (6,14) in particular on microcirculation due to excessive vasoconstrictive effects of increasing doses of NE required to reach the target. In their single-center interventional prospective study, Kazune et al. included 28 patients and 15 healthy volunteers (12). During the first four hours of resuscitation, all the patients had received at least 20 mL/kg of crystalloids and NE when MAP could not reach 65 mmHg despite fluid administration. At the study inclusion, MAP was 74 [69–79] mmHg in the subgroup of 13 septic patients (requiring no NE) and 65 [64–67] mmHg in the subgroup of 15 septic shock patients, who required a median NE dose of 0.1 [0.06–0.25] µg/kg/min. After the initial measurements of skin oxygen saturation (µHbO2) by HSI, the dose of NE was increased by 0.05 µg/kg/min every 10 minutes to increase MAP by 20±5 mmHg, and then µHbO2 was recorded again. For the whole population of patients (n=28), the final MAP was 85 [83–90] mmHg on average. Five important results were reported. Firstly, the baseline skin µHbO2 in sepsis and septic shock patients was 35% and 18%, respectively, and were significantly lower than those of healthy volunteers (µHbO2 >80%). Secondly, higher severity of organ failure evaluated by SOFA score was associated with lower µHbO2 values. Thirdly, skin µHbO2 increased from 26.0% (24.5–27.0%) at baseline to 30.0% (29.0–31.0%) after NE dose adjustment (P=0.04) suggesting a global improvement of skin microcirculation along with the increase in MAP. Change in skin µHbO2 did not differ between the sepsis and septic shock groups (P=0.69). Fourthly, lower baseline skin µHbO2 was significantly associated with higher illness severity as evaluated by SOFA score. Fifthly, the increase in skin µHbO2 was related to illness severity so that in patients with higher SOFA scores, a higher µHbO2 after MAP augmentation was observed (12). This suggests that the improvement of µHbO2 after NE-induced increase in MAP is mostly observed when baseline microcirculation is severely impaired. Based on these results, the authors concluded that in septic shock HIS and that this effect seems to be more pronounced in severely ill patients (12).
This prospective interventional study (12) provides another piece of evidence on the existence of some hemodynamic association between macrohemodynamics and microcirculation during the early resuscitation of septic patients. Indeed, fluid infusion was previously reported to improve sublingual microvascular perfusion in the early phase of resuscitation of septic shock but not in a later phase (2). In patients with severe sepsis or septic shock examined within the first 24 hours of their admission, Pottecher et al. found that both passive leg raising and fluid infusion increased improved sublingual microcirculatory perfusion variables in parallel to the increase in cardiac output (15) suggesting that no strong dissociation between macrohemodynamics and sublingual microcirculation existed in early sepsis.
Moreover, in a previous study, our group examined the consequences of NE administration on muscle tissue oxygenation (StO2) in 28 severely hypotensive septic shock patients (16). The StO2 and its changes during a vascular occlusion test (VOT) were measured at the level of the thenar eminence using near-infrared spectroscopy (NIRS). NE administration was associated with increased MAP (from 54 to 77 mmHg on average) and improvement of NIRS variables (16). This suggests that restoring MAP with NE may improve rather than worsen tissue oxygenation in severely hypotensive septic patients.
Interestingly, in the study by Kazune et al. (12) MAP was increased by 20 mmHg after that the patients had been already resuscitated and their MAP already at the initial target (65 mmHg) usually recommended by experts (13). Similar results were previously found in another series of patients with septic shock, in whom increasing MAP with NE from 65 to 85 mmHg, was associated with increase in cardiac output, decrease in blood lactate concentration, and improvement of the sublingual microcirculatory variables and improvement of the StO2 response to a VOT (17). Another recent study confirmed these findings by showing a significantly increase in sublingual microcirculation variables when MAP was increased from around 65 to 85–90 mmHg with NE in a series of 40 patients with septic shock (18). Taken together, results of these studies (12,17,18) suggest that the initial MAP target (65 mmHg) may be not sufficient and that a higher MAP would be advised in the majority of septic patients. Another interesting finding of the study by Kazune et al. (12) is the interindividual variability in the response of skin µHbO2 to the NE-induced increase in MAP, depending in part on the state of the baseline skin µHbO2. This finding is in agreement with data previously reported by Dubin et al. (19), who showed that increasing MAP (with NE) from 65 to 75 mmHg and then to 85 mmHg during septic shock improved sublingual microcirculation only in patients with impaired sublingual microcirculation at baseline. All these findings argue in favor of individualizing the MAP target and of taking into account peripheral perfusion indices to find the optimal MAP value. Performing a vasopressor challenge and assessing its result using a peripheral perfusion marker (10,11,20) could be helpful for that purpose.
Nevertheless, there are still some concerns about the study by Kazune et al. and caution is required when interpreting their results (12).
Firstly, authors did not provide convincing arguments about the reason why septic patients who had a MAP above 65 mmHg without NE were included in the study and received NE with the aim to further increase MAP by 20 mmHg. Nevertheless, the practice of a vasopressor test is common nowadays. It consists in increasing MAP with NE to see what could happen in terms of effectiveness (using predefined endpoints) and safety (10,11,20). Secondly, even if higher MAP was associated with higher skin µHbO2, no definitive conclusion can be drawn in terms of optimal MAP to target since microcirculation was not explored in other territories. One could not exclude that increasing doses of NE could have impaired perfusion of other important organs and/or have induced myocardial cell toxicity, oxidative stress or other side effects (21). Thirdly, the authors chose an arbitrary value of 20 mmHg for the increase in MAP and no justification of this choice was made. Nevertheless, in the group of patients with septic shock the final MAP value was around 85 mmHg and thus similar to the values obtained in previous studies that investigated the response of microcirculation to NE-induced MAP increase (17-19). Fourthly, the results were not analyzed in function of the existence of history of chronic hypertension. We learnt from a previous multicentric randomized controlled trial that in patients with chronic hypertension targeting MAP of 80–85 mmHg (compared to 65–70 mmHg) resulted in benefits in terms of renal function (including requirement of renal replacement therapy) (22). It would have been interesting to examine whether increases in MAP by 20 mmHg can result in more pronounced increases in skin µHbO2 in patients with chronic hypertension compared to patients with no prior hypertension. However, in a recent study in patients with septic shock, increasing MAP from to 65 to 85 mmHg resulted in a similar improvement in sublingual microcirculation in patients with chronic hypertension vs. no prior hypertension (18). Fifthly, the authors chose HIS to assess skin perfusion. Unfortunately, they did not explore other techniques to assess peripheral microcirculation or tissue oxygenation (8,23) (Table 1). Some of these techniques assess microcirculatory blood flow either directly by measuring it (videomicroscopy, laser Doppler) or indirectly by measuring tissue carbon dioxide pressure (PCO2) (and comparing it to arterial blood PCO2) (Table 1). Interestingly, videomicroscopy also provides additional information on microcirculation such as proportion of perfused vessels, perfused microvessels density and heterogeneity index (7). Other techniques assess tissue oxygenation (transcutaneous oxygen tension, NIRS StO2 and its response to a VOT) (Table 1). It is noteworthy that the majority of these techniques are currently restricted to research, and their routine use still needs further investigation (23). By contrast, other markers of skin perfusion such as the capillary refill time or the mottling score can be used routinely as they both do not need sophisticated devices (8). Capillary refill time has been recently proposed to guide hemodynamic resuscitation of early septic shock in a multicenter randomized controlled trial (ANDROMEDA-SHOCK trial) (10) with very promising results compared to a resuscitation strategy guided by lactate clearance (11). Unfortunately, capillary refill time was not assessed in the Kazune et al. study, which however, provided mottling score values (12). The mottling score is a semi-quantitative assessment tool, which was reported to well correlate with tissue perfusion variables and to be a strong predictor of mortality in septic patients (9). However, in the study by Kazune et al., the mottling score was 0 (no visible mottled skin) in 79% of the total population of septic patients and very importantly in 67% of the patients with septic shock (12). Another recent study from the same group investigated in 89 septic patients, the relationship between the mottling score and skin µHbO2 measured by HSI and showed that there is a large range of µHbO2 values while the mottling score was 0 or 1 (24). They also showed that low skin µHbO2 was more specific than high mottling score to predict mortality (24). Taken together, these findings suggest that assessment of skin perfusion using HIS can find abnormalities that cannot be easily detected by the mottling score. It is clear that confirmation by further studies is required.
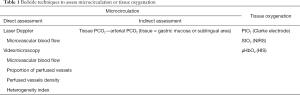
Full table
In conclusion, the study by Kazune et al. (12), provided evidence for a certain degree of coherence between macrocirculation and microcirculation during early hemodynamic resuscitation of septic patients. It also could reassure physicians about the safety of reaching MAP around 85 mmHg with NE during early resuscitation of septic shock. Another key message of the study is the variable effect of NE-induced increase in MAP on skin oxygenation in part depending on the degree of severity at baseline. This suggests the need of individualization of the vasopressor therapy and the use of peripheral perfusion/oxygenation indices to help defining the optimal MAP target. Whether HIS is a relevant technique to assess peripheral oxygenation and can be routinely used to guide hemodynamic resuscitation needs to be further explored in larger studies.
Acknowledgments
Funding: None.
Footnote
Conflicts of Interest: Both authors have completed the ICMJE uniform disclosure form (available at http://dx.doi.org/10.21037/atm.2020.01.70). The series “Hemodynamic Monitoring in Critically Ill Patients” was commissioned by the editorial office without any funding or sponsorship. The authors have no other conflicts of interest to declare.
Ethical Statements: The authors are accountable for all aspects of the work in ensuring that questions related to the accuracy or integrity of any part of the work are appropriately investigated and resolved.
Open Access Statement: This is an Open Access article distributed in accordance with the Creative Commons Attribution-NonCommercial-NoDerivs 4.0 International License (CC BY-NC-ND 4.0), which permits the non-commercial replication and distribution of the article with the strict proviso that no changes or edits are made and the original work is properly cited (including links to both the formal publication through the relevant DOI and the license). See: https://creativecommons.org/licenses/by-nc-nd/4.0/.
References
- De Backer D, Creteur J, Preiser JC, et al. Microvascular blood flow is altered In patients with sepsis. Am J Respir Crit Care Med 2002;166:98-104. [Crossref] [PubMed]
- De Backer D, Donadello K, Sakr Y, et al. Microcirculatory alterations in patients with severe sepsis: impact of time of assessment and relationship with outcome. Crit Care Med 2013;41:791-9. [Crossref] [PubMed]
- Orbegozo D, Mongkolpun W, Stringari G, et al. Skin microcirculatory reactivity assessed using a thermal challenge is decreased in patients with circulatory shock and associated with outcome. Ann Intensive Care 2018;8:60. [Crossref] [PubMed]
- Scorcella C, Damiani E, Domizi R, et al. A. MicroDAIMON study: Microcirculatory DAIly MONitoring in critically ill patients: a prospective observational study. Ann Intensive Care 2018;8:64. [Crossref] [PubMed]
- Ince C. Hemodynamic coherence and the rationale for monitoring the microcirculation. Crit Care 2015;19:S8. [Crossref] [PubMed]
- Lesur O, Delile E, Asfar P, et al. Hemodynamic support in the early phase of septic shock: a review of challenges and unanswered questions. Ann Intensive Care 2018;8:102. [Crossref] [PubMed]
- Ince C, Boerma EC, Cecconi M, et al. Second consensus on the assessment of sublingual microcirculation in critically ill patients: results from a task force of the European Society of Intensive Care Medicine. Intensive Care Med 2018;44:281-99. [Crossref] [PubMed]
- Hariri G, Joffre J, Leblanc G, et al. Narrative review: clinical assessment of peripheral tissue perfusion in septic shock. Ann Intensive Care 2019;9:37. [Crossref] [PubMed]
- Dumas G, Lavillegrand JR, Joffre J, et al. Mottling score is a strong predictor of 14-day mortality in septic patients whatever vasopressor doses and other tissue perfusion parameters. Crit Care 2019;23:211. [Crossref] [PubMed]
- Hernández G, Cavalcanti AB, Ospina-Tascón G, et al. Early goal-directed therapy using a physiological holistic view: the ANDROMEDA-SHOCK-a randomized controlled trial. Ann Intensive Care 2018;8:52. [Crossref] [PubMed]
- Hernández G, Ospina-Tascón GA, Damiani LP, et al. Effect of a resuscitation strategy targeting peripheral perfusion status vs serum lactate levels on 28-day mortality among patients with septic shock: the ANDROMEDA-SHOCK randomized clinical trial. JAMA 2019;321:654-64. [Crossref] [PubMed]
- Kazune S, Caica A, Luksevics E, et al. Impact of increased mean arterial pressure on skin microcirculatory oxygenation in vasopressor-requiring septic patients: an interventional study. Ann Intensive Care 2019;9:97. [Crossref] [PubMed]
- Scheeren TWL, Bakker J, De Backer D, et al. Current use of vasopressors in septic shock. Ann Intensive Care 2019;9:20. [Crossref] [PubMed]
- Khanna AK. Defending a mean arterial pressure in the intensive care unit: Are we there yet? Ann Intensive Care 2018;8:116. [Crossref] [PubMed]
- Pottecher J, Deruddre S, Teboul JL, et al. Both passive leg raising and intravascular volume expansion improve sublingual microcirculatory perfusion in severe sepsis and septic shock patients. Intensive Care Med 2010;36:1867-74. [Crossref] [PubMed]
- Georger JF, Hamzaoui O, Chaari A, et al. Restoring arterial pressure with norepinephrine improves muscle tissue oxygenation assessed by near-infrared spectroscopy in severely hypotensive septic patients. Intensive Care Med 2010;36:1882-9. [Crossref] [PubMed]
- Fiorese Coimbra KT, de Freitas FGR, Bafi AT, Pinheiro TT, Nunes NF, de Azevedo LCP, Machado FR. Effect of increasing blood pressure with noradrenaline on microcirculation of patients with septic shock and previous arterial hypertension. Crit Care Med 2019;47:1033-40. [Crossref] [PubMed]
- Thooft A, Favory R, Salgado DR, et al. Effects of changes in arterial pressure on organ perfusion during septic shock. Crit Care 2011;15:R222. [Crossref] [PubMed]
- Dubin A, Pozo MO, Casabella CA, et al. Increasing arterial blood pressure with norepinephrine does not improve microcirculatory blood flow: a prospective study. Crit Care 2009;13:R92. [Crossref] [PubMed]
- Legrand M, De Backer D, Dépret F, et al. Recruiting the microcirculation in septic shock. Ann Intensive Care 2019;9:102. [Crossref] [PubMed]
- Hernandez G, Teboul JL, Bakker J. Norepinephrine in septic shock. Intensive Care Med 2019;45:687-9. [Crossref] [PubMed]
- Asfar P, Meziani F, Hamel JF, et al. High versus low blood-pressure target in patients with septic shock. N Engl J Med 2014;370:1583-93. [Crossref] [PubMed]
- De Backer D, Donadello K. Assessment of microperfusion in sepsis. Minerva Anestesiol 2015;81:533-40. [PubMed]
- Kazune S, Caica A, Volceka K, et al. Relationship of mottling score, skin microcirculatory perfusion indices and biomarkers of endothelial dysfunction in patients with septic shock: an observational study. Crit Care 2019;23:311. [Crossref] [PubMed]