Effects of gamma irradiation on the measurement of hepatitis B virus DNA in dentin harvested from chronically infected patients
Introduction
The demineralized dentin matrix (DDM) has become a commonly used bone-graft substitute that is fabricated from the discarded extracted teeth. DDM consists of acid-insoluble type I collagenous scaffolds that contain non-collagenous proteins such as bone morphogenetic protein. Even though dentin is remarkably similar to bone with regard to its chemical composition and biological behavior, dentin is an acellular matrix without blood vessels, while bone includes osteocytes and blood vessels. Since DDM is an acellular and avascular matrix that displays antigenicity, human DDM can be biorecycled as an alternative to an autogenous bone graft, familial graft, or allograft (1). In several in vivo and clinical studies involving biological family members, DDM facilitated successful outcomes as an allogenic bone substitute without any immunological complications (2,3). However, similar to allogenic bone grafts, certain viral diseases (hepatitis B and C, and human immunodeficiency viruses) may be transmitted through infected human-based implantation materials, necessitating valid viral clearance (4).
Various allograft processing procedures aimed to ensure virus inactivation have been introduced and evaluated, including demineralization, use of detergents, freeze-drying, chemical sterilization, and gamma irradiation. These methods provide an exponential reduction in the potential graft contamination and disease transfer (5,6). The process of bone demineralization has been shown to drastically reduce the infectivity of all RNA and DNA viruses with an overall sterility assurance level greater than one million (10−6) for all viruses and as much as one trillion (10−12) for poliovirus (7). Similarly, processed DDM obtained from a Hepatitis B virus-infected (HBV-infected) donor showed complete viral inactivation after demineralization, whereas unprocessed DDM from the same HBV-infected donor showed active HBV DNA copy numbers (4). A low-dose gamma irradiation process is known to inactivate both enveloped and non-enveloped viruses containing either DNA or RNA, thus providing an additional assurance against the occurrence of viral transmission during bone graft procedures (8). According to the guidelines of the European Association of Tissue Banks (EATB) and the International Atomic Energy Agency (IAEA), the permissible dose for gamma irradiation is 25 kGy, whereas the American Association of Tissue Banks (AATB) suggests a dose of 15 kGy (8,9).
HBV has been detected in virtually all bodily secretions and excretions. However, only blood, visibly bloody body fluids, semen, and vaginal secretions pose a risk of transmission (10). HBV is transmitted by percutaneous and mucosal exposure to either infective blood or body fluids (10). HBV is stable on environmental surfaces for at least seven days and is 100 times more infectious than the human immunodeficiency virus (HIV). Several factors such as the viral load of the source can influence the risk of infective HBV transmission (10). In addition to its clinical feasibility, the use of DDM as an allogenic bone-graft substitute is hindered by the possibility that the allogenic dentin from infected patients can facilitate the transmission of viral diseases. Moreover, if a potential risk of viral transmission exists, it is necessary to determine whether terminal sterilization via gamma irradiation (GR) provides a sufficient reduction of the risk of transmission.
This study aimed to estimate the capability of GR using the gamma irradiation doses of 15 and 25 kGy to eliminate or inactivate HBV in dentin obtained from chronically HBV-infected patients by measuring the levels of HBV DNA contained within the pre- and post- irradiated dentin. It was hypothesized that GR sterilization of the fresh dentin matrix could completely inactivate or eliminate any HBV present within the matrix, preventing infectious transmission of HBV during transplantation.
Methods
This study was approved by the Seoul National University Bundang Hospital Institutional Review Board (SNUBH IRB No. B-1705/395-308), and all of the participants provided informed consent. All participants were chronically infected with HBV and were negative for HIV and hepatitis C virus antibodies, and vertically infected infants and child patients were excluded. 18 teeth were extracted from the participants, and all of the extractions were clinically justified. The HBV infection of each patient was verified by serological examinations exhibiting titers over 1,000 international units (IU)/mL (Table 1). After extraction, the teeth were placed in sterile bottles and stored in a refrigerator. Then, the teeth were sealed without contamination and transferred on dry ice to a manufacturer (Korea Tooth Bank, Seoul, Korea). To prevent the degradation of the HBV DNA, each tooth was packaged in a new sterile bottle and stored at −20 °C while awaiting HBV measurements.
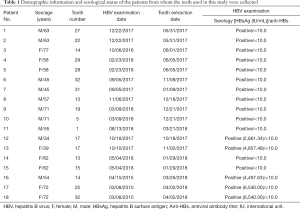
Full table
Experimental design
The dentin harvested from each HBV-infected patient was divided into three samples. The first sample was examined in HBV DNA measurements; these samples were not processed and were used as the control group. The second sample underwent gamma irradiation with a dose of 15 kGy (experimental group), and the third sample underwent gamma irradiation with a dose of 25 kGy (experimental group). After each experimental group underwent GA sterilization, the samples were analyzed for HBV DNA. The permissible doss of 15 and 25 kGy GR were determined by the guidelines of AATB, EATB, and IAEA (8,9).
Preparation of dentin for measurement of HBV DNA.
Each tooth was debrided of all cavities, calculus, soft tissues, previous restoration material, and root canal materials with a dental high-speed burr (FG 6856 018 Diamond Bur; Gebr. Brasseler GmbH & Co, Lemgo, Germany) and was irrigated with saline. Above the cemento-enamel-junctions, the enamel was removed using an FG700 010 Cutting Burr (Gebr. Brasseler GmbH & Co, Lemgo, Germany). All of the attached hard tissues, dental pulp, and soft tissues were debrided with a dental handpiece. The root cementum portion was eliminated by scraping with an FG 6856 018 Diamond Burr and #15 surgical blade. The remaining root matrix was split into three pieces using a FG700 010 Cutting Burr (Gebr. Brasseler GmbH & Co, Germany).
To identify the level of HBV DNA, the control group fragments were cleaned with sterile water and dried in a chamber at 56 °C for 2 h (Figure 1A). The experimental group fragments were gamma-irradiated with the doses of 15 or 25 kGY (Figure 1B,C) at Greenpia Technology (Greenpia Technology INC. R&D Center, Yeoju, Korea) using a Gamma-ray (Co-60) survey facility instrument (JS-8900 MDS Nord International Co. Ltd, Ottawa, ON, Canada). The total absorbed dose of GR was obtained at room temperature (14±1 °C) at a dose rate of 1 kGy per unit time. A dosimetry system (Harwell RED 4034 Dosimeters, Harwell Dosimeters LTD, Oxfordshire, UK) was used following the IAEA standards to verify the standardization of the absorbed doses, with the total absorbed dose error obtained as less than 8%.
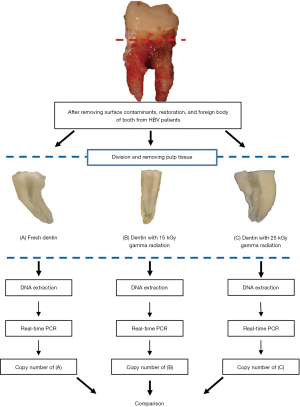
DNA isolation and purification
In preparation for the quantitative polymerase chain reaction, each sample of the fresh and irradiated dentin (0.5 g) underwent digestion and decalcification using a TBONE EX Kit (DNA Chip Research Inc., Tokyo, Japan) via phenol-chloroform extraction. Following the manufacturer instructions, total DNA was evaluated as follows. The samples were placed into Solution A (30 mL) in 50-mL tubes. After soaking in Solution, A at 23 °C for 12 h, Solution B (1.8 mL) was added into each tube. Then, the mixture was agitated gently at 37 °C for 2 h, followed by 5 min of centrifuging at 13,000×g. After processing, Solution C (400 µL) and Proteinase K (20 mg/mL, 50 µL) were added to the resulting supernatant, and the mixture was incubated under gentle agitation at 56 °C for 3 h (Table S1).
The resultant pure DNA solution from each sample was obtained using an QIAamp DNA Investigator Kit (Qiagen, Hilden, Germany, Table S1). Using a silica membrane, the DNA samples were purified, as described in detail in Table S1. The purified DNA was filtered in an adipose tissue extracts buffer (100 µL, Buffer ATE; 10 mM Tris-HCl, 0.1 mM EDTA, 0.04% sodium azide, Table S1).
Quantitative polymerase chain reaction
A Hepatitis B Virus polymerase chain reaction (PCR) Kit (MyBiosource, Inc., San Diego, CA, USA) with oasig lyophilized 2X qPCR Mastermix (Primerdesign, Ltd., Camberley, UK) and a 7900HT Fast Real-Time PCR System (Applied Biosystems, Foster City, CA, USA) were used for quantitative real-time PCR (qPCR). As per the manufacturer’s instructions, the PCR reactions were performed by aliquoting the master mix (15 µL) into the individual reaction wells of a 96-well reaction plate (Applied Biosystems, Foster City, CA, USA), followed by the addition of each experimental DNA sample (5 µL) to obtain the final volume of the reaction well (20 µL), as described in detail in Table S1. The ‘standard DNA’ was set up from six concentrations of a reference DNA sample that were prepared by serial dilution and analyzed in triplicate for each of the 96-well plates in the study. All of the DNA samples were analyzed in triplicate. The thermal cycling profile included two stages, namely Stage 1 of 2 min at 95 °C; and Stage 2 of 50 cycles of 10 s at 95 °C and 60 s at 60 °C followed by signal acquisition (Table S1).
Data and statistical analysis
A real-time PCR thermal cycler software (SDS 2.4.1 for the 7900HT) was used to analyze the data. Fluorescence data were normalized using the ROX (6-Carboxyl-X-Rhodamine, lmax =610 nm) signal, and both the threshold and baseline signals were activated automatically. The HBV DNA copy numbers in the radiation sterilized dentin were compared to those of the fresh dentin harvested from the same tooth. Due to the limited number of samples, neither cut-off levels nor specificity could be determined.
All statistical analyses were performed using the R statistical software (version 3.5.1; R Foundation for Statistical Computing, Vienna, Austria). The Wilcoxon signed-rank test was used for the comparison of the HBV DNA copy numbers of the control and experimental groups, and a difference was considered to be significant if the observed P value were less than 0.05.
Results
HBV DNA in fresh dentin
Among the 18 fresh dentin samples from the HBV patients, 12 specimens (66.67%) were found to contain HBV DNA. In the other six fresh dentin samples, HBV DNA was not measured either due to insufficient DNA quality or due to insufficient amounts for analysis (Figure 2).
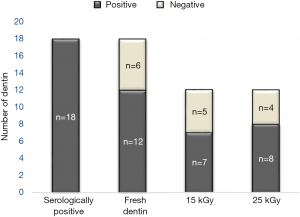
The HBV DNA of fresh dentin was significantly different from that of the radiation-sterilized dentin. Confirming the statistical significance of the results, for the experimental groups exposed to the GR doses of 15 and 25 kGy, the Wilcoxon signed-rank test found P value of 0.012 and 0.010, respectively. There was no observed significant difference between the radiation doses of 15 and 25 kGy (P=0.721).
HBV DNA in dentin gamma-irradiated with doses of 15 and 25 kGy
The HBV DNA in 5 (41.67%) and 4 (33.33%) of the 12 dentin samples known to be positive for HBV DNA was observed to be eliminated or degraded by GR doses of 15 and 25 kGy, respectively. HBV DNA copy numbers of 3.51, 11.34, and 1.43 were observed in the radiation-sterilized dentin from patients #15, #11, and #9, respectively; these samples corresponded to copy numbers greater than 10 (53.43, 46.67, and 16.35, respectively) in the fresh dentin samples, demonstrating that the viral DNA in these samples underwent extensive degradation by GR at a dose of 15 kGy. After the GR exposure of 25 kGy, the samples obtained from patients #15, #11, and #9 also demonstrated extensive degradation of the HBV DNA.
Eleven of the twelve (with the exception of patient #11 for irradiation with 15 kGy and of patient #15 for irradiation with 25 kGy) fresh dentin samples (91.67%) with the HBV DNA copy numbers of less than 10.0 were correlated with the samples that showed negative results for HBV after the irradiation with both 15 kGy and 25 kGy. This is considered to indicate a complete elimination or inactivation of HBV (Figure 3, Table S2).
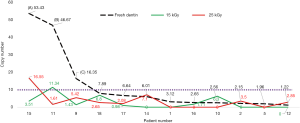
Discussion
The authors hypothesized that GR exposure with a dose of either 15 or 25 kGy inactivated HBV by degrading its nucleic acids, rendering it noninfectious. The viral load in the dentin harvested from infected patients may be the principal factor for determining the methods used in terminal sterilization to prevent the transmission of any viral infections. Based on the results of the current study, dentin may be a potential carrier of HBV, as indicated by the fact that 66.67% (12/18) of the fresh dentin samples tested positive for HBV DNA by qPCR. GR with the doses of either 15 or 25 kGy was shown to significantly reduce the HBV DNA in fresh dentin. A statistically significant reduction in the measured quantity of HBV DNA was achieved in five samples with 15 kGy (41.67%) and in four samples with 25 kGy (33.33%) within a total of twelve samples. Among the twelve HBV-DNA-positive fresh dentin samples, HBV DNA persisted in eleven of the post-irradiation dentin samples (91.67%) that were reduced to below copy number of 10, except for the dentin in sample #11 after 15 kGy of GR and the dentin in sample #15 after 25 kGy of GR. The highest copy numbers of 53.43, 46.67, and 16.35 in fresh dentin samples were reduced to 3.51, 11.34, and 1.43 after 15 kGy and 16.55, 1.61, and 5.42 after 25 kGy, respectively. Even though the actual cut-off levels and specificity were not determined in the current experiment, a copy number of less than 10 is generally considered to be negative for viral infection. Therefore, the validation of the viral inactivation by GR with either 15 or 25 kGy doses in dentin performed in this work may enable the expanded allogenic application of DDM from the GR-sterilized dentin. In this work, we observed no sterilization difference as measured by the HBV DNA levels between the radiation dose sizes of 15 and 25 kGy.
The DDM derived from dentin consists of osteoconductive type 1 collagen and non-collagenous proteins including the osteoinductive bone morphogenetic proteins. The procedure used to generate DDM involves washing, demineralization with organic solvents, freeze-drying, and sterilization, and should result in a significant level of viral inactivation in a similar fashion to processing of a demineralized bone matrix (DBM) (5). In 2018, it was proven that the procedure for producing DDM also extensively reduced the level of HBV DNA (4). The major differences between DDM and DBM are that bone contains viable osteocytes and blood vessels (haversian canals and endothelial cells), while there are neither cells nor blood vessels in dentin that may be a potential source of transmissible viruses (1). Because dentin is obtained from patients during dental treatment, the possibility of disease transmission via a dentin allograft may be lower than that from a bone allograft. Based on these reasons, a DDM allograft may be a safer alternative to a bone allograft in terms of viral disease transmission (11,12). Recent animal studies have shown promising results for the xenografts of DDM with the absence of any immunological complications (13,14). Furthermore, some clinical trials reported the successful results of nonpathogenic allografts derived from within a biological family (2,3). However, little evidence has been reported regarding the effective sterilization processes for infectious pathogens in DDM allografts derived from individuals with various medical conditions.
GR is a simple, cost-effective, and popular sterilization technique in bone allografts that is known to inactivate both enveloped and non-enveloped viruses. GR will fully penetrate tissue and thus has no exposure dependence on the tissue size or dimensions (15,16). Hilmy et al. showed that the clinical efficacy of long bones after a GR irradiation process with 25 kGy shows no prominent difference from the efficacy of the aseptically prepared bones (17). Various guidelines have been suggested for the optimal minimum dose of GR to ensure sterilization, with a 35 kGy dose suggested in some studies to attain sterility assurance levels (18).
One of the disadvantages of GR is its hazardous effects on the osteoinduction properties of bone allografts tissues such as the DBM. Glowacki et al. reported that a 35–50 kGy GR dose at the ambient temperature can eliminate the osteoinductive properties of demineralized bone powder. Additionally, it was found that a 20 kGy GR dose resulted in a 20 percent decrease in osteoinduction (19). Even though the effects of gamma irradiation on the osteoinductivity of DDM could be extrapolated from DBM, more studies are required to determine the specific effects of the doses of 15 and 25 kGy on the osteoinductivity of DDM (20-22).
As previously noted, it is currently not known whether or not the HBV DNA in fresh dentin is infectious because we did not determine the associated cutoff levels of the HBV DNA in the present experiments. Another limitation of the present study is the small number of dentin samples procured from the chronically HBV-infected patients. It is also noteworthy that the relatively low transmissible virus density in chronically infected dentin may be closely related to the acellular and avascular characteristics of the dentin matrix, and may suggest that doses in the 15–25 kGy range may be excessive for low viral load densities but necessary for the inactivation of high-density HBV.
Conclusions
The results of this study suggest that GR dose of 15–25 kGy significantly reduced the levels of HBV DNA. Despite the adverse effects of GR on bone osteoinductivity, the terminal sterilization of human DDM by GR appears to provide an expanded margin of safety against both HBV and the viruses that are currently not tested or are unknown for allogenic application. To achieve completely virus-free grafting DDM, additional studies, including both the validations of viral load inactivation and the investigations of the effects of GR exposure on the osteoinductivity of DDM, are necessary. In addition, despite its low copy number, testing of the infective potential of the HBV DNA that persisted after the GR sterilization may be required.
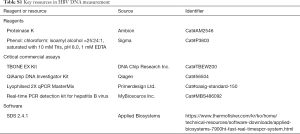
Full table
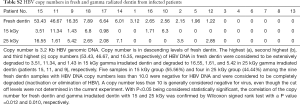
Full table
Acknowledgments
Funding: This research was supported by a grant from the Korea Health Technology R&D Project through the Korea Health Industry Development Institute (KHIDI) funded by the Ministry of Health & Welfare, Republic of Korea (grant number: HI15C1535) (to Dr. BJ Kim, Dr. YM Kim, and Dr. IW Um).
Footnote
Conflicts of Interest: The authors have no conflicts of interest to declare.
Ethical Statement: The authors are accountable for all aspects of the work in ensuring that questions related to the accuracy or integrity of any part of the work are appropriately investigated and resolved. The study was conducted following the World Medical Association Helsinki Declaration and ethical principles Committee of the Seoul National University Bundang Hospital Institutional Review Board approved the study (SNUBH IRB No. B-1705/395-308). All participants signed informed written consent.
Open Access Statement: This is an Open Access article distributed in accordance with the Creative Commons Attribution-NonCommercial-NoDerivs 4.0 International License (CC BY-NC-ND 4.0), which permits the non-commercial replication and distribution of the article with the strict proviso that no changes or edits are made and the original work is properly cited (including links to both the formal publication through the relevant DOI and the license). See: https://creativecommons.org/licenses/by-nc-nd/4.0/.
References
- Murata M, Okubo N, Shakya M, et al. Dentin materials as biological scaffolds for tissue engineering. Biomater Regenerative Med 2019. doi:
10.5772/intechopen.85452 - Kim YK, Bang KM, Murata M, et al. Retrospective clinical study of allogenic demineralized dentin matrix for alveolar bone repair. J Hard Tissue Biol 2017;26:95-102. [Crossref]
- Um IW, Jun JH, Yun PY, et al. Histological comparison of autogenous and allogenic demineralized dentin matrix loaded with recombinant human bone morphogenetic protein-2 for alveolar bone repair: a preliminary report. J Hard Tissue Biol 2017;26:417-24. [Crossref]
- Um IW, Choi SW, Kim YK, et al. Measurement of hepatitis B virus DNA in fresh versus processed dentin from chronically infected patients. J Transl Med 2018;16:351. [Crossref] [PubMed]
- Swenson CL, Amoczky SP. Demineralization for inactivation of infectious retrovirus in systemically infected cortical bone. J Bone Joint Surg Am 2003;85:323-32. [Crossref] [PubMed]
- Asselmeier MA, Caspari RB, Bottenfield S. A review of allograft processing and sterilization techniques and their role in transmission of the human immunodeficiency virus. Am J Sports Med 1993;21:170-5. [Crossref] [PubMed]
- Holtzclaw D, Toscano N, Eisenlohr L, Callan D. The Safety of Bone Allografts Used in Dentistry: A Review J Am Dent Assoc 2008;139:1192-9. [Crossref] [PubMed]
- Moore MA. Inactivation of enveloped and non-enveloped viruses on seeded human tissues by gamma irradiation. Cell Tissue Bank 2012;13:401-7. [Crossref] [PubMed]
- Nguyen H, Morgan DA, Forwood MR. Sterilization of allograft bone: is 25 kGy the gold standard for gamma irradiation? Cell Tissue Banking 2007;8:81-91. [Crossref] [PubMed]
- Tsai CY, Lin CL, Lin SC, et al. Detection of Hepatitis B Virus in the Aqueous Humor of a Hepatitis B Virus Carrier. Ophthalmologica 2009;223:93-5. [Crossref] [PubMed]
- Joshi CP, D'Lima CB, Samat UC, et al. Comparative alveolar ridge preservation using allogenous tooth graft versus free-dried bone allograft: A randomized, controlled, prospective, clinical pilot study. Contemp Clin Dent 2017;8:211-7. [Crossref] [PubMed]
- Joshi CP, D’Lima CB, Karde PA, et al. Ridge augmentation using sticky bone: A combination of human tooth allograft and autologous fibrin glue. J Indian Soc Periodontol 2019;23:493-6. [PubMed]
- Al-Asfour A, Farzad P, Al-Musawi A, et al. Demineralized xenogenic dentin and autogenous bone as onlay grafts to rabbit tibia. Implant Dent 2017;26:232-7. [Crossref] [PubMed]
- Gomes MF, Valva VN, Vieira EM, et al. Homogenous demineralized dentin matrix and platelet-rich plasma for bone tissue engineering in cranioplasty of diabetic rabbits: biochemical, radiographic, and histological analysis. Int. J. Oral Maxillofac. Surg 2016;45:255-66. [Crossref] [PubMed]
- Nguyen H, Morgan DA, Forwood MR. Sterilization of allograft bone: Effects of gamma irradiation on allograft biology and biomechanics. Cell Tissue Bank 2007;8:93-105. [Crossref] [PubMed]
- Islam MM, Sharifi R, Mamodaly S, et al. Effects of gamma radiation sterilization on the structural and biological properties of decellularized corneal xenografts. Acta Biomater 2019;96:330-44. [Crossref] [PubMed]
- Hilmy N, Lina M. Effect of ionising radiation on viruses, proteins and prions. In: Nather A, editor. Advances in Tissue Banking. 5th ed. The Scientific Basis of Tissue Transplantation. Singapore: World Scientific Publication, 2001:358-74.
- Türker NS, Özer AK, Kutlu B, et al. The Effect of Gamma Radiation Sterilization on Dental Biomaterials. Tissue Engineering and Regenerative Medicine 2014;11:341-9. [Crossref]
- Glowacki J. A review of osteoinductive testing methods and sterilization processes for demineralized bone. Cell Tissue Bank 2005;6:3-12. [Crossref] [PubMed]
- Arjmand B, Aghayan HR, Larijani B, et al. The effect of gamma irradiation on the osteoinductivity of demineralized human bone allograft. Acta Med Iran 2014;52:215-9. [PubMed]
- Pekkarinen T, Hietala O, Jämsä T, et al. Effect of gamma irradiation on the osteoinductivity of morphogenetic protein extract from reindeer bone. Acta Orthop 2005;76:231-6. [Crossref] [PubMed]
- Dziedzic-Goclawska A, Ostrowski K, Stachowicz W, et al. Effect of radiation sterilization on the osteoinductive properties and the rate of remodeling of bone implants preserved by lyophilization and deep-freezing. Clin Orthop Relat Res 1991;30-7. [PubMed]