Transcriptomic changes and potential regulatory mechanism of intrauterine human chorionic gonadotropin co-cultured with peripheral blood mononuclear cells infusion in mice with embryonic implantation dysfunction
Introduction
In the past few decades, in vitro fertilization and embryos transfer (IVF-ET) treatment have been widely used in infertile couples around the world. With the continuous advancement of assisted reproduction technology (ART), patients have access to a greater number of good-quality embryos. However, there is almost only 30% probability on the implantation of each embryo (1,2), even 10% of IVF patients suffer from repeated implantation failure (RIF) (3). RIF is a clinical quandary in reproductive medicine, which refers to a situation when implantation has repeated failed after transferring morphologically normal embryos to anatomically normal uterus (4).
The embryo implantation is a complex and delicate process, which requires a synchronization and coordinating cross-talk between an embryo with good developmental potential and a receptive endometrium (4). All of these events occur at a temporal period that is called the “window of implantation (WOI)” (5). It was reported that impaired endometrial receptivity was responsible for approximately two-thirds of the implantation failure (6), which made the unreceptive status of endometrium the most significant barrier to the establishment of pregnancy. Previous studies showed that a variety of factors participated in endometrium decidualization, including ovarian hormones, cytokines, growth factors, and transcription factors secreted by embryos and endometrium (5), of which chorionic gonadotropin (CG), secreted by developing embryos, was one of the most important signals profoundly modulating immunological tolerance and angiogenesis at maternal-fetal interface (7). Some studies also demonstrated that human chorionic gonadotropin (hCG) had an effect of promoting endometrial receptivity in women (8) and other primates (9).
Moreover, there is increasing evidence that large populations of decidual immune cells play a pivotal role on implantation and throughout the pregnancy (10,11), including the emergence of a receptive endometrium (12). During trophoblast cells invasion, blastocyst breaks through endometrial tissues and activates Th1 and Th17, while Th2 and Tregs are also needed to maintain pregnancy and regulate embryo invasion. At the implantation site, the endometrium has a sufficient blood supply with a large number of peripheral blood mononuclear cells (PBMCs), which are mainly composed of T lymphocytes, B lymphocytes, and monocytes. Preliminary studies showed that PBMCs could induce functional changes of endometrium and promote BeWo cells attachment (13), and hCG might be able to enhance such effects (14). Yoshioka et al. (15) developed an approach, who used hCG to culture with PBMCs obtained from RIF patients for 48 h, and administrated cultured PBMCs together with freshly isolated PBMCs into the uterus, which demonstrated a significant improvement on the clinical pregnancy rate, the implantation rate and the live birth rate in PBMCs-treated patients. Since then, intrauterine administration of autologous PBMCs has become one of the therapies for RIF patients. A recent meta-analysis indicated that PBMCs infusion increased the probability of clinical pregnancy and live birth irrespective of embryos stage and ET cycle types (16), though chemical and immunological indices were lacked to clarify the mechanisms of such benefits.
Several uterine receptivity biochemical markers have been proposed, however, none of them has been transformed into clinical practice successfully. Given the complexity of endometrial receptivity, the transcriptomic study as an alternative approach allowed scholars to simultaneously study all molecules involved in the implantation process. Kao et al. pioneered the microarray technology to describe the genetic changes of secretory-phase endometrium (17). From then on, many scientists utilized this test to explore the regulation of endometrial receptivity (18). Díaz-Gimeno et al. developed endometrial receptivity array (ERA) test (19), including 238 differentially expressed genes (DEGs) of WOI. As a molecular predictive model, ERA could objectively diagnose the status of endometrium and create possibilities for individualized ET (20). At present, high-throughput RNA sequencing (RNA-Seq) technology and massive data analysis could provide a more comprehensive molecular profiling for endometrium (21) of specific populations, such as RIF (22) and recurrent miscarriage (23) during implantation.
In our previous studies, we detected that hCG could induce interleukin-1β (IL-1β) and TNF-α in PBMCs, and its secretion peaked after a 24-hour culture (24). At the same time, we observed a promotion of leukemia inhibitory factor (LIF) and VEGF in endometrium and a higher pregnancy rate in embryo implantation dysfunction (EID) mouse model after a hCG co-cultured with PBMCs infusion (25). We also demonstrated administration of hCG co-cultured with PBMCs for 24 hours could significantly improve the adverse pregnancy outcome of RIF patients, especially for women who had undergone four or more implantation failures (26). However, the mechanisms of hCG co-cultured with PBMCs infusion in promoting a receptive endometrium remain unclear. In the current study, we performed a single intrauterine administration of hCG, PBMCs or hCG co-cultured PBMCs to mice with EID, and used RNA-Seq to analyze the potential role of such treatment during the putative implantation window. We showed that there were potential clinical benefits for intrauterine administration of hCG co-cultured with PBMCs prior to embryos implantation in mice with EID.
Methods
Animals
Adult male and female ICR mice of specific pathogen free (SPF) grade were obtained from Beijing Vital River Laboratory Animal Technology Co., Ltd. (Beijing, China). They were housed in Animal Laboratory Center of Renmin Hospital of Wuhan University (No. SYXK 2015-0027, Wuhan, China), under environmentally controlled conditions (12 hours light and 12 hours darkness, 22–24 °C, 75–85% relative humidity) with standard food and water ad libitum. Eight to ten weeks old mice were used in our study, females weighed about 26–28 g and males weighed about 29–32 g. All female mice were caged with male mice (ratio 2:1) at 7:00 pm and vaginal plugs were checked at 7:00 am on the next morning. Presence of vaginal plugs was considered as the evidence of mating, and the day was defined as the pregnant day 0.5 (PD 0.5). Intrauterine perfusion was performed through bilateral uterine horn at 9:00 am in PD 1.5 and EID mouse model was induced by mifepristone at 9:00 am in PD 3.5. At 9:00 pm in PD4, all female mice were sacrificed and the endometrium were collected for further experiments. All animal procedures included in this study conformed to the National Institutes of Health guidelines on the ethical use of animals (NIH Publication, revised 2011) and the Guideline for the Care and Use of Laboratory Animals of the Chinese Animal Welfare Committee. All experiments were approved by the Animal Use Committee of Renmin Hospital of Wuhan University (Wuhan, China).
Preparation of PBMCs
Consistent with our previous studies (25,27), we used Ficoll-PaqueTM PLUS (Tianjin Haoyang Biological Manufacture Co., Ltd., Tianjin, China) to centrifugate PBMCs from peripheral blood of unpregnant female mice. After centrifugation, PBMCs were collected from the interface layer and washed twice with PBS (Gibco®, Grand Island, NY, USA). Thereafter, PBMCs were suspended in RPMI1640 (Gibco®, Grand Island, NY, USA) supplemented with 10% FBS (Gibco®, Grand Island, NY, USA), with or without 5 IU/mL hCG (Zhuhai Livzon Diagnostics Inc., Zhuhai, China). And then incubated PBMCs at 37 °C under 5% CO2 and high humidity for 24 hours.
EID mouse model establishment
The mice were mated as described above. Then we randomly divided pregnant mice into normal pregnancy (NP) group, EID group, EID with hCG group, EID with PBMCs group and EID with hCG co-cultured with PBMCs group. EID mice were made as our previous study as follows (25): 0.1 mL mifepristone (dissolved in propanediol at a concentration of 0.08 mg/mL, Hubei Gedian Renfu pharmaceutical company, Hubei, China) was injected into each mouse at PD 3.5 subcutaneously.
Intrauterine administration
After animals were anesthetized by isoflurane (RWD Life Science, Shenzhen, China), we surgically administrated 2.5 µL therapeutic fluids into the uterus through cranial part of each uterine horn using a micro-syringe. We administrated blank culture medium to the NP and EID mice, culture medium with 5 IU/mL hCG, PBMCs and PBMCs with 5 IU/mL hCG to the EID. The overall operation time of a mouse was no more than 20 minutes, and the unilateral uterine horn administration time was about 5 seconds.
Tissue collection and tissue processing
All mice were sacrificed at PD4, which represented the “WOI” of mice, and endometrial samples were obtained. Endometrial tissues were snap-frozen in liquid nitrogen and then stored at –70 °C till further experiments.
RNA extraction and purification
Total RNA was extracted from endometrial tissue using RNAiso Plus Total RNA extraction reagent (Cat#9109, TAKARA Biomedical Technology Co., Ltd., Tokyo, Japan) according to the manufacturer’s instructions. Use Agilent Bioanalyzer 2100 (Agilent Technologies, Santa Clara, CA, US) checked the RNA integrity. Qualified total RNA was then purified by RNA Clean XP Kit (Cat.A63987, Beckman Coulter Inc., Kraemer Boulevard Brea, CA, USA) and RNase-Free DNase Set (Cat#79254, QIAGEN GmbH, Germany).
RNA-Seq procedure
We used Ribo-Zero rRNA removal beads (Mouse, Illumina, USA) to remove ribosomal RNA from total RNA and fragmented the mRNA into small pieces using divalent cations under 94 °C for 8 min. The cleaved RNA fragments were copied into first-strand cDNA using reverse transcriptase and random primers, and seconded-strand cDNA was synthesized using DNA Polymerase I and RNase H. Then cDNA fragments went through an end repair process, the addition of a single ‘A’ base and ligation of the adapters. The products were then purified and enriched with polymerase chain reaction (PCR) to create a final cDNA library. To confirm the insert size and calculate the mole concentration, purified libraries were quantified by Qubit® 2.0 Fluorometer (Life Technologies, Carlsbad, CA, USA) and validated by Agilent 2100 bioanalyzer (Agilent Technologies, USA). Cluster was generated by cBot with the library diluted to 10 pM, which were then sequenced on the Illumina HiSeq 2500 (Illumina, USA).
Before reads mapping, clean reads were achieved from raw reads by filtering out rRNA reads, sequencing adapters, short fragment reads and other low-quality reads. Those high-quality reads passed the Illumina quality filters were kept for the afterwards sequencing analysis.
Transcriptome data analysis and significant genes overlapping
Clean reads were aligned to the genome GRCm38.p4 (mm10) by Hisat2 v:2.0.4. Transcript levels were estimated using fragments per kilobases per million reads (FPKM), the DEGs were identified by edge R algorithms. The up- or down-regulated genes were filtered from the RNA-Seq data with P values <0.05.
In order to identify the potential functional molecules of our study, we performed genes overlapping over significantly DEGs between EID group and NP group, EID with hCG group, EID with PBMCs group and EID with hCG co-cultured with PBMCs group, EID with hCG co-cultured with PBMCs group and EID with hCG group and EID with hCG co-cultured with PBMCs-treated group. We first used |log2(fold change)| ≥1 and P<0.05 as the screening criteria, and then summarized genes which have been included in ERA test (19). After that, we expanded the range of our screening to the whole genome, and used |log2(fold change)| ≥2 and P<0.05 as the cut-off. Under two standards above, genes with high degree of coincidence were used for further verification.
Quantitative real-time polymerase chain reaction (qRT-PCR)
Total RNA was extracted from another 15 endometrium samples (3 samples per group) using TRIzol extraction reagents (Invitrogen, Grand Island, NY, USA), according to the methods of manufacturer’s instructions. RNA purity and concentration were photometrically tested. RNA was reverse-transcribed into cDNA using Reverse Aid First Strand cDNA Synthesis Kit (Thermo, Grand Island, NY, USA). RT-PCR was performed using Trans-Start Tip Green qPCR Supermix (Transgen Biotech, Beijing, China) with ABI 7500 RT-PCR (Bio-Rad, CA, USA). Specific primer sequences are listed in (Table 1). The gene expression levels were calculated relative to GAPDH using the 2–ΔΔCq method.
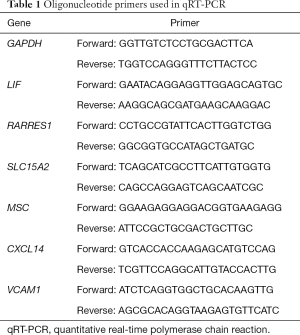
Full table
Detection of protein in cell supernatant by enzyme-linked immunosorbent assay (ELISA)
Glucocorticoid receptor (GR)-β ELISA kit was obtained from R&D company, and the procedure was practiced according instruction. Measure the absorbance at 450 nm, using a microtiter plate spectrophotometer. Perform an end-point measurement after 1 h. Calculate the titer of the sera. The titer can be defined as the dilution of cell culture supernatant giving an optical density (OD) of 0.2 above the background of the ELISA after a 1-h reaction. Before calculation of results, create a standard curve by plotting the mean absorbance for each standard concentration on the ordinate against the GR-β concentration on the abscissa.
Statistical analysis
Data were presented as mean ± standard error of mean (SEM). Unpaired t-test for two comparisons, and one-way analysis of variance (ANOVA) with multiple comparisons test for one data set were used to evaluate the statistical significances of the results and statistical analyses were undertaken by SPSS 22.0 (SPSS, Chicago, IL, USA). Significance was accepted at P<0.05.
Results
RNA-Seq analysis
In this experiment, a total of 21 endometrial samples from five groups were detected by RNA-Seq. At least three biological replicates for each set. We generated five libraries and sequenced them to a depth of greater than 40 million high-quality mappable clean reads, and more than 90% of the sequence bases could be aligned to the genome (Figure 1, Table 2). In summary, the sequence distribution of each sample was balanced and with evenness genome mapping. Our sequencing results were of good quality and could be used for further analysis.
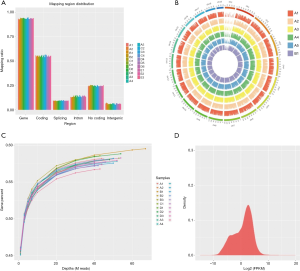
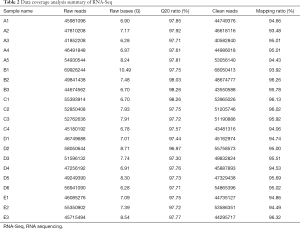
Full table
DEGs analysis
The number of DEGs were listed (P<0.05) (Table 3). Using fold change ≥4 and P<0.05 as the screening criteria, we overlapped genes which have been included in ERA test, and 15 genes were selected for a coincidence of greater than twice. We also used fold change ≥2 and P<0.05 as the filter to extensively overlap the DEGs of genome and 160 genes were selected for a coincidence of greater than three times (Figure 2). Then a literature research was conducted about the above 172 genes, of which 24 genes had been reported to be involved in embryo implantation, immune response and other reproductive processes. We also verified these genes by qRT-PCR for another 15 samples, the expression of retinoic acid receptor responder 1 (RARRES1), solute carrier family 15 member 2 (SLC15A2), musculin (MSC), chemokine (C-X-C motif) ligand 14 (CXCL14), and vascular cell adhesion molecule 1 (VCAM1) was different significantly (Figures 3,4). Though LIF failed to meet part of our requirements mentioned above, we specially included LIF as another candidate marker in our study, considering its representative role in endometrial receptivity assessment.
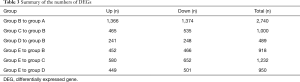
Full table
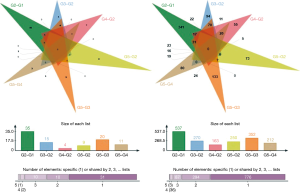
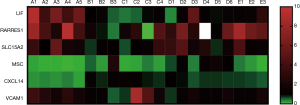
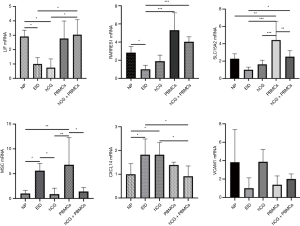
Intrauterine hCG co-cultured with PBMCs administration induced the expression of endometrial receptivity marker LIF
In our previous data, Intrauterine infusion of hCG co-cultured with PBMCs to EID mouse could induce an accumulation of LIF in endometrium (25). We observed a significant decrease of LIF expression in EID mice [P<0.001, log2(fold change) =–2.53], and a rebound after hCG co-cultured with PBMCs infusion [P<0.05, log2(fold change) =0.78]. In addition, we also discovered an elevation in PBMCs-treated group, though there was no statistical difference (P>0.05) (Figure 4).
Intrauterine hCG co-cultured with PBMCs administration induced expression of CXCL14
CXCL14 was highly chemotactic for monocytes and was elevated in the mid-secretory phase endometrium. In our study, we observed an increase of CXCL14 expression in EID mice [P<0.0001, log2(fold change) =1.87], and an infusion of PBMCs slightly reduced of CXCL14 (P>0.05), but an infusion of hCG co-cultured with PBMCs could reduce its expression significantly [P<0.01, log2(fold change) =–0.64] (Figure 4).
Intrauterine administration of PBMCs induced RARRES1 expression
According to ERA test (19), RARRES1 expression was increased in endometrium at WOI, while another transcriptome analysis demonstrated that RARRES1 was significantly decreased in RIF patients (28). Our results showed that RARRES1 was decreased in EID mice [P<0.001, log2(fold change) =–2.48], which was similar to the previous results. Additionally, RARRES1 expressions were all enhanced in EID with PBMCs group and hCG co-cultured with PBMCs group [P<0.01, log2(fold change) =2.35; P<0.001, log2(fold change) =–2.70], which occurs during the mid-luteal phase generally (Figure 4).
PBMCs intrauterine infusion induced SLC15A2 expression in EID mice
SLC15A2, reported to be involved in immune response, was reduced in the endometrium of EID mice [P<0.001, log2(fold change) =–1.46]. Simultaneously, we discovered that PBMCs and hCG co-cultured with PBMCs administration were able to increase SLC15A2 expression [P<0.01, log2(fold change) =1.29; P<0.01, log2(fold change) =1.23]. Though we failed to observe a significant down-regulation of SLC15A2 in EID mice by PCR analysis (P<0.05), there was still a high resemblance to RNA-Seq data (Figure 4).
Intrauterine hCG infusion significantly induced MSC expression in endometrium
We also conducted an analysis over the expression of MSC, which was down expressed infertile mice, and could regulate Tregs differentiation (29). In our current research, we detected MSC expression was significantly elevated [P<0.0001, log2(fold change) =3.19] in EID mice. hCG co-cultured with PBMCs administration exhibited an obvious inhibition in such elevation [P<0.001, log2(fold change) =–2.19; P<0.01, log2(fold change) =–2.07] (Figure 4).
Intrauterine hCG administration induced the VCAM1 expression in endometrium
In our previous data, we demonstrated an increase of VEGF by hCG combined PBMCs administration (21). VCAM1, as another regulator to affect vascular permeability, might be activated on the embryo implantation. RNA-Seq showed that VCAM1 expression was significantly decreased in endometrium of EID mice [P<0.001, log2(fold change) =–1.33], while hCG treatment significantly increased VCAM1 expression [P<0.01, log2(fold change) =1.97]. However, we failed to find a difference in hCG co-cultured PBMCs group (P>0.05). qRT-PCR results showed a difference within five groups (P<0.05), and the overall trend was similar, but no significant difference was found in multiple comparisons (Figure 4).
GR-β expression in PBMC
The GR, including GR-α and GR-β, is a key receptor involved in inflammatory responses. It was found that GR at a low level, could affect the biological effects of endogenous glucocorticoids (GCs), and decreased cellular responsiveness to GCs, so that patients were in the immune turbulence owing to weaker of immunosuppressive effect (30). PBMCs from normal pregnant mice and EID mice were isolated and incubated for 24 hours respectively, and the expression of GR-β in cell supernatant was detected by ELISA. The expression of GR-β in EID mice was decreased significantly (P<0.01) (Figure 5).
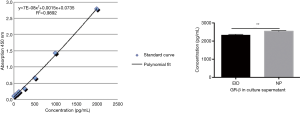
The correlation among LIF, SLC15A2, RARRES1, VCAM1, CXCL14, MSC and GR-β
Considering that GR-β is a transcriptional factor related to inflammation, we used PROMO program to predict the binding sites on the promoter regions of these six genes. We found that there were all GR-β binding sites in the upstream regions of these six genes (Figure 6). Furthermore, LIF, SLC15A2, RARRES1, VCAM1 were positively regulated and CXCL14, MSC were negatively regulated by GR-β. After isolation of normal unpregnant PBMCs, PBMCs were co-cultured with GR-β, and intrauterine perfusion was performed in EID mice. It was found that the expression of LIF, SLC15A2, RARRES1, VCAM1 in the endometrium of EID mice increased, while the expression of CXCL14 and MSC decreased, the effect of PBMCs co-cultured with GR-β was more obvious than that of PBMCs by RT-PCR (P<0.05) (Figure 7).
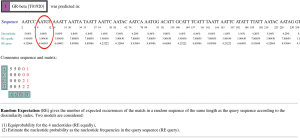
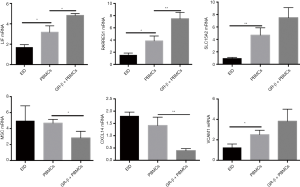
Discussion
Large amounts of immune cells and immune-related factors at implantation site are important components of maternal-fetal interface (31), which contribute to the immune tolerance while induce a moderate inflammatory response during embryo implantation. Many studies indicated that abnormal immune environment of endometrium and peripheral blood could lead to EID. Some scholars used a specific combination of immunological biomarkers to determine whether uterine immunological microenvironment played a role in implantation, and they discovered more than 80% of patients with previous implantation failures exhibited an immune imbalance in endometrium, which might be the root for their poor endometrial receptivity (32). Lymphocyte therapy treatment (LIT) (33), intravenous immunoglobulin (34) and other immune interventions could effectively improve the pregnancy outcome of RIF patients. Although detailed indications and further exploration was needed to verify clinical effectiveness, our previous data showed intrauterine infusion of hCG co-cultured with PBMCs before ET could achieve better clinical pregnancy outcomes for some RIF patients (26). We also discovered that hCG co-cultured with PBMCs intrauterine administration in advance could effectively improve the number of blastocysts implanting in EID mice (25). To explore the potential mechanisms responsible for such improvements in mice and RIF patients, we depicted the transcriptome profiles of endometrium in EID mice model after receiving a hCG, PBMCs and hCG co-cultured with PBMCs infusion by RNA-Seq, verified the most differential expressed genes by qRT-PCR, and discovered that LIF, RARRES1, SLC15A2, MSC, CXCL14, VCAM1 might be the important factors regulating embryos implantation.
LIF, as a member of IL-6 family, is a Th2-associated cytokine. LIF played a key role on endometrial decidualization and embryos implantation (35), which made it be a commonly molecular marker to evaluate endometrial receptivity (36). Early studies found that LIF knockout mice showed some defects during embryos implantation processes, while exogenous supplementation of LIF could restore their fertility (37). We demonstrated that hCG co-cultured with PBMCs could elevate LIF expression in endometrium, which suggested infusion treatment could be used as a supplement to patients with implantation failures.
CXCL14 reached peak at embryos implantation site during WOI (38), and its knockout in mice could lead to infertility (39). It is showed that CXCL14 might be regulated by progesterone and exerted a chemo-attractive effect on natural killer (NK) cells for the accumulation in epithelial glands (40). In our study, we detected a significant increase in endometrial CXCL14 expression, which might be associated with local immune disorders caused by mifepristone, and this compensatory increase could be restored by intrauterine infusion of hCG co-cultured with PBMCs. At the same time, we also observed slight changes in LIF and CXCL14 in mice treated with PBMCs only, which might relate to the luteinizing hormone (LH)/hCG receptor in lymphocyte surface (41).
RARRES1, induced by retinoic acid, expressed in multiple tissues. Previous studies suggested that RARRES1 was involved in the apoptosis of tumor cells, inhibited the proliferation and invasion of tumor cells (42), and placenta formation might share some molecular processes of tumor (43). Studies indicated that RARRES1 was an important regulator of trophoblast cells (44), and its overexpression in trophoblast cells was highly correlated with the onset of preeclampsia (45). There were also reports that RARRES1 in ectopic endometrium of patients with endometriosis was higher than endometrium infertile women of childbearing age, prompting its potential role in endometrial differentiation (46). Our results showed the similarities in the human, where RARRES1 was down-regulated in EID endometrium, and PBMCs treatment recovered its down-regulation. So, we hypothesized that PBMCs might participate in endometrial differentiation and trophoblastic invasion through RARRES1. However, further exploration is needed, owing to the lack of relevant research on the role of RARRES1 during the implantation process.
SLC15A2 or PepT2 is a transmembrane transporter expressed in the cell membrane and the organelle membrane. In mice, SLC15A2 was highly expressed in mature immune cells and macrophages in bone marrow (47). By utilizing the proton gradient of the membrane, SLC15A2 completed the transport of dipeptides (MDP), tripeptides (Tri-DAP) and other peptidomimetic substances involved in innate immune responses, to induced the release of inflammatory factors, such as IL-6 and TNF-α. Although it was found that SLC15A2 declined in human during WOI, there was no other study to report its dysregulation in patients and mice with implantation difficulties. SLC15A2 was also involved in the onset of several immune diseases, such as inflammatory bowel disease and systemic lupus erythematosus. Our study observed a decreased expression of SLC15A2 in mice with implantation dysfunction, and intrauterine perfusion treatment of PBMCs might be useful to repair the injury. Thus, we suspected that mifepristone might inhibit moderate inflammatory responses of implantation, and the infusion of PBMCs might contribute to the recovery of immune micro-environment.
MSC is a member of the helix-loop-helix transcription factor family. Literature reports suggested that its down-regulation could induce the transformation of Tregs to Th2 cells through Foxp3 (29). In addition, studies have also detected the expression of MSC in Th17 and therefore participating in IL-2 secretion (48). Our results suggested that MSC was significantly increased when undertaken endometrial damage, and hCG infusion might effectively reverse such changes. Considering the positive effect of hCG on immune cells (49), we speculated that hCG might repair the abnormalities of local immune balance by modulating the action of MSC, and promote embryos implantation.
VCAM1 was expressed on endothelial cells under inflammatory conditions, mediating the recruitment of various subsets of leukocytes (50). At implantation sites, ERA test suggested (19) that VCAM1 was elevated in the endometrium during implantation and experienced a reduction in unexplained infertility patients (51). Our results exhibited a similar trend as the expression of human, VCAM1 was significantly decreased in the mice model, while hCG intrauterine perfusion treatment significantly increased the expression of VCAM1. Previous studies have found out that hCG intrauterine perfusion therapy significantly increased Notch1 expression in the endometrium. As another component of the Notch signaling pathway (45), we speculated that the above changes might be due to the effect of hCG on the Notch signaling pathway in endometrium (49).
GC has a strong immunomodulatory and anti-inflammatory effect, and is used to treat autoimmune diseases and some malignant lymphomas (52). There is an intermodulation between GC in serum and GR in cells, and the reactivity of cells to GC is also associated with GR content, which mainly depends on three mechanisms: (I) combining GR with GC response elements to regulate gene expression directly; (II) interacting with other transcription factors indirectly regulate gene through GR; (III) second messenger Cascade effect mediated by GR. In this study, we found that GR-β in PBMCs was decreased in EID and could regulated six genes above. When GR-β was increased, the rebound of these downstream genes could be found. Clinical efficacy of dexamethasone in the treatment of RIF may be related to this mechanism.
In conclusion, our results indicated that intrauterine administration of hCG co-cultured with PBMCs prior to embryos implantation has a good influence on endometrial receptivity in mice with embryonic implantation dysfunction by regulating LIF and CXCL14. Furthermore, PBMCs might repair the decline of RARRES1 and SLC15A2, and hCG perfusion could improve the expression of VCAM1 and reduce the high expression of MSC. Such infusion could effectively improve the endometrial receptivity and might have an effect on the regulation of immune microenvironment, embryos adhesion and trophoblast invasion. However, considering that endometrial biopsy might cause further damages to patients with RIF, we failed to use human endometrium but endometrium of EID mice model as our test samples. Therefore, there might be differences between the human and the mouse. To our knowledge, this was the first study to comprehensive profile of the molecular regulation of endometrium after hCG co-cultured with PBMCs intrauterine administration. Further studies are needed to determine if these changes of the genes above are helpful to improve implantation failures in infertile populations.
Acknowledgments
We thank the Shanghai Biotechnology Corporation for technical support in the bioinformatics analysis process.
Funding: This work was supported by grants from the National Natural Science Foundation of China (award number: 81571513 & 81771618, recipient: Jing Yang).
Footnote
Conflict of Interest: The authors have no conflicts of interest to declare.
Ethical Statement: The authors are accountable for all aspects of the work in ensuring that questions related to the accuracy or integrity of any part of the work are appropriately investigated and resolved. All animal procedures included in this study conformed to National Institutes of Health guidelines on the ethical use of animals (NIH Publication, revised 2011) and the Guideline for the Care and Use of Laboratory Animals of the Chinese Animal Welfare Committee. All experiments were approved by the Animal Use Committee of Renmin Hospital of Wuhan University (Wuhan, China) (No. 20190705).
References
- Coughlan C, Ledger W, Wang Q, et al. Recurrent implantation failure: definition and management. Reprod Biomed Online 2014;28:14-38. [Crossref] [PubMed]
- European IVF-monitoring Consortium (EIM), European Society of Human Reproduction and Embryology (ESHRE), Calhaz-Jorge C, et al. Assisted reproductive technology in Europe, 2013: results generated from European registers by ESHRE. Hum Reprod 2017;32:1957-73. [Crossref] [PubMed]
- Somigliana E, Vigano P, Busnelli A, et al. Repeated implantation failure at the crossroad between statistics, clinics and over-diagnosis. Reprod Biomed Online 2018;36:32-8. [Crossref] [PubMed]
- Das M, Holzer HE. Recurrent implantation failure: gamete and embryo factors. Fertil Steril 2012;97:1021-7. [Crossref] [PubMed]
- Miravet-Valenciano JA, Rincon-Bertolin A, Vilella F, et al. Understanding and improving endometrial receptivity. Curr Opin Obstet Gynecol 2015;27:187-92. [Crossref] [PubMed]
- Achache H, Revel A. Endometrial receptivity markers, the journey to successful embryo implantation. Hum Reprod Update 2006;12:731-46. [Crossref] [PubMed]
- Tsampalas M, Gridelet V, Berndt S, et al. Human chorionic gonadotropin: a hormone with immunological and angiogenic properties. J Reprod Immunol 2010;85:93-8. [Crossref] [PubMed]
- Licht P, Losch A, Dittrich R, et al. Novel insights into human endometrial paracrinology and embryo-maternal communication by intrauterine microdialysis. Hum Reprod Update 1998;4:532-8. [Crossref] [PubMed]
- Banerjee P, Fazleabas AT. Endometrial responses to embryonic signals in the primate. Int J Dev Biol 2010;54:295-302. [Crossref] [PubMed]
- Yoshinaga K. Review of factors essential for blastocyst implantation for their modulating effects on the maternal immune system. Semin Cell Dev Biol 2008;19:161-9. [Crossref] [PubMed]
- Sen A, Kushnir VA, Barad DH, et al. Endocrine autoimmune diseases and female infertility. Nat Rev Endocrinol 2014;10:37-50. [Crossref] [PubMed]
- Granot I, Gnainsky Y, Dekel N. Endometrial inflammation and effect on implantation improvement and pregnancy outcome. Reproduction 2012;144:661-8. [Crossref] [PubMed]
- Kosaka K, Fujiwara H, Tatsumi K, et al. Human peripheral blood mononuclear cells enhance cell-cell interaction between human endometrial epithelial cells and BeWo-cell spheroids. Hum Reprod 2003;18:19-25. [Crossref] [PubMed]
- Nakayama T, Fujiwara H, Maeda M, et al. Human peripheral blood mononuclear cells (PBMC) in early pregnancy promote embryo invasion in vitro: HCG enhances the effects of PBMC. Hum Reprod 2002;17:207-12. [Crossref] [PubMed]
- Yoshioka S, Fujiwara H, Nakayama T, et al. Intrauterine administration of autologous peripheral blood mononuclear cells promotes implantation rates in patients with repeated failure of IVF-embryo transfer. Hum Reprod 2006;21:3290-4. [Crossref] [PubMed]
- Maleki-Hajiagha A, Razavi M, Rezaeinejad M, et al. Intrauterine administration of autologous peripheral blood mononuclear cells in patients with recurrent implantation failure: A systematic review and meta-analysis. J Reprod Immunol 2019;131:50-6. [Crossref] [PubMed]
- Kao LC, Tulac S, Lobo S, et al. Global gene profiling in human endometrium during the window of implantation. Endocrinology 2002;143:2119-38. [Crossref] [PubMed]
- Haouzi D, Mahmoud K, Fourar M, et al. Identification of new biomarkers of human endometrial receptivity in the natural cycle. Hum Reprod 2009;24:198-205. [Crossref] [PubMed]
- Díaz-Gimeno P, Horcajadas JA, Martínez-Conejero JA, et al. A genomic diagnostic tool for human endometrial receptivity based on the transcriptomic signature. Fertil Steril 2011;95:50-60, 60.e1-15.
- Ruiz-Alonso M, Blesa D, Díaz-Gimeno P, et al. The endometrial receptivity array for diagnosis and personalized embryo transfer as a treatment for patients with repeated implantation failure. Fertil Steril 2013;100:818-24. [Crossref] [PubMed]
- Hu S, Yao G, Wang Y, et al. Transcriptomic changes during the pre-receptive to receptive transition in human endometrium detected by RNA-Seq. J Clin Endocrinol Metab 2014;99:E2744-53. [Crossref] [PubMed]
- Koot YE, van Hooff SR, Boomsma CM, et al. An endometrial gene expression signature accurately predicts recurrent implantation failure after IVF. Sci Rep 2016;6:19411. [Crossref] [PubMed]
- Huang J, Qin H, Yang Y, et al. A comparison of transcriptomic profiles in endometrium during window of implantation between women with unexplained recurrent implantation failure and recurrent miscarriage. Reproduction 2017;153:749-58. [Crossref] [PubMed]
- Yu N, Yan W, Yin T, et al. HCG-activated human peripheral blood mononuclear cells (PBMC) promote trophoblast cell invasion. PLoS One 2015;10:e0125589. [Crossref] [PubMed]
- Yu N, Yang J, Guo Y, et al. Intrauterine administration of peripheral blood mononuclear cells (PBMCs) improves endometrial receptivity in mice with embryonic implantation dysfunction. Am J Reprod Immunol 2014;71:24-33. [Crossref] [PubMed]
- Wu W, Liang J, Chen Y, et al. Microstructural changes are coincident with the improvement of clinical symptoms in surgically treated compressed nerve roots. Sci Rep 2017;7:44678. [Crossref] [PubMed]
- Hashii K, Fujiwara H, Yoshioka S, et al. Peripheral blood mononuclear cells stimulate progesterone production by luteal cells derived from pregnant and non-pregnant women: possible involvement of interleukin-4 and interleukin-10 in corpus luteum function and differentiation. Hum Reprod 1998;13:2738-44. [Crossref] [PubMed]
- Lédée N, Munaut C, Aubert J, et al. Specific and extensive endometrial deregulation is present before conception in IVF/ICSI repeated implantation failures (IF) or recurrent miscarriages. J Pathol 2011;225:554-64. [Crossref] [PubMed]
- Wu C, Chen Z, Dardalhon V, et al. The transcription factor musculin promotes the unidirectional development of peripheral Treg cells by suppressing the TH2 transcriptional program. Nat Immunol 2017;18:344-53. [Crossref] [PubMed]
- Zanchi NE, Filho MA, Felitti V, et al. Glucocorticoids: extensive physiological actions modulated through multiple mechanisms of gene regulation. J Cell Physiol 2010;224:311-5. [Crossref] [PubMed]
- Teles A, Zenclussen AC. How cells of the immune system prepare the endometrium for implantation. Semin Reprod Med 2014;32:358-64. [Crossref] [PubMed]
- Lédée N, Petitbarat M, Chevrier L, et al. The uterine immune profile may help women with repeated unexplained embryo implantation failure after in vitro fertilization. Am J Reprod Immunol 2016;75:388-401. [Crossref] [PubMed]
- Hajipour H, Nejabati HR, Latifi Z, et al. Lymphocytes immunotherapy for preserving pregnancy: mechanisms and challenges. Am J Reprod Immunol 2018;80:e12853. [Crossref] [PubMed]
- Ahmadi M, Abdolmohammadi-Vahid S, Ghaebi M, et al. Regulatory T cells improve pregnancy rate in RIF patients after additional IVIG treatment. Syst Biol Reprod Med 2017;63:350-9. [Crossref] [PubMed]
- Rosario GX, Stewart CL. The multifaceted actions of leukaemia inhibitory factor in mediating uterine receptivity and embryo implantation. Am J Reprod Immunol 2016;75:246-55. [Crossref] [PubMed]
- Camargo-Díaz F, García V, Ocampo-Bárcenas A, et al. Colony stimulating factor-1 and leukemia inhibitor factor expression from current-cycle cannula isolated endometrial cells are associated with increased endometrial receptivity and pregnancy. BMC Womens Health 2017;17:63. [Crossref] [PubMed]
- Stewart CL, Kaspar P, Brunet LJ, et al. Blastocyst implantation depends on maternal expression of leukaemia inhibitory factor. Nature 1992;359:76-9. [Crossref] [PubMed]
- Kuang H, Chen Q, Fan X, et al. CXCL14 inhibits trophoblast outgrowth via a paracrine/autocrine manner during early pregnancy in mice. J Cell Physiol 2009;221:448-57. [Crossref] [PubMed]
- Meuter S, Schaerli P, Roos RS, et al. Murine CXCL14 is dispensable for dendritic cell function and localization within peripheral tissues. Mol Cell Biol 2007;27:983-92. [Crossref] [PubMed]
- Mokhtar NM, Cheng CW, Cook E, et al. Progestin regulates chemokine (C-X-C motif) ligand 14 transcript level in human endometrium. Mol Hum Reprod 2010;16:170-7. [Crossref] [PubMed]
- Lin J, Lojun S, Lei ZM, et al. Lymphocytes from pregnant women express human chorionic gonadotropin/luteinizing hormone receptor gene. Mol Cell Endocrinol 1995;111:R13-7. [Crossref] [PubMed]
- Oldridge EE, Walker HF, Stower MJ, et al. Retinoic acid represses invasion and stem cell phenotype by induction of the metastasis suppressors RARRES1 and LXN. Oncogenesis 2013;2:e45. [Crossref] [PubMed]
- Ferretti C, Bruni L, Dangles-Marie V, et al. Molecular circuits shared by placental and cancer cells, and their implications in the proliferative, invasive and migratory capacities of trophoblasts. Hum Reprod Update 2007;13:121-41. [Crossref] [PubMed]
- Rhinn M, Dolle P. Retinoic acid signalling during development. Development 2012;139:843-58. [Crossref] [PubMed]
- Huebner H, Hartner A, Rascher W, et al. Expression and regulation of retinoic acid receptor responders in the human placenta. Reprod Sci 2018;25:1357-70. [Crossref] [PubMed]
- Zafrakas M, Tarlatzis BC, Streichert T, et al. Genome-wide microarray gene expression, array-CGH analysis, and telomerase activity in advanced ovarian endometriosis: a high degree of differentiation rather than malignant potential. Int J Mol Med 2008;21:335-44. [PubMed]
- Hu Y, Song F, Jiang H, et al. SLC15A2 and SLC15A4 mediate the transport of bacterially derived di/tripeptides to enhance the nucleotide-binding oligomerization domain-dependent immune response in mouse bone marrow-derived macrophages. J Immunol 2018;201:652-62. [Crossref] [PubMed]
- Santarlasci V, Mazzoni A, Capone M, et al. Musculin inhibits human T-helper 17 cell response to interleukin 2 by controlling STAT5B activity. Eur J Immunol 2017;47:1427-42. [Crossref] [PubMed]
- Strug MR, Su R, Young JE, et al. Intrauterine human chorionic gonadotropin infusion in oocyte donors promotes endometrial synchrony and induction of early decidual markers for stromal survival: a randomized clinical trial. Hum Reprod 2016;31:1552-61. [Crossref] [PubMed]
- Schlesinger M, Bendas G. Vascular cell adhesion molecule-1 (VCAM-1)--an increasing insight into its role in tumorigenicity and metastasis. Int J Cancer 2015;136:2504-14. [Crossref] [PubMed]
- Bai R, Bai H, Kuse M, et al. Involvement of VCAM1 in the bovine conceptus adhesion to the uterine endometrium. Reproduction 2014;148:119-27. [Crossref] [PubMed]
- Braitch M, Harikrishnan S, Robins RA, et al. Glucocorticoids increase CD4CD25 cell percentage and Foxp3 expression in patients with multiple sclerosis. Acta Neurol Scand 2009;119:239-45. [Crossref] [PubMed]