Clinical characteristics of perivascular space and brain CT perfusion in stroke-free patients with intracranial and extracranial atherosclerosis of different extents
Introduction
Perivascular space (PVS) refers to a fluid-filled space that traverses the distribution of blood vessels across the gray matter and white matter of the brain (1). Around the cortical arteries at the centrum ovale, PVS is composed of monolayer pia mater; around the cortical arteries at the basal ganglia, PVS is composed of double-layered pia mater; the veinlets in the cortex and basal ganglia are not capsulated by the pia mater, and thus the PVS directly communicates with the subdural space, but the PVS disappears when it reaches the capillary bed (2).
Studies have shown that PVS is associated with brain atrophy (including the cortical and central atrophy) (3), β amyloid protein deposition (4), dysfunction of intracranial vascular endothelial and inflammatory response (5), heritability (6), age and hypertension (7). van Swieten et al. found that PVS was not associated with cerebral atherosclerosis of nine dilated PVS (8), but Gutierrez et al. revealed for the first time that non-stenotic carotid plaques were associated with PVS (9). Thus, the relationship between atherosclerosis and PVS is still controversial.
Little is known about whether the decline in the cerebral blood flow (CBF) caused by arteriosclerosis is related to the PVS. To date, various methods have been employed to clinically determine the CBF, and the most common methods are CT and magnetic resonance imaging (MRI). Computed tomography perfusion (CTP) has been widely used in clinical practice due to the convenient operation, fast imaging, low cost and favorable tolerance. Arterial spin labeling (ASL) in MRI does not require the use of contrast agents, but white matter perfusion is often underestimated and ASL is highly sensitive to the motion of the subject. Blood oxygen level-dependent functional magnetic resonance imaging (BOLD-fMRI) can indirectly reflect CBF by detecting the blood oxygen content in the brain. It has high spatial and temporal resolution, but is often affected by the oxyhemoglobin and nerve activity.
PVS is not a focal lesion and both hemispheres are often symmetrically involved. The focal intra or extracranial arteriosclerosis usually causes damage to the structure and function of focal brain tissues. We speculate that the overall cerebral arteriosclerosis has a strong relationship with PVS. The increased intracranial and extracranial arteriosclerosis may involve the cerebral arterioles, especially the arterioles close to the carotid artery in the basal ganglia. The arterial plaques may damage the wall of arterioles, reduce the CBF and increase the interstitial fluid around the PVS, leading to the expansion of the PVS. This study was conducted to investigate the exposure factors related to PVS, and to assess the relationship among intracranial/extracranial atherosclerosis, CBF and PVS in stroke-free patients. Our findings may provide evidence on the etiology of PVS.
Methods
Inclusion criteria and exclusion criteria
From January 2016 to October 2018, 222 patients with no history of stroke were consecutively recruited from the Department of Neurology Stroke Screening Clinic in Tongji Hospital of Shanghai. Informed consent was obtained from each patient before study. There were 101 males and 121 females with the mean age of 69.55±9.81 years (range, 35–94 years).
The inclusion criteria were as follows: (I) patients were admitted due to the complaints of “dizziness, headache, limb numbness and transient limb weakness”, including those with transient ischemic attack (TIA); (II) all patients underwent brain CTP, computed tomography angiography (CTA), MRI and carotid ultrasonography, which were done within 1 week after initial admission; (III) all patients had complete medical record; (IV) informed consent was obtained.
The exclusion criteria were as follows: (I) patients had previous or new non-lacunar ischemic stroke or cerebral hemorrhage; (II) patients had lacunar infarction with >2 lesions; (III) patients had a history of Alzheimer’s disease, atrial fibrillation, mental illness, severe anxiety and depression, and severe Parkinson’s disease; (IV) patients received placement of metal stents and pacemakers, or had severe visual impairment/aphasia; (V) patients had serious infections, organ failure, brain trauma and tumors; (VI) the medical record was incomplete.
Study methods
Carotid ultrasonography and scoring of extracranial atherosclerosis
Carotid artery ultrasonography was performed with the Sonosite X-Porte color Doppler ultrasound diagnostic apparatus (USA) equipped with 5–10 MHz linear array probe. All patients were examined by a full-time, experienced ultrasound doctor, the carotid artery was scanned and the dynamic and static images were stored according to standardized procedures. All the images were analyzed by two experienced neurologists who were blind to the study. Patients lied in a supine position and the side neck was exposed. The blood vessels were scanned, and following parameters were measured: (I) carotid intima-media thickness (IMT) (vertical distance from the leading edge of the endovascular interface to the medial-adventitial interface leading edge) was measured at the internal carotid artery (ICA), carotid bifurcation (BIF), common carotid artery (CCA) (about 1 cm of vessel at each site) (Figure 1A); the scanning angle was normalized, and the selected angle was: right side 90, 120 and 150; left side 210, 240 and 270. (II) The location, morphology, size and echo characteristics of carotid atherosclerotic plaques were also determined. A carotid plaque was defined as a structure that protrudes into the lumen with the intima-media thickness greater than or equal to 1.5 cm (10).
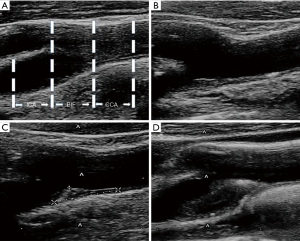
According to the Sutton-Tyrrell’s method (11), the extracranial arteriosclerosis was scored as follows: 0, IMT <1.5 mm; 1, one plaque (IMT: 1.5–2.0 mm); 2, one plaque or multiple plaques (one of the plaques IMT: 2.1–4.0 mm); 3, one large plaque (IMT: ≥4.1 mm). The sum score of extracranial arteriosclerosis was calculated as the sum of the bilateral carotid CCA, BIF, and ICA intravascular plaque scores (Figure 1B,C,D).
CTP and CTA scanning and scores of intracranial arteriosclerosis
The whole brain was examined by using an Aquilion One 320 row of dynamic volume CT (Toshiba, Japan). The upper edge of the aortic arch was scanned to the cranial apex. Following scanning parameters were used: voltage, 100 kV; current, 150–310 mA; matrix, 512×512; slice thickness, 0.5 mm; coverage, 14 cm. A double-tube high-pressure syringe was used to sequentially inject 50 mL of non-ionic contrast agent (350 mgI/mL Lohexol) and 30 mL of physiological saline through the right middle elbow vein at a rate of 5 mL/s. After injection, the scanning was initiated 7 s later, the arterial phase last for 2 s, the venous interval was 5 s, and the total scanning time was about 60 s. The whole brain dynamic volume data of 19 phases were obtained. After brain CTP, the non-ionic contrast agent (350 mgI/mL) was added at 0.6 mL/kg, and the aortic arch was scanned to the skull base by CTA. Images of the arteries and veins of the neck were obtained at an injection rate of 4.0 mL/s. The trigger threshold was 150 HU.
All volumetric data were imported into Toshiba’s 3D CTA software package, and the vascular subtraction imaging was performed automatically to obtain CTA three-dimensional image data. The morphology of intracranial arteries was analyzed and evaluated comprehensively. The intracranial arteries included the anterior cerebral artery (ACA), middle cerebral artery (MCA), posterior cerebral artery (PCA), intracranial segment of the internal carotid artery and intracranial segment of the vertebral basilar artery (VBA). Intracranial arteriosclerosis was analyzed according to Samuels method (12). Stenosis rate =(1– Ds/Dn) ×100% (Ds: intracranial artery stenosis diameter; Dn: normal diameter). The stenosis rate of any intracranial artery ≥50% was defined as intracranial atherosclerosis (Figure 2A). Then, the number of vessels with the stenosis rate of any intracranial artery greater than or equal to 50% was determined as the sum score of intracranial arteriosclerosis in each patient. In addition to the basilar artery, the number of intracranial stenotic arteries was also determined at both sides. The sum score of intracranial and extracranial arteriosclerosis was calculated as the sum of intracranial arteriosclerosis score and carotid plaque score.
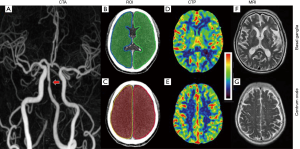
CBF acquisition
The volume data were analyzed using the Perfusion Mismatch Analyzer software (PMA version 5.0.5358.55864, http://asist.umin.jp/) to obtain a brain perfusion parameter map including the CBF. The PMA reconstructed perfusion map was imported into Mango software version 4.1 (http://rii.uthscsa.edu/mango/). The region of interest (ROI) was manually delineated in the centrum ovale layer with the largest number of PVS and in basal ganglia layer with the largest number of PVS, and the CBF of both sides was measured with the mirror method. The blood vessel and the sulcus return zone were avoided as much as possible in the measurements (Figure 2B,C,D,E).
Cranial MRI and PVS scoring
Cranial MRI was done by using the SiemensVerio 3.0T superconducting nuclear magnetic resonance equipment (Germany), with conventional T1WI, T2WI, FLAIR scanning (slice thickness: 5 mm; interval: 1 mm; field of view: 230 mm × 230 mm). The spin echo sequence was used: TR/TE, 1530/9 ms; the fast spin echo sequence was used in the T2WI: TR/TE, 4,210/96 ms; FLAIR: TR/TE/TI, 5,000/94/1,800 ms.
PVS was determined according to the following criteria: (I) depending on the image plane, the PVS was spotty or strip-like, with clear and smooth borders and usually <3 mm in diameter (13); (II) the PVS was consistent with the passage of the perforating vessels; (III) on all MRI sequences, the density of the PVS was equal to that of cerebrospinal fluid; (IV) there was no enhancement and occupancy effect. The Doubal’s method (13) was used to classify PVS into five grades by an experienced neurologist on T1WI, T2WI, and FLAIR images. The diameter and number of gaps around the tube were recorded on the T2WI. When measuring the size, the circular and elliptical gaps were selected to measure their maximum diameter, and the strip gap was used to measure the maximum diameter of the short axis. The layer with the largest number of PVS was selected independently in the centrum ovale and basal ganglia, and then centrum ovale and basal ganglia were further divided into four regions based on the left and right hemispheres. The PVS of each region was graded as follows: 0, none; 1, ≤10; 2, 11–20; 3, 21–40; 4, ≥40 (Figure 2F,G).
Statistical analysis
Continuous variables are expressed as mean ± standard deviation (
Results
Vascular risk factors, intracranial atherosclerosis, carotid plaque and medications in patients with and without PVS
The traditional vascular risk factors in these patients were as follows: hypertension (n=119), hyperlipidemia (n=115), diabetes mellitus (n=35), homocysteine (n=41), smoking (n=33), and drinking (n=25). Among them, 92 had intracranial artery stenosis, 122 had carotid plaque and 76 had coronary atherosclerotic heart disease. Of 222 patients, there were 173 patients in the PVS group and 49 patients in the non-PVS group. PVS patients were older (70.62±9.67 vs. 65.76±9.44, P<0.01) and had high incidences of intracranial arterial stenosis (49% vs. 16%, P<0.001), carotid plaque (65% vs. 18%, P<0.001) and coronary heart disease (39% vs. 18%, P<0.01) as compared to non-PVS patients. There were no significant differences in the hypertension, diabetes mellitus, hyperlipidemia, hyperhomocysteinemia, gender, smoking status and drinking status between PVS patients and non-PVS patients (P>0.05). In terms of medication, there was difference in the percentage of patients receiving treatment with statin between two groups (P<0.05). There was no significant difference in the percentage of patients receiving antiplatelet therapy, antihypertensive therapy, and glucose-lowering therapy between two groups (P>0.05) (Table 1). Logistic binary unconditional regression analysis showed that the risk factors of basal ganglia PVS were age, intracranial and extracranial atherosclerosis (Table 2), but there were no evident vascular risk factors for the PVS in the centrum ovale (Table 3).
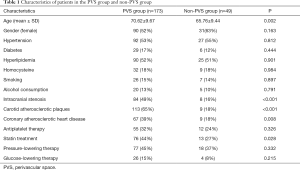
Full table
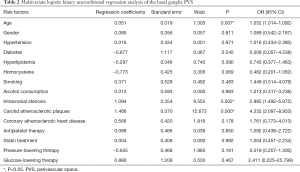
Full table
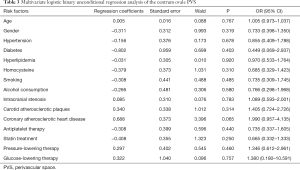
Full table
Incidence of cerebral PVS in patients with intracranial and/or extracranial atherosclerosis
According to CTA and carotid vascular ultrasonography, patients were divided into control group (no carotid plaque and no intracranial arteriosclerosis; n=70), carotid plaque group (presence of carotid plaque, but absence of intracranial atherosclerosis; CP group, n=60); intracranial atherosclerosis group without carotid plaques (IA group, n=30) ; carotid plaques and intracranial arterial stenosis group (CP + IA group, n=62). The incidences of cerebral PVS and basal ganglia PVS in patients with arteriosclerosis were significantly higher than in controls (cerebral PVS: IA group: 76.67%; CP group: 86.67%; CP + IA group: 98.39%; control group: 52.86%), (basal ganglia PVS: IA group: 60.00%; CP group: 68.33%; CP + IA group: 85.48%; control group: 28.57%). The incidence of centrum ovale PVS in patients of CP group, IA group, CP + IA group was similar to that in control group. The incidence of PVS in the whole brain, centrum ovale and basal ganglia was comparable between CP group and IA group. The incidences of PVS in the whole brain and basal ganglia of patients in the CP + IA group were significantly higher than in the IA group (98.39% vs. 76.67%, 85.48% vs. 60.00%; P<0.05) and CP group (98.39% vs. 86.67%, 85.48% vs. 68.33%, P<0.05), but there was no marked difference in the incidence of centrum ovale PVS (Figure 3A).
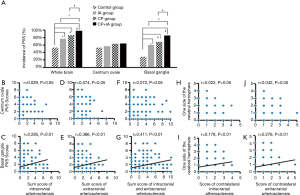
The score of basal ganglia PVS was positively related to the scores of intracranial arteriosclerosis, extracranial arteriosclerosis, intracranial and extracranial arteriosclerosis (r=0.285, 0.366 and 0.411, respectively; P<0.01), but there was no relationship between centrum ovale PVS score and arteriosclerosis score (Figure 3B,C,D,E,F,G). The basal ganglia PVS score at one side was positively related to the scores of contralateral intracranial arteriosclerosis and contralateral extracranial arteriosclerosis (r=0.178 and 0.276, respectively; P<0.01). There was no correlation between centrum ovale PVS score at one side and score of contralateral intracranial arteriosclerosis or contralateral extracranial arteriosclerosis (Figure 3H,I,J,K).
CBF and its relationship with PVS score
In the CP group and CP + IA group, the CBF in the basal ganglia and centrum ovale was significantly lower than in the control group [basal ganglia: 34.01±10.96 vs. 38.48±7.42 (CP vs. control), 30.6±8.38 vs. 38.48±7.42 (CP + IA vs. control), P<0.05; centrum ovale: 27.85±9.29 vs. 33.69±6.92 (CP vs. control), 25.16±7.09 vs. 33.69±6.92 (CP + IA vs. control); P<0.05]. The CBF in the basal ganglia and centrum ovale was comparable between IA group and control group. The CBF in the basal ganglia and centrum ovale of patients in the CP group was also similar to that in the IA group and CP + IA group. The CBF in the basal ganglia and centrum ovale of patients in the CP + IA group was significantly lower than in the IA group (basal ganglia: 30.60±8.38 vs. 36.52±8.57, P<0.05; centrum ovale: 25.16±7.09 vs. 31.09±7.67, P<0.05) (Figure 4A).
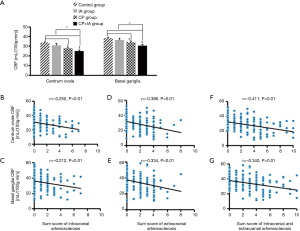
The scores of intracranial arteriosclerosis, extracranial arteriosclerosis, and intracranial and extracranial arteriosclerosis were negatively related to the CBF in the basal ganglia (r=−0.212, −0.334 and −0.340, respectively; P<0.01) and the centrum ovale (r=−0.250, −0.386 and −0.411, respectively; P<0.01) (Figure 4B,C,D,E,F,G).
Correlation between CBF and PVS
In the basal ganglia and centrum ovale, the CBF was negatively related to the corresponding PVS score (r=−0.375 and −0.220, respectively; P<0.01) (Figure 5A,B). The sum score of intracranial and extracranial atherosclerosis and age were used as control variables, and partial correlation analysis showed the CBF was negatively related to PVS score in the basal ganglia and centrum ovale (r=−0.294 and −0.226, respectively; P<0.01) (Figure 5C,D).
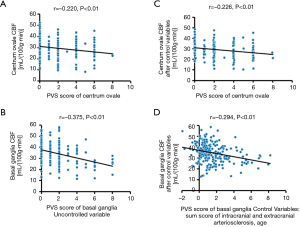
Discussion
Studies have suggested that the pathogenesis of PVS is related to age (7). Until now, the exact pathogenesis of PVS is still unknown. Our results showed age was related to the incidence of PVS, which might be explained as the deterioration of arteriosclerosis over age. The present study showed no significant difference in the hypertension between patients with or without PVS, which was inconsistent with previous findings (7). Considering that more patients in the PVS group had only centrum ovale PVS, and the centrum ovale PVS may be more related to the nontraditional vascular risk factors (such as β amyloid protein deposition) but not hypertension, because the centrum ovale is located at the farthest point of the branch of cerebral vessels, the collateral circulation is abundant and the centrum ovale is less affected by the hypertension induced vascular wall damage. Our study showed that PVS patients had a higher prevalence of coronary heart disease, intracranial artery stenosis and carotid plaque, and there were no significant differences in the use of antiplatelet treatment and statins treatment between the two groups, suggesting that PVS may be associated with atherosclerosis, and statins and antiplatelet treatments may prevent the occurrence of PVS while improving atherosclerosis.
Few studies about the etiology of PVS have focused on the atherosclerosis, and little is known about the relationship between carotid arteriosclerosis and PVS. Thus, the correlation between extracranial carotid atherosclerosis and intracranial PVS is still poorly understood. In the study of Gutierrez et al., 706 subjects from Manhattan, North America were investigated and results indicated the PVS was closely related to carotid plaque (9). In the present study, ultrasonography was done to assess the carotid arteriosclerosis and results showed the prevalence of PVS in patients with carotid plaque increased significantly. Furthermore, the carotid plaque was scored for the assessment of arteriosclerotic severity. Results showed the severity of PVS was positively related to the score of arteriosclerosis. In recent years, the correlation between intracranial large vessel atherosclerosis and intracranial PVS is still controversial. Pathological studies reveal a close relationship between intracranial microarteriosclerosis and PVS (14,15). Our study showed the incidence of PVS significantly increased in patients with intracranial arteriosclerosis, in which CTA was employed to assess the extent of intracranial arteriosclerosis. Further analysis showed the intracranial arteriosclerosis was positively related to the severity of PVS. Of interest, the incidence of PVS was higher in the patients with intra-/extracranial arteriosclerosis, and the relationship between sum score of intra-/extracranial arteriosclerosis and PVS score was stronger. These results indicate that intracranial arteriosclerosis or extracranial atherosclerosis is positively related to the basal ganglia PVS: the greater the extent of intracranial and extracranial arteriosclerosis, the higher the incidence of PVS is. This suggests that the pathogenesis of PVS is associated with the load of overall arteriosclerosis in the brain. Correlation analysis further supported this hypothesis: the severity of PVS increased with the elevation of arteriosclerosis score. This implies that the higher the score of intra-/extracranial arteriosclerosis, the higher the incidence of PVS is and the more severe the PVS is.
There is still controversy on the CBF in stroke-free patients with carotid plaque. There is evidence showing that the mild to severe carotid stenosis due to carotid plaque may cause CBF reduction (16). However, the CBF fails to reduce significantly in patients with carotid plaque, and only ≥90% carotid stenosis can cause significant CBF reduction (17). Our results showed that the CBF of patients with carotid arteriosclerosis due to carotid plaque reduced markedly as compared to the controls, and the score of extracranial arteriosclerosis was negatively related to CBF. Few studies have been conducted to investigate the intracranial arteriosclerosis and CBF in patients without stroke. Cheng et al. found intracranial arteriosclerosis had no relationship with reduced CBF (18). In the study of Ran et al., results showed intracranial arteriosclerosis was associated with reduced CBF (19). Our results indicated that the CBF was similar between patients with intracranial arteriosclerosis and controls. We speculate that intracranial arteriosclerosis has little influence on the CBF and hemodynamics due to abundant intracranial arteries and Willis cycle in the brain. Further analysis showed the CBF in patients with intra-/extracranial arteriosclerosis was significantly lower than in those with intracranial arteriosclerosis alone, but the CBF was comparable between patients with carotid arteriosclerosis and those with intracranial arteriosclerosis. This indicates that intra-/extracranial arteriosclerosis has significant influence on the CBF. Correlation analysis indicated that the relationship between intra-/extracranial arteriosclerosis score and CBF reduction was stronger. This implies that the CBF reduces with the elevation of overall load of arteriosclerosis in the brain if intra-/extracranial arteriosclerosis score is employed for the assessment of overall cerebral arteriosclerosis.
Our results showed the higher the overall load of cerebral arteriosclerosis, the higher the incidence of PVS was and the lower the CBF was. PVS score was negatively related to CBF. This indicates the reduced CBF in focal cerebral tissues due to intra-/extracranial arteriosclerosis may be related to the elevated incidence of PVS, and the higher the load of intra-/extracranial arteriosclerosis, the more severe the PVS is. Of interest, our results showed the PVS in patients with intracranial arteriosclerosis, carotid plaque or intra-/extracranial arteriosclerosis was mainly found in the basal ganglia. However, Tao et al. (20) found basal ganglia PVS was negatively related to the intracranial arteriosclerosis assessed by intracranial carotid calcification, which was inconsistent with the results reported by Del Brutto et al. (21). This discrepancy might be ascribed to small number of patients with PVS at score 3–4 and different distributions of PVS scores. Our results were consistent with those reported by Del Brutto et al., but contrary to those reported by Tao et al. This might be ascribed to the differences in the methodology, risk factors and sample size. We speculate that the elevation of incidence and severity of basal ganglia PVS with the increase in arteriosclerosis score might be related to the susceptibility of basal ganglia to hemodynamic change because the basal ganglia is mainly supplied by the lenticulostriate artery being vertically branched from the middle cerebral artery and the hemodynamic change may cause damage to the arteriole wall, resulting in arteriosclerosis and lipid hyaline degeneration (22). In addition, pathological studies have confirmed that there are small arteries and veins in the enlarged PVS, and the enlarged PVS can reduce the volume of surrounding brain parenchyma (22), which decreases the number and density of local capillaries, thus leading to the decrease of regional CBF. Studies have shown that the order of blood vessels that affect the local brain perfusion is aorta, arterioles, capillaries and veins (23). In our study, the PVS diameter was less than 3 mm, and the whole CBF in the centrum ovale and basal ganglia was investigated in the study. The contribution of intracranial and extracranial arteriosclerosis to the CBF reduction was more than that of parenchymal volume reduction secondary to PVS. The low perfusion or ischemia in this area may cause secondary inflammatory reaction and cell metabolism disorder (24), which may damage the vascular endothelium, increase blood-brain barrier permeability, induce the atrophy of small vessels, and enlarge the PVS. Our results showed the incidence of centrum ovale PVS was comparable between patients with intracranial arteriosclerosis, carotid plaque or intra-/extracranial arteriosclerosis and controls. This indicates that the pathogenesis of centrum ovale PVS has involvement of factors other than arteriosclerosis. The PVS is susceptible to intracranial and extracranial atherosclerosis because (I) atherosclerosis causes inflammatory reaction and intracranial vascular endothelial dysfunction, which increases the permeability of blood-brain barrier and therefore elevates the leakage of intravascular fluid, resulting in PVS; (II) arteriosclerosis decreases the CBF, which increases the expression of intracranial matrix metalloproteinase-2 (MMP-2) (22), leading to the acetylation of nitric oxide synthase (25) and redistribution of AQP4 in the cerebral small vessels (26). These change the cerebral small vascular permeability and cause the imbalance of energy metabolism in vascular endothelial cells, increasing the incidence of PVS.
Currently, the mechanism of PVS dilation is still poorly understood, and it was primarily explored in the present study. The intergroup comparison might be affected by the age and other potential factors, but our results were still clinically important considering the close relationship between intra-/extracranial arteriosclerosis and PVS score. There are still some limitations in the present study. (I) The complex patients with a wide range of mixed stenosis were not excluded from this study. (II) The severity of intracranial vascular stenosis was not refined, and the PVS at stenosis and non-stenosis sides were not independently investigated. (III) PVS counting and grading according to the location is easy to cause the “ceiling effect”. (IV) The PVS was basically <3 mm in diameter in our study. (V) The PVS was macroscopically classified. In future research, it is better to automatically analyze the PVS lesions with the aid of a computer, which will make our results more convincing. (VI) This was a cross-sectional study and the relationship between intra-/extracranial arteriosclerosis and PVS should be further confirmed in more studies with large sample size.
Conclusions
In summary, the incidence of PVS in patients with intra-/extracranial arteriosclerosis is higher than in those without arteriosclerosis. The extent of intracranial and extracranial atherosclerosis is related to PVS, especially the basal ganglia PVS. Decreased CBF may be associated with the pathogenesis of PVS.
Acknowledgments
Funding: This work was partially supported by the National Key R&D Program of China (No. 2018YFC1314700); the National Science Foundation of China (No. 81671307); the Priority of Shanghai Key Discipline of Medicine (2017ZZ02020); and the Fundamental Research Funds for the Central Universities (No. 22120180079).
Footnote
Conflicts of Interest: The other authors have no conflicts of interest to declare.
Ethical Statement: The authors are accountable for all aspects of the work in ensuring that questions related to the accuracy or integrity of any part of the work are appropriately investigated and resolved. This study was approved by the Ethics Committee of Tongji Hospital [No. (Tong)-LUNSHEN-KYSB-2016-(99)] and all participants provided informed consent.
Open Access Statement: This is an Open Access article distributed in accordance with the Creative Commons Attribution-NonCommercial-NoDerivs 4.0 International License (CC BY-NC-ND 4.0), which permits the non-commercial replication and distribution of the article with the strict proviso that no changes or edits are made and the original work is properly cited (including links to both the formal publication through the relevant DOI and the license). See: https://creativecommons.org/licenses/by-nc-nd/4.0/.
References
- Wardlaw JM, Smith EE, Biessels GJ, et al. Neuroimaging standards for research into small vessel disease and its contribution to ageing and neurodegeneration. Lancet Neurol 2013;12:822-38. [Crossref] [PubMed]
- Brinker T, Stopa E, Morrison J, et al. A new look at cerebrospinal fluid circulation. Fluids Barriers CNS 2014;11:10. [Crossref] [PubMed]
- Arba F, Quinn TJ, Hankey GJ, et al. Enlarged perivascular spaces and cognitive impairment after stroke and transient ischemic attack. Int J Stroke 2018;13:47-56. [Crossref] [PubMed]
- Shams S, Martola J, Charidimou A, et al. Topography and Determinants of Magnetic Resonance Imaging (MRI)-Visible Perivascular Spaces in a Large Memory Clinic Cohort. J Am Heart Assoc 2017. [Crossref] [PubMed]
- Wang X, Chappell FM, Valdes Hernandez M, et al. Endothelial Function, Inflammation, Thrombosis, and Basal Ganglia Perivascular Spaces in Patients with Stroke. J Stroke Cerebrovasc Dis 2016;25:2925-31. [Crossref] [PubMed]
- Duperron MG, Tzourio C, Sargurupremraj M, et al. Burden of Dilated Perivascular Spaces, an Emerging Marker of Cerebral Small Vessel Disease, Is Highly Heritable. Stroke 2018;49:282-7. [Crossref] [PubMed]
- Potter GM, Doubal FN, Jackson CA, et al. Enlarged perivascular spaces and cerebral small vessel disease. Int J Stroke 2015;10:376-81. [Crossref] [PubMed]
- van Swieten JC, van den Hout JH, van Ketel BA, et al. Periventricular lesions in the white matter on magnetic resonance imaging in the elderly. A morphometric correlation with arteriolosclerosis and dilated perivascular spaces. Brain 1991;114:761-74. [Crossref] [PubMed]
- Gutierrez J, Rundek T, Ekind MS, et al. Perivascular spaces are associated with atherosclerosis: an insight from the Northern Manhattan Study. AJNR Am J Neuroradiol 2013;34:1711-6. [Crossref] [PubMed]
- Touboul PJ, Hennerici MG, Meairs S, et al. Mannheim intima-media thickness consensus. Cerebrovasc Dis 2004;18:346-9. [Crossref] [PubMed]
- Sutton-Tyrrell K, Alcorn HG, Wolfson SK Jr, et al. Predictors of carotid stenosis in older adults with and without isolated systolic hypertension. Stroke 1993;24:355-61. [Crossref] [PubMed]
- Samuels OB, Joseph GJ, Lynn MJ, et al. A standardized method for measuring intracranial arterial stenosis. AJNR Am J Neuroradiol 2000;21:643-6. [PubMed]
- Doubal FN, MacLullich AM, Ferguson KJ, et al. Enlarged perivascular spaces on MRI are a feature of cerebral small vessel disease. Stroke 2010;41:450-4. [Crossref] [PubMed]
- Weller RO, Djuanda E, Yow HY, et al. Lymphatic drainage of the brain and the pathophysiology of neurological disease. Acta Neuropathol 2009;117:1-14. [Crossref] [PubMed]
- Deramecourt V, Slade JY, Oakley AE, et al. Staging and natural history of cerebrovascular pathology in dementia. Neurology 2012;78:1043-50. [Crossref] [PubMed]
- Hashimoto N, Hama S, Yamane K, et al. Carotid arterial intraplaque hemorrhage and calcification influences cerebral hemodynamics. Neurosurg Rev 2013;36:421-7. [Crossref] [PubMed]
- Huang L, Cao W, Ge L, et al. Intracranial Blood Flow Changes in Patients with High-Grade Severe Carotid Artery Stenosis After Stenting. World Neurosurg 2017;104:863-8. [Crossref] [PubMed]
- Cheng XQ, Tian JM, Zuo CJ, et al. Hemodynamic alterations in unilateral chronic middle cerebral artery stenosis patients and the effect of percutaneous transluminal angioplasty and stenting: a perfusion-computed tomography study. Acta Radiol 2015;56:754-60. [Crossref] [PubMed]
- Ran YC, Zhu M, Zhang Y, et al. Perfusion-weighted magnetic resonance imaging in the assessment of haemodynamics following stent angioplasty in patients with symptomatic middle cerebral artery plaque stenosis at the M1 segment. Exp Ther Med 2017;14:1899-904. [Crossref] [PubMed]
- Tao XX, Li GF, Wu YL, et al. Relationship between intracranial internal carotid artery calcification and enlarged cerebral perivascular space. Neuroradiology 2017;59:577-86. [Crossref] [PubMed]
- Del Brutto OH, Mera RM. Enlarged perivascular spaces in the basal ganglia are independently associated with intracranial atherosclerosis in the elderly. Atherosclerosis 2017;267:34-8. [Crossref] [PubMed]
- Ihara M, Yamamoto Y. Emerging Evidence for Pathogenesis of Sporadic Cerebral Small Vessel Disease. Stroke 2016;47:554-60. [Crossref] [PubMed]
- Zhu S, Li Y, Lu H, et al. Imaging the early cerebral blood flow changes in rat middle cerebral artery occlusion stroke model. Conf Proc IEEE Eng Med Biol Soc 2012;2012:2655-8. [PubMed]
- Kitamura A, Saito S, Maki T, et al. Gradual cerebral hypoperfusion in spontaneously hypertensive rats induces slowly evolving white matter abnormalities and impairs working memory. J Cereb Blood Flow Metab 2016;36:1592-602. [Crossref] [PubMed]
- Hattori Y, Okamoto Y, Maki T, et al. Silent information regulator 2 homolog 1 counters cerebral hypoperfusion injury by deacetylating endothelial nitric oxide synthase. Stroke 2014;45:3403-11. [Crossref] [PubMed]
- Mestre H, Kostrikov S, Mehta RI, et al. Perivascular spaces, glymphatic dysfunction, and small vessel disease. Clin Sci (Lond) 2017;131:2257-74. [Crossref] [PubMed]