The JAK2/STAT3 pathway is involved in dexmedetomidine-induced myocardial protection in rats undergoing cardiopulmonary bypass
Introduction
Cardiopulmonary bypass (CPB) is an essential technique in cardiovascular surgery. However, during CPB, the myocardium may be harmed by cardiac ischemia-reperfusion injury, oxidative stress injury, and CPB-related systemic inflammatory response (1). Severe myocardial injury after cardiovascular surgery results in heart failure, slowing the recovery of patients and increasing hospitalization costs. Several attempts have been made to develop procedures for myocardial protection against CPB-related injury in cardiovascular surgery, such as remote ischemic preconditioning (2), modified cardioplegia (3), and drug intervention (4).
Dexmedetomidine, an α2 adrenoreceptor agonist, is extensively applied in clinics. It exhibits a sedative effect and has proven organ-protective effects (5). A previous study has shown that dexmedetomidine protects the myocardium from ischemia-reperfusion injury via decreasing troponin I and creatine kinase-MB (CK-MB) (6). Interestingly, Bulow et al. found that dexmedetomidine exerts anti-inflammatory effects by reducing interleukin (IL)-1, IL-6, TNF-α, and INF-γ levels in plasma after mini-CPB (7). The latter finding suggests that inflammatory factors are involved in myocardial injury related to CPB.
Janus kinase (JAK) is a member of the protein tyrosine kinase family. It participates in cell physiological activities including growth, proliferation, differentiation, and embryonic development. Activated JAK triggers phosphorylation of signal transducer and activator of transcription (STAT). Phosphorylated STAT enters the nucleus via the Ran pathway, activating or inhibiting the transcription of different target genes (8). Several studies have demonstrated that inhibiting the JAK2-STAT3 signaling pathway reduces inflammation and apoptosis (9). Therefore, we hypothesized that the JAK2-STAT3 signaling pathway could play the same role in CPB-related myocardial injury.
In this study, we investigated the effect of different dexmedetomidine doses on myocardial injury caused by CPB in rats, and explored the role of the JAK2-STAT3 pathway in dexmedetomidine effects.
Methods
Rat grouping and treatments
Forty-eight healthy adult male Sprague-Dawley rats, supplied by the Guangxi Medical University Laboratory Animal Center (No. SCXK GUI 2004-0002), were randomly divided into 6 experimental groups: sham, CPB, L, H, AG490, and dimethyl sulfoxide (DMSO). Rats in the sham group were not subjected to a CPB procedure, while rats in the other 5 groups underwent a 2-h CPB. Rats in groups L and H received 2.5 and 5 µg/kg loading doses of dexmedetomidine (Hengrui Medicine, Lianyungang, Jiangsu, China), respectively, by intravenous infusion 15 min before CPB. The same dexmedetomidine doses were maintained during CPB. Rats in the AG490 group received 10 mg/kg of the JAK2 inhibitor, AG490 (Selleck Chemicals, Dallas, TX, USA), 30 min before anesthesia [10 mg of AG490 were diluted with 0.68 ml of dimethyl sulfoxide (DMSO) and then diluted with physiological saline]. Rats in the DMSO group received 0.68 mL/kg DMSO (Solarbio Life Sciences, Beijing, China) in physiological saline.
Preparation of the CPB model
Forty-eight rats received general anesthesia by intraperitoneal injection of pentobarbital sodium at the dose of 65 mg/kg. Mechanical ventilation was applied to maintain breathing. The CPB model was established according to a previously described protocol (10). Blood gas analyses were carried out at the following time points: prior to CPB (t0), 1 h after CPB (t1), and 2 h after CPB (t2); the parameters were maintained in the normal range. The mean arterial pressure (MAP) of each rat was stabilized at 75–100 mmHg and the hematocrit (HCT) was kept at 20–25%. To maintain body temperature at 36.5±1.0 °C, rats were irradiated with heat lamps. The oxygen concentration was maintained between 50% and 100%. The experiment procedure lasted for 2 h.
Specimen collection and management
Venous blood specimens (n=8) were collected at t0, t1, and t2, and were centrifuged at 1,000 g for 15 min. The plasma supernatants were collected and stored at −20 °C before measurement of IL-6 and IL-10 plasma concentrations by enzyme-linked immunosorbent assay (ELISA).
Rats in all groups were sacrificed after CPB, and myocardial tissues from the left ventricular apex were collected (n=8). Part of the myocardial tissues were stored at −80 °C for western blot analysis, while other parts were stored in RNA-preserving liquid at −80 °C. The rest were fixed with 4% refrigerated paraformaldehyde (<24 h), dehydrated by ethanol, cleared in xylene, embedded in paraffin wax, and then cut into 4-µm coronal sections for terminal deoxynucleotidyl transferase dUTP nick end labeling (TUNEL) and immunohistochemistry assays.
ELISA
The plasma concentrations of IL-6 and IL-10 were analyzed using commercially available ELISA kits (for IL-6: Cusabio biotech, Wuhan, China; for IL-10: Thermo Fisher Scientific, Waltham, MA, USA). The determinations were performed according to the manufacturers’ instructions.
TUNEL assay
To assess apoptosis in myocardial cells, the sections of myocardial tissues, prepared as previously described, were analyzed by using a TUNEL kit (Roche Life Science, Basel, Switzerland), as per instructions. The stained tissues were examined by an optical microscope. TUNEL-positive cells were counted, and the apoptosis rate was quantified by the apoptosis index.
Reverse transcription quantitative polymerase chain reaction (RT-qPCR)
Stored myocardial tissues were homogenized in microRNA (miRNA) Miniprep Kit (Axygen®, Corning Life Science, New York, NY, USA), and total RNA was extracted according to the protocol. A reverse transcription kit (Takara Biomedical Technology, Dalian, Liaoning, China) was used to synthetize cDNA. Polymerase chain reaction (PCR) amplification was performed with an Agilent-Stratagene Mx3000P Q-PCR System (Agilent Technologies Inc, Santa Clara County, CA, USA). The glyceraldehyde phosphate dehydrogenase (GAPDH) gene was used as the reference gene. The primer sequences were as follows: GAPDH forward, 5'-CATGGTCTACATGTTCCAGT-3'; GAPDH reverse, 5'-GGCTAAGCAGTTGGTGGTGC-3'; JAK2 forward, 5'-AGCTCCTCTCCTTGACGACT-3'; JAK2 reverse, 5'-GCACGCACTTCGGTAAGAAC-3'; STAT3 forward, 5'-CAGCAATACCATTGACCTGCC-3'; STAT3 reverse, 5'-TTTGGCTGCTTAAGGGGTGG-3'. PCR was performed under the following conditions: incubation at 95 °C for 10 min, followed by 40 cycles of 95 °C for 15 sec and 60 °C for 1 min, in 20 µL of reaction mixture. The products were visualized in 2% agarose gels, which were stained with GoldView I Nuclear Staining Dyes (Solarbio Life Sciences).
Western blot
The JAK2, STAT3, pJAK2, pSTAT3, and cleaved caspase-3 proteins of myocardial tissue were extracted as per kit indications. The protein concentration was quantified. Western blot analysis was performed as previously described (11). Primary antibodies against the following antigens were used: JAK2 (1:1,000, AB_2128522; Cell Signaling Technology, Danvers, MA, USA), STAT3 (1:1,000, AB_331269; Cell Signaling Technology), phospho-JAK2 (phosphor-Y1007 + Y1008, 1:1,000, AB_775808; Abcam, Cambridge, UK), phospho-STAT3 (Tyr705, 1:1,000, AB_1658549; Abcam), β-actin (1:3,000, Bioworld Technology, Minneapolis, MN, USA). The secondary antibodies were horseradish peroxidase-conjugated goat anti-rabbit/mouse IgG (1:10,000, Cell Signaling Technology). The membranes were detected with an Odyssey two-color infrared scanner (LI-Cor Biosciences, Lincoln, NE, USA).
Immunohistochemistry
The protein expression of pJAK2 and pSTAT3 was determined by immunohistochemistry following a previously described protocol (12). Tissue sections were incubated with rabbit monoclonal anti-pJAK2 (1:200) or anti-pSTAT3 (1:100) antibodies.
Statistical analysis
Data were expressed as means ± SEM. All statistical analyses were performed with the statistical software SPSS 16.0 (SPSS Inc, Chicago, IL, USA). Multiple comparisons were tested by one-way analysis of variance. P values<0.05 were considered statistically significant.
Results
All rats survived during the experiment. The MAP, temperature, and blood gas parameters of each rat remained stable, and there was no significant difference among all groups (P>0.05).
Dexmedetomidine reduced IL-6 levels in plasma
The plasma levels of IL-6 and IL-10 were significantly higher at t1 and t2, compared to those at t0, in CPB, DMSO, L, and H rats (P<0.05). At t1 and t2, levels of both cytokines were significantly higher in the CPB group than in the sham group (P<0.05). The levels of IL-6 were significantly decreased at t2 in the AG490, L, and H groups compared to those in the CPB group (P<0.05). However, IL-10 levels did not change significantly between these groups (P>0.05). Moreover, plasma levels of IL-6 and IL-10 at t1 and t2 were similar in rats from the L and H groups (P>0.05, Figure 1).
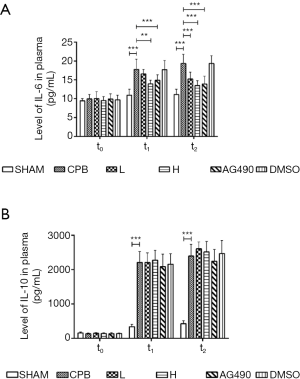
Apoptosis of myocardial cells
After TUNEL staining, few apoptotic nuclei could be observed in the myocardial tissue of sham, AG490, L, and H rats. On the contrary, numerous apoptotic nuclei appeared in the myocardial tissue of CPB and DMSO rats.
The apoptotic rate of myocardial cells was higher in the CPB group compared to that in the sham group (P<0.05). The apoptosis rate was decreased in the AG490, L, and H groups compared to that in the CPB group (P<0.05). Furthermore, the apoptosis rate of myocardial cells in H rats was significantly reduced compared to that in L rats (P<0.05, Figure 2).
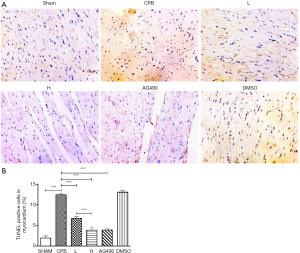
Dexmedetomidine reduced the level of JAK2 and STAT3 mRNA in myocardial tissue
JAK2 and STAT3 mRNA levels were significantly increased in the CPB group compared to those in the sham group (P<0.05). JAK2 expression was significantly reduced in AG490, L, and H rats, and STAT3 mRNA level was significantly decreased in AG490 and H rats compared to that in the CPB group. H rats exhibited a significantly lower level of STAT3 mRNA compared to L rats (P<0.05). Expression of JAK2 mRNA did not show significant difference between the L and H groups (P>0.05, Figure 3).
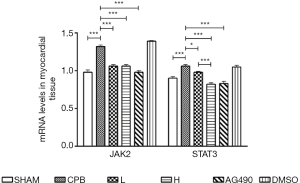
Dexmedetomidine reduced the expression of pJAK2 and pSTAT3 proteins
Western blotting revealed that the level of phosphorylated JAK2 and STAT3 (pJAK2 and pSTAT3) proteins was significantly increased in the CPB group compared to those in the sham group (P<0.05). pJAK2 was significantly downregulated in AG490, L, and H rats (P<0.05), and pSTAT3 was significantly decreased in the AG490 and H groups, compared to that in the CPB group (P<0.05). pJAK2 was significantly decreased in H rats compared that in L rats, (P<0.05), whereas there was no difference in pSTAT3 expression between H and L rats (P>0.05, Figure 4).
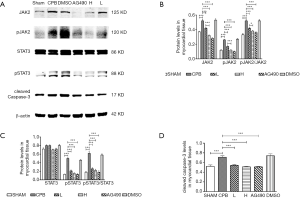
Immunohistochemistry showed the presence of a high number of brown nuclei in the myocardial tissues of CPB and DMSO rats, which was indicative of high pJAK2 and pSTAT3 expression. On the other hand, few brown nuclei were observed in rats of the Sham, AG490, L, and H groups. CPB rats exhibited a higher expression of pJAK2 and pSTAT3 compared to rats in the sham group, AG490, L and H rats (P<0.05). In H rats, pJAK2 and pSTAT3 expression was significantly lower than that in L rats (P<0.05, Figure 5).
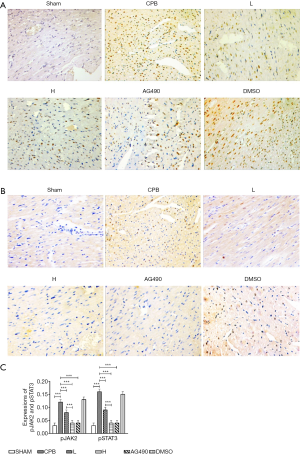
Dexmedetomidine reduced the expression of cleaved caspase-3 protein
To verify the results of the TUNEL assay, cleaved caspase-3 protein expression was assessed. The CPB group exhibited a dramatic increase in the expression of cleaved caspase-3, as compared to the sham group (P<0.05). Moreover, compared to the sham group, cleaved caspase-3 was significantly downregulated in the AG490, L, and H groups (P<0.05). Caspase-3 was expressed at comparable levels in H and L rats (P>0.05, Figure 4).
AG490 decreased pJAK2 and pSTAT3 expression and attenuated myocardial apoptosis
To clarify the role of the JAK2-STAT3 signaling pathway in CPB-induced myocardial apoptosis, the effects of AG490 on apoptosis and on the expression of the relevant signaling proteins, were examined. The TUNEL assay revealed that treatment with AG490 was associated with a reduced number of apoptotic nuclei and a lower apoptotic rate compared to CPB rats. Furthermore, AG490 treatment downregulated JAK2 and STAT3 at both mRNA and protein levels, and reduced the expression of pJAK2, pSTAT3, and cleaved caspase-3 proteins, which indicated that CPB-induced myocardial apoptosis was related to the JAK2-STAT3 signaling pathway (Figures 2-5).
Discussion
Dexmedetomidine produces sedation and analgesia by activating α2 adrenergic receptors in the brain and spinal cord. It has been demonstrated that dexmedetomidine can protect the myocardium by reducing inflammatory cytokines following CPB-related ischemic-reperfusion injury (6).
In this study, CPB was applied for 2 h, based on the average duration of extracorporeal circulation in cardiovascular surgery, as reported in clinical data from several medical institutions (13).
According to the dose conversion between rats and humans (14), 2.5 µg/(kg·h) and 5 µg/(kg·h) dexmedetomidine dosages in rats are equivalent to the clinical dosages of 0.3 µg/(kg·h) and 0.7 µg/(kg·h) in humans, respectively (15,16).
Caspase-3 is a member of the cysteine aspartic acid-specific protease (caspase) family, which plays an important role in the executive phase of cell apoptosis. It has been proven that increased apoptosis is related to the enhanced expression of active caspase-3 (cleaved caspase-3) (17,18). In this study, the level of cleaved caspase-3 protein was increased in all groups undergoing CPB, as compared to sham rats. However, dexmedetomidine administration resulted in a reduction of cleaved caspase-3 level.
CPB induces systemic inflammatory responses and causes injuries of various organs (19). It has been reported that the plasma levels of the inflammatory cytokines IL-6 and IL-10 are increased after CPB, which triggers sequential inflammatory responses (20). IL-6 and IL-10 increase in the early stages of CPB-induced inflammation (21). Therefore, we chose IL-6 and IL-10 as indicators. We found that the plasma levels of IL-6 and IL-10 were increased in rats after CPB. Moreover, the level of IL-6 was decreased in the groups treated with dexmedetomidine, demonstrating that systemic inflammatory responses were induced by CPB and attenuated by dexmedetomidine. These results were consistent with a previous report (7).
IL-6 is an important pro-inflammatory cytokine that activates intracellular signaling pathways (22). JAK2 is a subtype of nonreceptor tyrosine kinase that transduces cytokine-mediated signals via the JAK/STAT pathway. After being activated by IL-6, JAK2 triggers STAT3 phosphorylation. Activated STAT3 transmits signals that result in increased expression of specific target genes. STAT3 is involved in inflammation, endotheliocyte differentiation, angiogenesis, and apoptosis (23). Previous studies (24) have reported that the activation of JAK2/STAT3 pathway protects organs from ischemia-reperfusion injury by inhibiting apoptosis. According to the study of Kim et al. (25), sevoflurane protects the brain by activating the JAK2/STAT3 signaling pathway and reducing neuronal apoptosis in rats undergoing transient cerebral ischemia. On the contrary, recent studies support the view that the inhibition of the JAK2/STAT3 pathway could result in protective effects. In particular, Jia et al. reported that, in rats undergoing autologous orthotopic liver transplantation, propofol attenuates brain ischemia-reperfusion injury by downregulating JAK2 and STAT3 expression (26). Dexmedetomidine was reported to protect the kidney from ischemia-reperfusion injury by inhibition of the JAK/STAT pathway (27). Moreover, triptolide intrathecal injection in the rat model of neuropathic pain was found to downregulate IL-6 by inhibiting the JAK2/STAT3 pathway, thereby suppressing astrocyte activation and relieving neuropathic pain (28). These discrepancies could be due to different experimental conditions, objects, and injury models.
In this study, we observed that administration of dexmedetomidine reduced the level of the inflammatory cytokine IL-6 and decreased JAK2, STAT3, pJAK2, and pSTAT3 protein expression. Therefore, we hypothesized that dexmedetomidine-induced protective effects depended on the inhibition of the JAK2/STAT3 pathway. To verify this hypothesis, we compared the effects of dexmedetomidine with that of AG490, a well-known and specific JAK2 inhibitor. Interestingly, in similar fashion to AG490, dexmedetomidine affected the expression of the proteins JAK2, STAT3, pJAK2, and pSTAT3. Therefore, we concluded that dexmedetomidine reduced myocardial injury by inhibiting the JAK2-STAT3 signaling pathway.
Conclusions
Dexmedetomidine attenuated CPB-related myocardial injury by reducing inflammatory reactions and myocardial apoptosis.
Acknowledgments
Funding: This work was funded by the National Natural Science Foundation of China (No. 81373498 and No. 81060277), the Science Study and Technology Development Program of Guangxi (No. 1355005-4-2), the Natural Science Foundation of Guangxi (2015GXNSFBA139139), the Youth Science Foundation of Guangxi Medical University (GXMUYSF2014041) and the Department of Education Scientific Research Subject of Guangxi (KY2016YB079).
Footnote
Conflicts of Interest: All authors have completed the ICMJE uniform disclosure form (available at http://dx.doi.org/10.21037/atm.2020.03.67). The authors have no conflicts of interest to declare.
Ethical Statement: The authors are accountable for all aspects of the work in ensuring that questions related to the accuracy or integrity of any part of the work are appropriately investigated and resolved. All the experiments in this study were performed according to the ethical review committee approval (2016[KY-E-002]). All applicable international, national, and/or institutional guidelines for the care and use of animals were followed.
Open Access Statement: This is an Open Access article distributed in accordance with the Creative Commons Attribution-NonCommercial-NoDerivs 4.0 International License (CC BY-NC-ND 4.0), which permits the non-commercial replication and distribution of the article with the strict proviso that no changes or edits are made and the original work is properly cited (including links to both the formal publication through the relevant DOI and the license). See: https://creativecommons.org/licenses/by-nc-nd/4.0/.
References
- Bignami E, Saglietti F, Di Lullo A. Mechanical ventilation management during cardiothoracic surgery: an open challenge. Ann Transl Med 2018;6:380. [Crossref] [PubMed]
- Fiorentino F, Angelini GD, Suleiman MS, et al. Investigating the effect of remote ischaemic preconditioning on biomarkers of stress and injury-related signalling in patients having isolated coronary artery bypass grafting or aortic valve replacement using cardiopulmonary bypass: study protocol for a randomized controlled trial. Trials 2015;16:181. [Crossref] [PubMed]
- Huang H, Zhou C, Liu J, et al. Adding Emulsified Isoflurane to Cardioplegia Solution Produces Cardiac Protection in a Dog Cardiopulmonary Bypass Model. Sci Rep 2016;6:23572. [Crossref] [PubMed]
- Liu X, Shao F, Yang L, et al. A pilot study of perioperative esmolol for myocardial protection during on-pump cardiac surgery. Exp Ther Med 2016;12:2990-6. [Crossref] [PubMed]
- Cheng XY, Gu XY, Gao Q, et al. Effects of dexmedetomidine postconditioning on myocardial ischemia and the role of the PI3K/Akt-dependent signaling pathway in reperfusion injury. Mol Med Rep 2016;14:797-803. [Crossref] [PubMed]
- Chen S, Hua F, Lu J, et al. Effect of dexmedetomidine on myocardial ischemia-reperfusion injury. Int J Clin Exp Med 2015;8:21166-72. [PubMed]
- Bulow NM, Colpo E, Pereira RP, et al. Dexmedetomidine decreases the inflammatory response to myocardial surgery under mini-cardiopulmonary bypass. Braz J Med Biol Res 2016;49:e4646. [Crossref] [PubMed]
- Rawlings JS, Rosler KM, Harrison DA. The JAK/STAT signaling pathway. J Cell Sci 2004;117:1281-3. [Crossref] [PubMed]
- Zhang Y, Liu J, Yang B, et al. Ginkgo biloba Extract Inhibits Astrocytic Lipocalin-2 Expression and Alleviates Neuroinflammatory Injury via the JAK2/STAT3 Pathway After Ischemic Brain Stroke. Front Pharmacol 2018;9:518. [Crossref] [PubMed]
- Kim J, Yin T, Yin M, et al. Examination of physiological function and biochemical disorders in a rat model of prolonged asphyxia-induced cardiac arrest followed by cardio pulmonary bypass resuscitation. PLoS One 2014;9:e112012. [Crossref] [PubMed]
- Lv J, Wei Y, Chen Y, et al. Dexmedetomidine attenuates propofol-induce neuroapoptosis partly via the activation of the PI3k/Akt/GSK3beta pathway in the hippocampus of neonatal rats. Environ Toxicol Pharmacol 2017;52:121-8. [Crossref] [PubMed]
- Liu X, Zhang X, Zhang J, et al. Diosmin protects against cerebral ischemia/reperfusion injury through activating JAK2/STAT3 signal pathway in mice. Neuroscience 2014;268:318-27. [Crossref] [PubMed]
- Matata BM, Scawn N, Morgan M, et al. A single-center randomized trial of intraoperative zero-balanced ultrafiltration during cardiopulmonary bypass for patients with impaired kidney function undergoing cardiac surgery. J Cardiothorac Vasc Anesth 2015;29:1236-47. [Crossref] [PubMed]
- Reagan-Shaw S, Nihal M, Ahmad N. Dose translation from animal to human studies revisited. FASEB J 2008;22:659-61. [Crossref] [PubMed]
- Pan W, Lin L, Zhang N, et al. Neuroprotective effects of dexmedetomidine against hypoxia-induced nervous system injury are related to inhibition of NF-kappaB/COX-2 pathways. Cell Mol Neurobiol 2016;36:1179-88. [Crossref] [PubMed]
- Zhu YJ, Peng K, Meng XW, et al. Attenuation of neuroinflammation by dexmedetomidine is associated with activation of a cholinergic anti-inflammatory pathway in a rat tibial fracture model. Brain Res 2016;1644:1-8. [Crossref] [PubMed]
- Watanabe J, Nakamachi T, Ohtaki H, et al. Low dose of methylmercury (MeHg) exposure induces caspase mediated-apoptosis in cultured neural progenitor cells. J Toxicol Sci 2013;38:931-5. [Crossref] [PubMed]
- Yi F, Ni W, Liu W, et al. Expression and biological role of CIP2A in human astrocytoma. Mol Med Rep 2013;7:1376-80. [Crossref] [PubMed]
- Baranowska K, Juszczyk G, Dmitruk I, et al. Risk factors of neurological complications in cardiac surgery. Kardiol Pol 2012;70:811-8. [PubMed]
- Jongman RM, Zijlstra JG, Kok WF, et al. Off-pump CABG surgery reduces systemic inflammation compared with on-pump surgery but does not change systemic endothelial responses: a prospective randomized study. Shock 2014;42:121-8. [Crossref] [PubMed]
- Sayed S, Idriss NK, Sayyedf HG, et al. Effects of propofol and isoflurane on haemodynamics and the inflammatory response in cardiopulmonary bypass surgery. Br J Biomed Sci 2015;72:93-101. [Crossref] [PubMed]
- Steinbrenner H, Bilgic E, Pinto A, et al. Selenium pretreatment for mitigation of ischemia/reperfusion injury in cardiovascular surgery: Influence on acute organ damage and inflammatory response. Inflammation 2016;39:1363-76. [Crossref] [PubMed]
- Owais K, Huang T, Mahmood F, et al. Cardiopulmonary bypass decreases activation of the signal transducer and activator of transcription 3 (STAT3) Pathway in Diabetic Human Myocardium. Ann Thorac Surg 2015;100:1636-45; discussion 1645. [Crossref] [PubMed]
- Li L, Li H, Li M. Curcumin protects against cerebral ischemia-reperfusion injury by activating JAK2/STAT3 signaling pathway in rats. Int J Clin Exp Med 2015;8:14985-91. [PubMed]
- Kim HC, Kim E, Bae JI, et al. Sevoflurane Postconditioning Reduces Apoptosis by Activating the JAK-STAT Pathway After Transient Global Cerebral Ischemia in Rats. J Neurosurg Anesthesiol 2017;29:37-45. [Crossref] [PubMed]
- Jia L, Wang F, Gu X, et al. Propofol postconditioning attenuates hippocampus ischemia-reperfusion injury via modulating JAK2/STAT3 pathway in rats after autogenous orthotropic liver transplantation. Brain Res 2017;1657:202-7. [Crossref] [PubMed]
- Si Y, Bao H, Han L, et al. Dexmedetomidine protects against renal ischemia and reperfusion injury by inhibiting the JAK/STAT signaling activation. J Transl Med 2013;11:141. [Crossref] [PubMed]
- Tang J, Li ZH, Ge SN, et al. The inhibition of spinal astrocytic JAK2-STAT3 pathway activation correlates with the analgesic effects of triptolide in the rat neuropathic pain model. Evid Based Complement Alternat Med 2012;2012:185167.