Two-dimensional speckle tracking echocardiography detected interventricular dyssynchrony predicts exercise capacity and disease severity in pre-capillary pulmonary hypertension
Introduction
Pulmonary hypertension (PH) describes a group of disorders that cause pulmonary vascular remodeling and pulmonary artery pressure increase, which subsequently increases the afterload of right ventricle (RV) and ultimately leads to progressive RV failure (1). Pre-capillary PH (PcPH), defined as a pulmonary artery wedge pressure (PAWP) ≤15 mmHg and a pulmonary vascular resistance (PVR) >3 Wood units (WU), includes pulmonary arterial hypertension (PAH), chronic thromboembolic PH (CTEPH) and PH due to other diseases, except left heart disease (1). It is well established that the progression of PH results in an increase of RV afterload and eventually systolic dysfunction of RV, simultaneously, left ventricular (LV) dysfunction has been gradually recognized by researchers, even in mild PH patients (2). The bilateral ventricles are anatomically and functionally coupled (3), and the dysfunction of one ventricle will inevitably affect the other. However, little attention has been paid to biventricular dysfunction in PcPH patients.
Impaired exercise capacity is one of the typical symptoms of PH, and it is often used to evaluate disease severity and prognosis as well as to determine the indication and opportunity for heart and/or lung transplantations (4,5). The 2015 European Society of Cardiology (ESC) Guidelines for the diagnosis and treatment of PH recommended that the basic assessment of PH patients include WHO functional class (WHO-FC) and exercise capacity, which is detected by a cardiopulmonary exercise test (CPET) or a six-minute walk test (6MWT) (6). Peak oxygen consumption (PVO2), characterized by objective and digital appraisal of monolithic cardiopulmonary functions, is one of the most widespread CPET parameters used for therapeutic decision making (7,8). However, in patients with certain contraindications, such as uncontrolled symptomatic heart failure, CPET may be associated with procedural risks (9). Additionally, some of the indices of the 2015 ESC risk assessment strategy (6) were invasive, such as right heart catheterization (RHC). Therefore, we need safer and more convenient evaluation techniques for risk assessment of PH patients, especially for those with absolute or relative contraindications to RHC or CPET.
As a low-cost, noninvasive and reliable tool, echocardiography is widely used to assess the structure and function of heart. Recent studies have shown that impaired RV longitudinal strain, strain rate and intraventricular mechanical dyssynchrony detected by two-dimensional speckle tracking echocardiography (2D-STE) were correlated with a decrease in RV contractile efficiency (10), impaired exercise capacity (11,12), invasive hemodynamic parameters (13) and worse prognosis (14,15) in PH patients without obvious electrical dyssynchrony, which was identified by QRS duration >120 ms based on electrocardiography (ECG). Furthermore, simultaneous LV dyssynchrony was observed in patients with PH, quantified by STE imaging or cardiac magnetic resonance imaging (CMR) (16,17). We hypothesize that both inter- and intraventricular dyssynchrony may correlate with decreased exercise capacity. Therefore, we first proposed 2D-STE to detect interventricular dyssynchrony and investigated the predictive value of interventricular dyssynchrony for exercise capacity and risk assessment according to the 2015 ESC Guidelines in PcPH patients.
Methods
This is a single-center study from the Pulmonary Vascular Disease Center of Chinese Academy of Medical Sciences, Fuwai Hospital. Sixty-six patients diagnosed with PcPH according to the 2015 ESC Guidelines were consecutively enrolled from April 2017 to March 2018, the sample included 44 patients with idiopathic PAH (IPAH, 66.7%), 10 with CTEPH (15.2%), 8 with connective tissue disease induced PAH (CTD-PAH, 12.1%) and 4 with PAH after surgery for congenital heart diseases (6.1%). The exclusion criteria were as follows: (I) intracardiac right-to-left or left-to-right shunts; (II) QRS duration >120 ms; (III) arrhythmia at the time of enrollment; (IV) significant left heart diseases or valve disorders; (V) acute heart failure; (VI) renal or hepatic failure; and (VII) other concomitant diseases, such as hypertension and diabetes.
Standard transthoracic echocardiography was performed in 66 PcPH patients using Vivid S6 equipment (GE Medical Systems) with a 2.5- to 3.5-mHz probe by an experienced investigator. Standard M-mode, 2D and Doppler images were obtained from at least 3 consecutive beats. Then, GE EchoPAC version 201 was used to assess multiple parameters as follows: RV areas were measured from 2D imaging at two points—end ventricular systole, corresponding to end atrial diastole and identified as the frame just before tricuspid valve opening; and end ventricular diastole was identified as the point before the ventricular contraction, corresponding to end atrial systole. Then, the formula (Areamax − Areamin)/Areamax was used to calculate the RV fractional area change (FAC). Left ventricular ejection fraction (LVEF, 2D imaging), Doppler velocities of the trans-tricuspid flow (E, A), Doppler velocities of the tricuspid annulus (E', S', pulsed Doppler tissue image) and tricuspid annular plane systolic excursion (TAPSE, M-mode tracings) were measured according to the guidelines of the American Society of Echocardiography (18).
Standard grayscale 2D images in 4-chamber and RV-focus 4-chamber views were obtained for speckle-tracking analyses using GE EchoPAC version 201, which required at least 3 consecutive beats and a frame rate >40 fps. The RV and LV endocardial borders were traced and fine-tuned manually to ensure that the six segments (basal, middle and apical of the free wall and interventricular septum) were tracked appropriately (insert Figure 1, left), then the time-strain longitudinal curves of each segment and RV global longitudinal strain (RV-GLS) were generated (insert Figure 1, right). The time intervals between QRS onset and peak longitudinal systolic strain were calculated for all RV/LV segments and corrected for heart rate according to Bazett’s formula (19). RV intraventricular dyssynchrony was calculated as the standard deviation (SD) of the aforementioned corrected time intervals for the six segments of RV, referred to as RV-SD6, and LV intraventricular dyssynchrony (LV-SD6) was identified in the same way. We calculated LV/RV interventricular dyssynchrony in two different ways because there were no standard definitions or measurements for 2D-STE identified interventricular dyssynchrony: (I) LSR-SD9: the SD of corrected time intervals of nine segments that contained six segments of the RV and three segments of the LV free wall; (II) LR-SD6: the SD of corrected time intervals of six segments including the left ventricle and the RV free wall, without the segments of the interventricular septum.
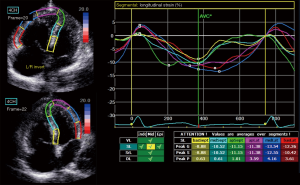
A symptom-limited CPET with a 10 W/min incremental cycle ergometer was performed in 66 patients, and PVO2 and peak respiratory exchange ratio (RER) were measured breath-by-breath (COSMED Quark PFT Ergo, Italy). If the RER was greater than 1.1, we considered the test to be maximal. Medical records were reviewed to obtain other clinical information: age, sex, body mass index (BMI), WHO-FC, laboratory examinations, ECG, six-minute walk distance (6MWD), hemodynamics and targeted agents. Then, a comprehensive risk assessment was performed according to the 2015 ESC Guidelines, and patients were divided into a low-risk group and a moderate-to-high-risk group, which were scored as 1 and 2 points, respectively (6). The low-risk classification was considered when a patient met all the following criteria simultaneously: no progression of symptoms or syncope; the absence of clinical signs of right heart failure; WHO-FC I-II; 6MWD >440 m; PVO2 >15 mL/min/kg; N-terminal pro-brain natriuretic peptide (NT-proBNP) <300 pg/mL; no pericardial effusion; RAP <8 mmHg; CI ≥2.5 L/min/m2 and SvO2 >65%. Among the 66 PH patients, 58 patients were treatment-naïve and the intervals between echocardiography and RHC were less than 24 hours. Coincidentally, irrespective of RHC parameters, other clinical data of the other 8 patients did not meet the criteria of low risk; therefore, these patients were not excluded from our study and were classified in the intermediate-to-high-risk group.
Continuous data are described as the mean ± SD, and categorical data are expressed as counts and proportions. Comparisons between groups were performed with one-way ANOVA, while the homogeneity of variance was analyzed by the Levene test. Linear correlation analyses were used to evaluate the correlations between clinical data, echocardiography parameters, 2D-STE characteristics and PVO2, expressed as a Pearson correlation coefficient (r). Multivariate linear regression analyses were performed to identify the independent predictors of PVO2 by a stepwise variable selection method with a significant level for entry of 0.1 and a significant level to remain of 0.05. Sixty-nine variables were included in each linear regression analysis, and the appropriate sample size was supposed to be five-to-ten-times that of the variables; therefore, the sample size in our study was considered acceptable for our purpose. Four models were constructed: Model-1 was limited to clinical data. We subsequently added conventional echocardiographic variables and 2D-STE parameters, including RV/LV intra-/interventricular dyssynchrony and RV-GLS, to clinical data, and Model-2, -3 and -4 were constructed. Details for each model are described by the corresponding adjusted r2, constant, regression coefficient and P value, and the adjusted r2 was used to compare the predictive accuracy. Patients were further divided into three groups according to the tertiles of LV/RV interventricular dyssynchrony—LSR-SD9. In addition, multivariable logistical regression modeling was used to analyze the association between interventricular dyssynchrony and risk assessment, described as odds ratios (ORs) and 95% confidence intervals (CIs). A receiver operator characteristic curve (ROC) was used to evaluate the predictive value of LSR-SD9 and identify the optimal cut-off point for risk assessment.
For 2D-STE measurement, intra- and interobserver variability were assessed for 20 randomly selected patients by the Bland-Altman method, and the results were described as follows: RV-GLS: −0.13%±1.47%, 95% confidence interval (CI): −3.02% to 2.76% and 0.86%±1.29%, 95% CI: −1.67% to 3.40%, respectively; RV-SD6: 1.48±6.37 ms, 95% CI: −10.99 to 13.96 ms and 3.08±7.18 ms, 95% CI: −10.99 to 17.16 ms, respectively; LV-SD6: −0.43±5.02 ms, 95% CI: −10.28 to 9.42 ms and 1.08±5.50 ms, 95% CI: −9.70 to 11.87 ms, respectively; LSR-SD9: 0.88±6.27 ms, 95% CI: −11.40 to 13.18 ms and 3.30±7.87 ms, 95% CI: −12.14 to 18.73 ms, respectively; LR-SD6: 0.73±7.51 ms, 95% CI: −13.99 to 15.46 ms and 1.19±8.69 ms, 95% CI: −15.83 to 18.22 ms, respectively. These findings were similar to those of a previous study (20) and can be considered acceptable for our clinical purpose.
All statistical analyses were performed using SPSS software (version 19.0, IBM), GraphPad Prim software (version 6.01) and MedCalc (version 15.2). All statistical tests were two-sided, and P<0.05 was considered statistically significant.
Results
As described in Table 1, we recruited 66 PcPH patients (19 male and 47 female, 35±13 years) for the present study, and approximately 90% of the population was characterized as II-III (WHO-FC). In addition, linear correlation analyses showed that there were significant but relatively weak correlations between PVO2 and WHO-FC (r=−0.512, P<0.001), NT-proBNP (r=−0.493, P<0.001), blood urea nitrogen (BUN, r=−0.304, P=0.013), oxygen partial pressure (PO2, r=−0.343, P=0.006), E/E' (r=−0.41, P=0.001), and RV-FAC (r=0.369, P=0.002).
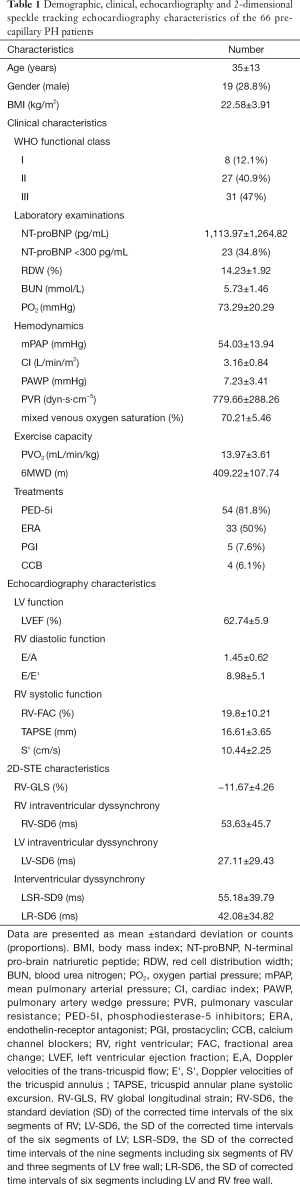
Full table
The first multivariate regression analysis considered clinical variables that significantly correlated with PVO2 (WHO-FC, NT-proBNP, BUN, PO2) and prognostic predictors suggested by previous studies, such as BMI (21) and red blood cell distribution width (RDW) (22,23), while echocardiographic parameters were excluded. Model-1 was constructed (r2=0.423, P<0.001) and identified WHO-FC, NT-proBNP and BMI as independent predictors of PVO2. The second analysis added conventional echocardiographic parameters (E/E', RV-FAC) to the clinical variables and identified WHO-FC, RV-FAC and BMI as independent predictors of PVO2 (Model-2, r2=0.417, P<0.001). Finally, we respectively added RV-GLS, RV-SD6, LV-SD6, LSR-SD9 and LR-SD6 to the conventional echocardiographic and clinical parameters, and identified RV-GLS (Model-3, r2=0.454, P=0.001), RV-SD6 (Model-4, r2=0.474, P<0.001) and LSR-SD9 (Model-5, r2=0.483, P<0.001) as independent predictors of PVO2, and the predictive capability increased. Of all the models constructed in our study, Model-5 including LSR-SD9 had the highest predictive capability for PVO2 (Table 2).
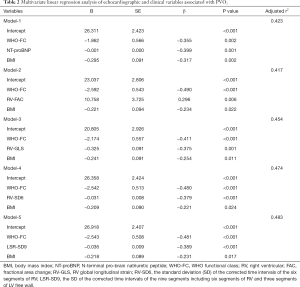
Full table
To further evaluate the robustness of our results, the PH patients were divided into three groups based on the tertiles of LSR-SD9, and there were significant differences in PVO2 and 6MWD among the three groups. As Figure 2 shows, the PVO2 of the three groups in ascending order was 16.11±3.44, 13.99±3.54 and 11.80±2.49 mL/min/kg, respectively (P<0.001); while the 6MWD was 458.64±96.5, 385.05±113.64 and 380.25±96.11 m, respectively (P=0.025). The risk scores of the comprehensive risk assessment according to the 2015 ESC Guidelines are shown in Figure 3 (lower tertile vs. intermediate tertile vs. upper tertile: 1.55±0.51 vs. 1.82±0.4 vs. 1.91±0.29; P<0.001). In other words, the worse the degree of interventricular dyssynchrony, the worse the exercise capacity and the higher the 1-year mortality risk.
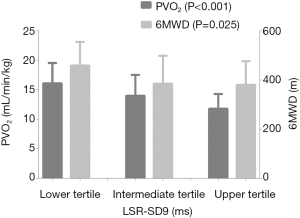
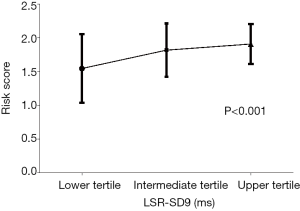
Among the 66 PcPH patients in the present study, 16 patients met the criteria for low-risk classification according to the 2015 ESC guidelines. Logistic regression showed that after adjusting for age, sex and BMI, LSR-SD9 was independently associated with risk assessment (OR 1.027, 95% CI: 1.003–1.052, P=0.03), and the results are shown in Table 3. The ROC curve of LSR-SD9 for the prediction of low risk classification is shown in Figure 4, and the area under the curve was 0.73 (P=0.006). The optimal cut-off point of LSR-SD9 was 27.53 ms, which had 84.0% sensitivity and 62.5% specificity for the prediction of risk classification in PH patients.
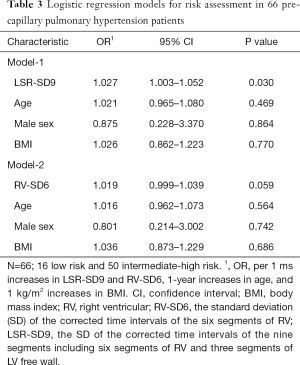
Full table
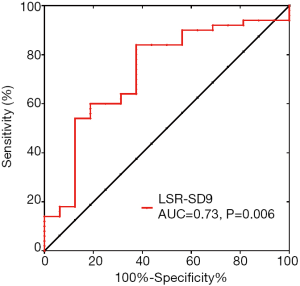
Discussion
The present study, to the best of our knowledge, was the first time to propose the conception of 2D-STE-detected interventricular dyssynchrony and showed that interventricular dyssynchrony contributed to a better evaluation of impaired exercise capacity and disease severity in PcPH patients; compared with RV intraventricular dyssynchrony, the predictive capability of interventricular dyssynchrony tended to be stronger. Additionally, this added predictive value was not observed for LV intraventricular dyssynchrony.
We mainly focused on interventricular dyssynchrony in our study, because LV dysfunction was commonly observed in PH patients but has not previously received sufficient attention, and its mechanisms remain unclear. From the macroscopic point of view, LV dysfunction in PH patients may develop due to a preload-dependent pathway. Kallianos et al. reported a relationship between RV end diastolic volume index (RVEDVI) and septal strain measured by CMR in PH patients, suggesting that ventricular interdependent was a possible mechanism of LV dysfunction before a significant decline of LVEF in this population (16). Additionally, several studies have demonstrated that the septum deformity caused by high pressure of RV may be responsible for LV contractile dysfunction in PAH patients with preserved or mildly depressed LVEF (24,25). At the micro level, Sharma et al. showed that pyruvate dehydrogenase-4 (PDK4) and B-MHC levels expressed by LV changed during RV remodeling induced by hypoxia (26); Lourenço et al. reported an upregulation of endothelin-1 mRNA in the left ventricle in a hypoxia/monocrotaline-induced PAH model, which was also observed in the context of impaired LV function (27). Research by Imai et al. showed some pathological molecular pathways activated in the RV myocardium in a transverse aortic constriction-induced short-term LV pressure-overload model, such as increased levels of brain natriuretic peptide (BNP) mRNA, interleukin-1 beta (IL-1b) and interleukin-6 (IL-6) and increased calcineurin activity, suggesting that RV-LV interventricular interaction might be mediated by inflammatory activity (28). For CTD-PAH patients, LV dyssynchrony may be a direct consequence of primary myocardial involvement, subsequent to the concurrent increases in pulmonary arterial pressures (29,30); and the pathological mechanism underlying LV dyssynchrony may include decreased capillary density, increased apoptosis of endothelial cells, perivascular inflammation, collagen deposition and myocardial fibrosis (31). All these studies suggested that simultaneous LV dysfunction was inevitable in patients with PH as the disease progressed.
PcPH originates as a primary pathology of the pulmonary vasculature (32), which causes a persistently increased RV afterload. RV myocytes are characterized by the longitudinal inflow (proximal) and circumferential outflow (distal) (33), but predominantly oriented longitudinally so that RV contraction primarily results in longitudinal shortening (34). Therefore, RV dyssynchrony based on longitudinal strain measured by 2D-STE corresponded to RV contractile function and was thereby associated with cardiac output (CO) and exercise capacity, as demonstrated in our study and a previous study (11). A possible pathophysiological explanation of this phenomenon is that patients with severe PH often have slower blood flow from the right to the left side of the heart and the coupled LV dyssynchrony further reduces the CO of the left ventricle. Then, this process is gradually accentuated during exercise, which adds to the decrease in both right and LV CO, eventually leading to impaired exercise capacity (35).
The 2015 ESC risk assessment strategy was used to evaluate disease severity in PH patients; however, the assessment was comprehensive and included invasive methods such as RHC. Furthermore, there were no detailed recommendations about management in the context of combinations of different risk stratification parameters. We considered a patient to have low risk only if he or she met all criteria indicating low risk in clinical practice; otherwise, changes in treatment should be considered. Rehman et al. showed that RV global and free-wall longitudinal strain identified by 2D-STE might classify PAH patients according to exercise testing risk stratification cut-offs, but the parameters of intra- and interventricular dyssynchrony were not mentioned. They also found that none of the echocardiographic LV parameters had the same ability, which may be due to their exclusion of all patients with LV dysfunction from their study groups (36). In contrast, research from Nakano indicated that the evaluations of both LV and RV functions were of great importance for PAH and CTEPH patients, but they used standard echocardiography rather than 2D-STE parameters to evaluate the LV and RV functions (37). In the present study, we found that interventricular dyssynchrony may be a noninvasive and convenient indicator for risk stratification of PcPH patients. 2D-STE-detected LV/RV inter- and intraventricular dyssynchrony could be a routine measurement that could contribute to the assessment of severity and therapeutic strategies for PcPH patients in the future.
Notably, compared with RV intraventricular dyssynchrony, the interventricular dyssynchrony described by LSR-SD9 may have a slightly stronger value for predicting PVO2 and risk assessment. In other words, LV dysfunction is inevitable during disease progression, and comprehensive analyses of the left and right heart functions may be superior to the analysis of right heart function alone, even in PH patients who are thought to present with predominantly RV dysfunction. These findings were not surprising, and were supported by a previous study in a similar group of patients (38). Therefore, early identification of biventricular dysfunction in clinical practice may help clinicians assess disease severity and prognosis more accurately and in a timely manner, which further contributes to determining therapeutic strategies and optimizing the time to heart/lung transplantation, ultimately benefiting PH patients.
There were also some limitations in our study. First, as a single center study, the sample size was relatively small, but considering a relatively rare disease was being explored, the sample size was acceptable. Second, although we performed all the echocardiographic measurements according to the guidelines, there was inevitable heterogeneity and variability of the quality of echocardiography and 2D speckle-tracking parameters. To improve the data quality, all of the measurements were performed in strict accordance with the guidelines, and the intra- and interobserver variability were considered acceptable for our clinical purpose. Third, for a few patients in our study, echocardiography and RHC were not performed simultaneously, which was described in the Methods section, which may have had little or no influence on our results. Finally, this was a cross-sectional study; no cause-effect relationships were demonstrated, and regular follow-up will be performed in the future.
Conclusions
In conclusion, interventricular dyssynchrony detected by 2D-STE contributed to a better evaluation of exercise capacity and disease severity in PcPH patients.
Acknowledgments
We are indebted to the patients who participated in this study. We thank the research staff, the Department staff of Ultrasound for their most valuable efforts.
Funding: The present study was supported by the grant of Capital Health Development and Scientific Research Projects (2016–2-4036), CAMS Initiative for Innovative Medical (2016-I2M-3-006) and the Biomedicine and Life Sciences Innovation Cultivation Research Project (Z161100000116052).
Footnote
Conflicts of Interest: All authors have completed the ICMJE uniform disclosure form (available at http://dx.doi.org/10.21037/atm.2020.03.146). The authors have no conflicts of interest to declare.
Ethical Statement: The authors are accountable for all aspects of the work in ensuring that questions related to the accuracy or integrity of any part of the work are appropriately investigated and resolved. The present study was complied with the 1975 Declaration of Helsinki and was approved by the Ethics Committee of Fuwai Hospital (No. 2018-1063). All participants signed their written informed consent.
Open Access Statement: This is an Open Access article distributed in accordance with the Creative Commons Attribution-NonCommercial-NoDerivs 4.0 International License (CC BY-NC-ND 4.0), which permits the non-commercial replication and distribution of the article with the strict proviso that no changes or edits are made and the original work is properly cited (including links to both the formal publication through the relevant DOI and the license). See: https://creativecommons.org/licenses/by-nc-nd/4.0/.
References
- Vonk-Noordegraaf A, Haddad F, Chin KM, et al. Right heart adaptation to pulmonary arterial hypertension: physiology and pathobiology. J Am Coll Cardiol 2013;62:D22-33. [Crossref] [PubMed]
- Kasner M, Westermann D, Steendijk P, et al. Left ventricular dysfunction induced by nonsevere idiopathic pulmonary arterial hypertension: a pressure-volume relationship study. Am J Respir Crit Care Med 2012;186:181-9. [Crossref] [PubMed]
- Saleh S, Liakopoulos OJ, Buckberg GD. The septal motor of biventricular function. Eur J Cardiothorac Surg 2006;29 Suppl 1:S126-38. [Crossref] [PubMed]
- Benza RL, Miller DP, Gomberg-Maitland M, et al. Predicting survival in pulmonary arterial hypertension: insights from the Registry to Evaluate Early and Long-Term Pulmonary Arterial Hypertension Disease Management (REVEAL). Circulation 2010;122:164-72. [Crossref] [PubMed]
- Arena R, Lavie CJ, Milani RV, et al. Cardiopulmonary exercise testing in patients with pulmonary arterial hypertension: an evidence-based review. J Heart Lung Transplant 2010;29:159-73. [Crossref] [PubMed]
- Galiè N, Humbert M, Vachiery JL, et al. 2015 ESC/ERS Guidelines for the diagnosis and treatment of pulmonary hypertension: The Joint Task Force for the Diagnosis and Treatment of Pulmonary Hypertension of the European Society of Cardiology (ESC) and the European Respiratory Society (ERS): Endorsed by: Association for European Paediatric and Congenital Cardiology (AEPC), International Society for Heart and Lung Transplantation (ISHLT). Eur Respir J 2015;46:903-75. [Crossref] [PubMed]
- Wensel R, Opitz CF, Anker SD, et al. Assessment of survival in patients with primary pulmonary hypertension: importance of cardiopulmonary exercise testing. Circulation 2002;106:319-24. [Crossref] [PubMed]
- Blumberg FC, Arzt M, Lange T, et al. Impact of right ventricular reserve on exercise capacity and survival in patients with pulmonary hypertension. Eur J Heart Fail 2013;15:771-5. [Crossref] [PubMed]
- Marcadet DM. Exercise testing: New guidelines. Presse Med 2019;48:1387-92. [Crossref] [PubMed]
- Badagliacca R, Reali M, Poscia R, et al. Right Intraventricular Dyssynchrony in Idiopathic, Heritable, and Anorexigen-Induced Pulmonary Arterial Hypertension: Clinical Impact and Reversibility. JACC Cardiovasc Imaging 2015;8:642-52. [Crossref] [PubMed]
- Badagliacca R, Papa S, Valli G, et al. Right ventricular dyssynchrony and exercise capacity in idiopathic pulmonary arterial hypertension. Eur Respir J 2017. [Crossref] [PubMed]
- Liu BY, Wu WC, Zeng QX, et al. Two-dimensional speckle tracking echocardiography assessed right ventricular function and exercise capacity in pre-capillary pulmonary hypertension. Int J Cardiovasc Imaging 2019;35:1499-508. [Crossref] [PubMed]
- Park JH, Kusunose K, Kwon DH, et al. Relationship between Right Ventricular Longitudinal Strain, Invasive Hemodynamics, and Functional Assessment in Pulmonary Arterial Hypertension. Korean Circ J 2015;45:398-407. [Crossref] [PubMed]
- Motoki H, Borowski AG, Shrestha K, et al. Right ventricular global longitudinal strain provides prognostic value incremental to left ventricular ejection fraction in patients with heart failure. J Am Soc Echocardiogr 2014;27:726-32. [Crossref] [PubMed]
- Cheng XL, Liu BY, Wu WC, et al. Impact of right ventricular dyssynchrony on prognosis of patients with idiopathic pulmonary arterial hypertension. Pulm Circ 2019;9:2045894019883609. [Crossref] [PubMed]
- Kallianos K, Brooks GC, Mukai K, et al. Cardiac Magnetic Resonance Evaluation of Left Ventricular Myocardial Strain in Pulmonary Hypertension. Acad Radiol 2018;25:129-35. [Crossref] [PubMed]
- Liu BY, Wu WC, Zeng QX, et al. EXPRESS: Left ventricular early diastolic strain rate detected by two-dimensional speckle tracking echocardiography and disease severity in pre-capillary pulmonary hypertension. Pulm Circ 2019. [Epub ahead of print]. [Crossref] [PubMed]
- Rudski LG, Lai WW, Afilalo J, et al. Guidelines for the echocardiographic assessment of the right heart in adults: a report from the American Society of Echocardiography endorsed by the European Association of Echocardiography, a registered branch of the European Society of Cardiology, and the Canadian Society of Echocardiography. J Am Soc Echocardiogr 2010;23:685-713. [Crossref] [PubMed]
- Bazett HC. An analysis of the time-relations of electrocardiograms. Heart 1920.353-70.
- Kalogeropoulos AP, Georgiopoulou VV, Howell S, et al. Evaluation of right intraventricular dyssynchrony by two-dimensional strain echocardiography in patients with pulmonary arterial hypertension. J Am Soc Echocardiogr 2008;21:1028-34. [Crossref] [PubMed]
- Hu EC, He JG, Liu ZH, et al. Survival advantages of excess body mass index in patients with idiopathic pulmonary arterial hypertension. Acta Cardiol 2014;69:673-8. [Crossref] [PubMed]
- Abul Y, Ozsu S, Korkmaz A, et al. Red cell distribution width: a new predictor for chronic thromboembolic pulmonary hypertension after pulmonary embolism. Chron Respir Dis 2014;11:73-81. [Crossref] [PubMed]
- Rhodes CJ, Wharton J, Howard LS, et al. Red cell distribution width outperforms other potential circulating biomarkers in predicting survival in idiopathic pulmonary arterial hypertension. Heart 2011;97:1054-60. [Crossref] [PubMed]
- Puwanant S, Park M, Popovic ZB, et al. Ventricular geometry, strain, and rotational mechanics in pulmonary hypertension. Circulation 2010;121:259-66. [Crossref] [PubMed]
- Hardegree EL, Sachdev A, Fenstad ER, et al. Impaired left ventricular mechanics in pulmonary arterial hypertension: identification of a cohort at high risk. Circ Heart Fail 2013;6:748-55. [Crossref] [PubMed]
- Sharma S, Taegtmeyer H, Adrogue J, et al. Dynamic changes of gene expression in hypoxia-induced right ventricular hypertrophy. Am J Physiol Heart Circ Physiol 2004;286:H1185-92. [Crossref] [PubMed]
- Lourenço AP, Roncon-Albuquerque R Jr, Brás-Silva C, et al. Myocardial dysfunction and neurohumoral activation without remodeling in left ventricle of monocrotaline-induced pulmonary hypertensive rats. Am J Physiol Heart Circ Physiol 2006;291:H1587-94. [Crossref] [PubMed]
- Imai Y, Kariya T, Iwakiri M, et al. Sildenafil ameliorates right ventricular early molecular derangement during left ventricular pressure overload. Plos One 2018;13:e0195528. [Crossref] [PubMed]
- Guerra F, Stronati G, Fischietti C, et al. Global longitudinal strain measured by speckle tracking identifies subclinical heart involvement in patients with systemic sclerosis. Eur J Prev Cardiol 2018;25:1598-606. [Crossref] [PubMed]
- Fowler RM, Maiorana AJ, Jenkins SC, et al. A comparison of the acute haemodynamic response to aerobic and resistance exercise in subjects with exercise-induced pulmonary arterial hypertension. Eur J Prev Cardiol 2013;20:605-12. [Crossref] [PubMed]
- Venalis P, Kumanovics G, Schulze-Koops H, et al. Cardiomyopathy in murine models of systemic sclerosis. Arthritis Rheumatol 2015;67:508-16. [Crossref] [PubMed]
- Simonneau G, Robbins IM, Beghetti M, et al. Updated clinical classification of pulmonary hypertension. J Am Coll Cardiol 2009;54:S43-54. [Crossref] [PubMed]
- Lang RM, Badano LP, Tsang W, et al. EAE/ASE recommendations for image acquisition and display using three-dimensional echocardiography. Eur Heart J Cardiovasc Imaging 2012;13:1-46. [Crossref] [PubMed]
- Ho SY, Nihoyannopoulos P. Anatomy, echocardiography, and normal right ventricular dimensions. Heart 2006;92 Suppl 1:i2-13. [Crossref] [PubMed]
- Popovic D, Arena R, Guazzi M.. A flattening oxygen consumption trajectory phenotypes disease severity and poor prognosis in patients with heart failure with reduced, mid-range, and preserved ejection fraction. Eur J Heart Fail 2018;20:1115-24. [Crossref] [PubMed]
- Rehman MB, Garcia R, Christiaens L, et al. Power of resting echocardiographic measurements to classify pulmonary hypertension patients according to European society of cardiology exercise testing risk stratification cut-offs. Int J Cardiol 2018;257:291-7. [Crossref] [PubMed]
- Nakano Y, Okumura N, Adachi S, et al. Left ventricular end-diastolic dimension and septal e' are predictors of cardiac index at rest, while tricuspid annular plane systolic excursion is a predictor of peak oxygen uptake in patients with pulmonary hypertension. Heart Vessels 2018;33:521-8. [Crossref] [PubMed]
- Henein MY, Gronlund C, Tossavainen E, et al. Right and left heart dysfunction predict mortality in pulmonary hypertension. Clin Physiol Funct Imaging 2017;37:45-51. [Crossref] [PubMed]