Effect of vasa vasorum in cerebrovascular compensation: 2 case reports
The vasa vasorum (VV), also called the “vessel of the vessel”, was first observed by Gimbert in 1865 (1). The majority of VVs are distributed in the tunica adventitia, and the rest extend from the adventitial layer to the tunica media (2). Intracranial VVs are rare and develop predominantly on the proximal segments of the internal carotid artery (ICA) and vertebral artery (VA) (2). However, the typical appearance of intracranial VV has not yet been reported in clinical practice. In this study, we reported the existence of VV in occlused basilar artery (BA) and ICA, which improved downstream perfusion. Based on these two cases, we discuss the positive effects of VV in cerebrovascular occlusion.
Case presentation
Case 1
A 74-year-old woman with hypertension was admitted after experiencing slurred speech and facial drooping on the left side for 6 hours previously. Two months before, she had started experiencing tiredness and decreased alertness. Magnetic resonance imaging (MRI) revealed serious stenosis of the BA and multiple acute infarcts in the right pons, cerebellum, thalamus, and the left cerebral peduncle. Unfortunately, the patient’s family refused endovascular treatment. Three days later, the patient developed more severe symptoms and went into hypnosia. The second MRI showed larger infarcts. We performed an emergency operation once informed consent had been signed. Digital subtraction angiography (DSA) revealed BA occlusion with a stump in the proximal end (Figure 1A) and existence of VV originating from the proximal BA lumen, which penetrated through the vessel wall, bypassed the occlusion, re-penetrated through the vessel wall, and reconnected to the distal BA lumen (Figure 1A). The microguidewire was threaded directly through the occluded segment from the stump to the left posterior cerebral artery in endovascular treatment, avoiding the VV path. Balloon angioplasty was then performed. After these procedures, follow-up DSA images showed that the VV had disappeared (Figure 1B,C).
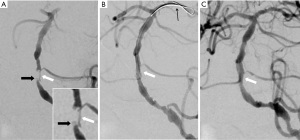
Case 2
A 62-year-old woman with hypertension and a history of stroke was admitted after the onset of severe right hemiparesis. Two years before, she was diagnosed with occlusion in the initial segment of the left ICA (L-C1), and was left with mild aphasia and weakness in her right limbs associated with stroke. She maintained the ability to take care of herself after medical treatments. We performed cerebrovascular assessments. Perfusion-weighted MRI showed a delay in diffuse perfusion in the left cerebral hemisphere. High resolution MRI revealed a narrowed lumen and wall thickening in the left ICA and M1 segment of the middle cerebral arterial (MCA), which was defined as negative reconstitution, and there was no enhancement in the thickened wall. Spectral and color Doppler imaging showed a narrow lumen in L-C1, with a single peak in the blood flow spectrum and a peak velocity of 46 cm/s. VV collaterals were revealed by DSA to penetrate directly through the plaque of obstructive site and to connect to the distal vessel lumen, and low hemodynamic compensation caused by VV and atrophy and collapse of the left ICA were also showed (Figure 2A). There was no collateral circulation from the anterior or posterior communicating arteries. Angioplasty was then performed to establish vessel recanalization in L-C1 and improve the perfusion within the left hemisphere (Figure 2B,C). The patient was prescribed aspirin (100 mg) and clopidogrel (75 mg) after being discharged home. Two months later, DSA confirmed that the VV had disappeared and the lumen of the left ICA had widened (Figure 2D).
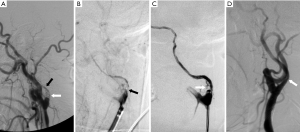
Discussion
Scarcity of intracranial VV
While rare, intracranial VV are essential for supplying nutrients and oxygen (3). VV are found in 67%, 45%, and 13% of VA, ICA, and BA, respectively, as well as in the distal cerebral arteries (MCA, ACA). Furthermore, the distribution of VV is very sparse (M1: 5%, A1: 2%) (2). Intracranial arteries have thinner media and adventitia than comparably sized extracranial vessels (4). The nutrient-rich cerebrospinal fluid (CSF) that bathes the adventitial surface probably contributes to the decreased demand for supplemental nourishment from the VV (5). Therefore, differences in the vascular structure and external environment of the arteries may be relative to the rarity of intracranial VV.
Methods of observing VV
In clinical practice, VV could be visualized directly by several imaging modalities, including microcomputed tomography (micro-CT), contrast-enhanced ultrasound, intravascular ultrasound, optical coherence tomography, contrast agent-enhanced MRI (6) and DSA. However, for observing intracranial VV, only contrast agent-enhanced MRI and DSA were the feasible modalities. There were challenges in interpreting the MR images. Notably, the plaque could be enhanced by the contrast agent as well as the VV. Although the VV were an important contributing factor to contrast agent uptake in the plaque, the mechanism of plaque enhancement was complex, and the VV were not the sole contributors. The small size of intracranial vessels can also increase the difficulty in identifying vessel wall enhancement. In addition, cerebral aneurysms, intracranial dissections, and intracranial vasculitis, which are related to the occurrence of VV, can express contrast enhancement (7). DSA seemed to be a prospective approach for observing intracranial VV, because although the VV were usually very small, often below the resolving power of DSA, thickened VV could be shown by DSA.
Clinical evidence of intracranial VV
There is not a typical description of intracranial VV in the literature of clinical practice. Muthusami et al. (8) reported a case of spontaneous revascularization caused by collaterals reconstituting distal flow in a chronically occluded MCA branch of a 9-year old boy. However, the collateral segment manifested multiple tortuous channels on DSA images, mimicking a brain arteriovenous malformation. We presented the case of typical VV which improved downstream perfusion in occluded BA (Sig. 1a). The VV originated from the proximal BA lumen, penetrated through the vessel wall, bypassed the occlusion, re-penetrated through the vessel wall, and reconnected to the distal BA lumen. After the occluded BA was recanalized by endovascular treatment, the VV were not detected in the following cerebral angiogram. Harnoss et al. (9) produced a porcine model by minimally invasively implanting a copper stent into the coronary artery, resulting in a novel semi-acute vessel occlusion. The micro-CT angiogram imaging of the coronary arteries clearly showed VV collaterals, which closely resembled the DSA images of VV in our case. They considered that the hemodynamic pressure difference between the two ends of the occlusion played an important role in the occurrence and development of VV. In our case, the relief of hemodynamic pressure difference resulting from BA recanalization caused the immediate closure of the VV collaterals. This phenomenon indicated that the effect of hemodynamic pressure difference was reversible. Since the VV bypassed the occlusion from the tunica adventitia or the outer layer, the microguidewire could not follow the path of the VV in the operation.
Growing paths of VV in ICA
Several reports have studied revascularization in the ICA via VV after occlusion (10,11). The VV originated from the proximal vessel lumen and penetrated through the vessel wall, forming an irregular and tortuous vascular channel across the occlusion, then penetrated the wall again and reconnected to the distal ICA lumen. The connection points could be either close to the occlusion, or at the distantly cavernous segment (11). However, far less attention has been paid to the idea that VV connect the two ends of the occlusion through the plaque. In our case, the DSA images clearly showed that the VV collaterals penetrated directly through the plaque leading to occlusion from the proximal to the distal vessel lumen, causing low flow compensation (Figure 2A). Although the pathogenesis of atherosclerosis is inseparable from the appearance of VV, VV conventionally penetrate from the adventitia and outer layers of the media to the tunica intima, supplying them with nutrients and oxygen (3). Our case suggested that the VV did not necessarily follow the conventional growth path. Although the majority of the VV penetrate though the vascular wall, under certain conditions, the VV in plaque may become the mainstream and eventually reconnect the two ends of the occlusion. After VV reconnection, the difference in hemodynamic pressure between the two ends of the occlusion declined and the ischemia and hypoxia were improved. When they reached an equilibrium point, the angiogenesis would stop. As the VV reconnected the distal lumen from the plaque in the vessels, the microguidewire could be guided along the VV path in endovascular operation.
The occurrence of VV was not itself pathological, but was rather a normal physiological response triggered by the oxygenation and nutritional needs of the artery. Obstruction, hypoperfusion, or endothelial barrier leakiness could reduce oxygen and nutrient metabolism and trigger the occurrence of VV (3). The VV would follow paths which benefited revascularization and improvement of downstream perfusion. From this point of view, VV could be regarded as third-level collateral compensation in the cerebrovascular system. Moreover, VV could also prove useful in endovascular treatment, depending on whether the microguidewire is able to follow the VV path.
Conclusions
Arterial occlusion, including in the intracranial and extracranial artery, could trigger the occurrence of VV, which can improve downstream perfusion. VV could be regarded as third-level collateral compensation in the cerebrovascular system and play a role of signal light in endovascular treatment.
Acknowledgments
Funding: This work was supported by (Science and Technology Planning Project of Guangdong Province) grant number (2016A020226040).
Footnote
Conflicts of Interest: All authors have completed the ICMJE uniform (available at http://dx.doi.org/10.21037/atm.2020.03.77). The authors have no conflicts of interest to declare.
Ethical Statement: The authors are accountable for all aspects of the work in ensuring that questions related to the accuracy or integrity of any part of the work are appropriately investigated and resolved. Written informed consent was obtained from the patients for publication of this manuscript and any accompanying images.
Open Access Statement: This is an Open Access article distributed in accordance with the Creative Commons Attribution-NonCommercial-NoDerivs 4.0 International License (CC BY-NC-ND 4.0), which permits the non-commercial replication and distribution of the article with the strict proviso that no changes or edits are made and the original work is properly cited (including links to both the formal publication through the relevant DOI and the license). See: https://creativecommons.org/licenses/by-nc-nd/4.0/.
References
- Gimbert JL. Mémoire sur la structure et la texture des artères. Paris, France: Adrien Delahaye, Libraire-Editeur, 1865.
- Takaba M, Endo S, Kurimoto M, et al. Vasa vasorum of the intracranial arteries. Acta Neurochir (Wien) 1998;140:411-6. [Crossref] [PubMed]
- Fibla M, Hernández-Aguilera A, Camps J, et al. Treating atherosclerosis: targeting risk factors should not be the only option. Ann Transl Med 2018;6:S34. [Crossref] [PubMed]
- Baker AB. Structure of the small cerebral arteries and their changes with age. Am J Pathol 1937;13:453-62. [PubMed]
- Zervas NT, Liszczak TM, Mayberg MR, et al. Cerebrospinal fluid may nourish cerebral vessels through pathways in the adventitia that may be analogous to systemic vasa vasorum. J Neurosurg 1982;56:475-81. [Crossref] [PubMed]
- Sun Z. Atherosclerosis and atheroma plaque rupture: imaging modalities in the visualization of vasa vasorum and atherosclerotic plaques. ScientificWorldJournal 2014;2014:312764.
- Portanova A, Hakakian N, Mikulis DJ, et al. Intracranial vasa vasorum: insights and implications for imaging. Radiology 2013;267:667-9. [Crossref] [PubMed]
- Muthusami P, Krings T, Raybaud C, et al. Intracranial artery to artery spontaneous revascularization in a child. Childs Nerv Syst 2017;33:2035-8. [Crossref] [PubMed]
- Harnoss JM, Krackhardt F, Ritter Z, et al. Porcine arteriogenesis based on vasa vasorum in a novel semi-acute occlusion model using high-resolution imaging. Heart Vessels 2017;32:1400-9. [Crossref] [PubMed]
- Meguro T, Muraoka K, Terada K, et al. Recanalisation of the internal carotid artery via the vasa vasorum after coil occlusion. Br J Radiol 2011;84:e23-6. [Crossref] [PubMed]
- Colon GP, Deveikis JP, Dickinson LD. Revascularization of occluded internal carotid arteries by hypertrophied vasa vasorum: report of four cases. Neurosurgery 1999;45:634-7. [Crossref] [PubMed]