Complications associated with mechanical circulatory support
Introduction
Over the last decade there has been a significant increase in the utilisation of mechanical circulatory support (MCS) devices (1). Traditionally, MCS was reserved primarily for patients with end stage heart failure who were deemed candidates for transplantation, with MCS used as a ‘bridge to transplantation’. Nowadays, advanced MCS devices are commonplace in the cardiothoracic intensive care and the indications for their use have broadened significantly including prophylactic use in high risk percutaneous coronary interventions or surgery (2), a salvage therapy as an adjunct to cardiopulmonary resuscitation (3) and as part of the routine management of intractable cardiogenic shock (4).
There are several forms of MCS that are typically classified as either temporary or durable. Temporary MCS devices include the intra-aortic balloon pump (IABP), the Impella (Abiomed), the TandemHeart system (CardiacAssist), veno-arterial extra-corporeal membrane oxygenation (VA-ECMO) and temporary ventricular assist devices (VAD) (Table 1) (5). These devices are typically used in the context of acute, intractable, cardiogenic shock that may be associated with a range of aetiologies including myocardial infarction, post-cardiotomy failure, myocarditis and acute deterioration in patients with end stage cardiac failure (4,5). Durable MCS devices are the implantable left ventricular assist devices (LVAD). These devices are most commonly used as either a bridge to transplantation/candidacy or as destination therapy, in patients with end stage cardiac failure (6). In this review, we will discuss the outcomes and major complications of these different MCS modalities.
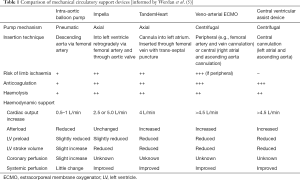
Full table
IABP
The IABP is the commonest form of mechanical support utilised in the cardiothoracic ICU. It was first used in the 1960s, being introduced by Kantrowitz who identified that ‘diastolic augmentation’ improved myocardial oxygenation (7). The IABP comprises a double-lumen balloon catheter that is typically introduced percutaneously into the femoral artery using the Seldinger technique. The haemodynamic effects of the IABP depend on ‘counterpulsation’ resulting from inflation and deflation of the balloon with helium at precise points in the cardiac cycle. Balloon inflation occurs immediately after the end of ventricular systole resulting in augmentation of the diastolic pressure (‘diastolic augmentation’), increasing coronary artery perfusion. Deflation of the balloon is timed to occur immediately prior to ventricular systole, creating a vacuum effect leading to reduced afterload. The effect is to both increase myocardial oxygen supply whilst reducing myocardial oxygen demand (2). There are multiple indications for the use of an IABP including unstable angina, post-cardiotomy cardiogenic shock, refractory ventricular arrhythmias and primary graft dysfunction following cardiac transplantation.
The indications for IABP use are varied and therefore outcomes are difficult to specify. If a patient continues to deteriorate despite IABP therapy, then they will likely become candidates for more advanced form of MCS, such as VA-ECMO. Therefore, long-term survival following IABP therapy is more dependent on the indication and therefore, natural history or treatment of the underlying disease process
Complications
A large registry study, including >17,000 patients treated with an IABP, reported an incidence of complications of 7% with 2.6% described as major, and a device associated mortality rate of 0.5% (8). Complications can be classified as either vascular or non-vascular (Table 2) (2,8,10). Vascular complications are found to be the commonest, and include limb ischaemia, vascular trauma during insertion (dissection or laceration) leading to false aneurysm formation, haematoma or haemorrhage. Distal pulses should be regularly examined and surveillance for the development of compartment syndrome as evidence of limb ischaemia. An incorrectly positioned or incorrectly sized IABP catheter may lead to compromised abdominal visceral perfusion (11). Cerebrovascular accident (CVA) is also reported which may occur due to the balloon catheter being positioned or migrating proximally, leading to obstruction of cerebral blood flow, or rarely due to dislodgement of proximal thrombus which embolises—although this is more likely to lead to visceral or limb ischaemia/infarction (9).
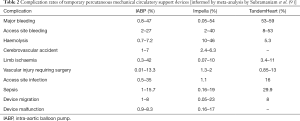
Full table
Non-vascular complications include thrombocytopaenia from platelet deposition on the balloon and due to mechanical disruption of thrombocytes, and haemolysis from disruption of erythrocytes. Sepsis may also occur, particularly in patients with prolonged IABP therapy. Rarely, balloon rupture can occur resulting in gas embolization, although the console will detect this immediately and cease functioning, although immediate removal is required as there is a significant risk of thrombus formation. Patients receiving IABP therapy must remain bed-bound and supine, and therefore are at risk of associated complications.
Impella
The Impella (Abiomed, Danvers, MA, USA) is a non-pulsatile axial flow Archimedes-screw pump designed to propel blood into the ascending aorta in series with the left ventricle (LV) (12). It is a catheter based, miniaturised pump which is introduced retrogradely through the femoral artery (either percutaneously or via surgical cutdown), or via the axillary artery, into the aorta and through the aortic valve. Blood from the LV is drawn into the inlet area near the tip of the device and is delivered into the aortic root through the outlet, thus offloading the LV. The device can provide flow rates of up to 5 L/min (13). The Impella unloads the LV reducing the myocardial oxygen consumption which improves the patients haemodynamics. The Impella is indicated in isolated LV failure as adequate right ventricular function is necessary to maintain LV preload.
The percutaneous nature of the Impella is leading to increasing popularity and utilisation. The most common indications for using Impella are in the treatment of acute myocardial infarction complicated by cardiogenic shock and to facilitate high risk PCI. With increasing experience, the indications broadening and others include: treatment of end stage heart failure with acute decompensation, post-cardiotomy cardiogenic shock, and to facilitate off-pump coronary bypass surgery (12,14).
Complications
The type of complications associated with the Impella are similar to those of the IABP (Table 2). Similarly, vascular complications are common. Limb ischaemia is an important complication requiring continued surveillance. The reported incidence ranges from 0.07–10% (9). These studies suggest that there may be a higher incidence of limb ischaemia with the Impella compared to the IABP. The Impella 5.0 is inserted through a graft sewn to the axillary artery. This may be associated with local vascular and/or graft complications.
The reported incidence of significant bleeding ranges from 0.05% to 54% (9). A meta-analysis comparing Impella with IABP reported a 2.5-fold higher risk of bleeding with the Impella (15). More serious bleeding occurs in the gastrointestinal and neurological systems and is associated with poor outcomes. The risk of bleeding is associated with the requirement for therapeutic anticoagulation for the duration of therapy to minimise the risk of thrombosis. Bleeding may also be associated with thrombocytopenia which results from damage to thrombocytes, however there is limited data on the incidence of thrombocytopenia in patients with the Impella. Thrombocytopenia may also be due to ‘heparin-induced-thrombocytopenia’.
Haemolysis is observed as a common complication with an incidence ranging 7–8% (16), although it is believed that this is likely an underestimate since formal diagnosis is infrequently sought however, longer duration of Impella support is associated with a requirement for multiple blood transfusions suggestive of haemolysis (17). Haemolysis is thought to be caused by shear stress from axial pumping and can lead to renal failure.
CVA, both haemorrhagic and ischaemic, is an important complication of Impella support. The incidence is reported to range 2.4–6.3% (9,18). In patients experiencing an ischaemic CVA, there may be associated risk of haemorrhagic transformation due to the anticoagulation.
Similar to the IABP, there is a risk of access site infection and sepsis, that increases with duration of support. Device migration and malfunction have both rarely been reported with the Impella, more so in the early experience (19). This can lead to injury to the aortic valve, mitral regurgitation secondary to injury to the papillary muscles or chordae, and may even lead to tamponade due to LV perforation (20). The presence of the catheter within the LV can also lead to arrhythmias developing.
TandemHeart
The TandemHeart (TandemLife, Pittsburgh, PA, USA) is an extracorporeal percutaneous axial-flow pump that can provide circulatory support up to 4 L/min. Oxygenated blood is withdrawn from the left atrium which is accessed by an inflow cannula inserted percutaneously through the femoral vein, up the inferior vena cava, into the right atrium and then via trans-septal puncture into the left atrium. Blood is then pumped by a centrifugal pump through an outflow cannula which is inserted into the femoral artery. The TandemHeart works by simultaneously contributing blood flow to the aorta, working in parallel—or ‘tandem’—to the LV. Additionally, offloading blood from the left atrium reduces LV preload, wall stress and therefore oxygen demand. This facilitates increased cardiac output and systemic perfusion pressures (4,21).
The indications for use of the TandemHeart are essentially the same as for the Impella. However, the requirement and technical challenge of transseptal puncture has been a limitation to its widespread uptake and use in comparison to the Impella.
Complications
The type of complications associated with the TandemHeart are similar to those experienced in patients with an IABP or Impella (Table 2) (9). Limb ischaemia is an important complication associated with the femoral arterial cannula and is reported to occur with an incidence between 3.4–11% (22,23). There are no documented amputations associated with the use of the TandemHeart to date.
Major bleeding is more frequently experienced in patients with a TandemHeart. This may be associated with the need for trans-septal puncture, the presence of two sites of percutaneous vascular access, and the need for anticoagulation to reduce the risk of thromboembolism. The incidence is reported between 53–59.8% (22). Gastrointestinal bleeding has been reported in up to 19.7%. The TandemHeart has a high risk of access-site related bleeding, which is likely related to the large sheath required for insertion. Rates range from 8% to 53% (9).
CVA is similarly an important complication in patients supported with TandemHeart. The incidence is reported to be similar to that with the Impella: 2.4–6.3% (9,18). Haemolysis is also an important complication with the TandemHeart and the incidence has been reported to be 5.3% (24). Site associated infection has been reported with an incidence of 16% (25).
More specifically to the TandemHeart is the potential for a significant right-to-left shunt to develop if there is dislodgement of the inflow canula into the right atrium. Some degree of dislodgement was reported with an incidence of 8% in an early study with this device (25). Another specific complication is the risk of air embolism during septal puncture and also the risk of atrial perforation associated with the trans-septal puncture that can lead to tamponade. It was reported with an incidence of 0.85% in early experiences with this device although it is now much more rare (22). A residual atrial septal defect can be present following device removal which can lead to problems in the future (20).
Veno-arterial extracorporeal membrane oxygenation (ECMO)
ECMO is an advanced form of temporary life support to aid respiratory and/or cardiac function. ECMO evolved from cardiopulmonary bypass (CPB) technology and similarly involves diverting venous blood through an extracorporeal circuit, in which gaseous exchange occurs. A venous drainage cannula is inserted into the vena cavae or right atrium, either percutaneously through the femoral or internal jugular vein, or centrally. Blood is returned to the patient through a cannula in either the ascending aorta if inserted centrally, or peripherally in the femoral or subclavian artery which can be performed percutaneously or via surgical cut-down.
VA-ECMO is utilised to support patients with cardiogenic shock that is refractory to maximal therapy (26). It is reserved for patients who are believed to have a reversible cause of their cardiogenic shock or as a bridge to further MCS, such as a ventricular assist device, or cardiac transplantation. VA-ECMO can also be a salvage treatment option in the setting of cardiac arrest with unsuccessful Advanced Life Support (ALS). So-called ‘extracorporeal cardiopulmonary resuscitation’ (eCPR) may be considered especially when cardiac arrest occurs in an intensive care or cardiac catheter laboratory setting where VA-ECMO can be rapidly instituted (27,28).
Complications
The morbidity associated with ECMO is significant, a meta-analysis has summarised the incidence of the commonest complications (Table 3) (29). The most common serious complications are bleeding and thrombosis. Due to blood contact with foreign surfaces, blood stasis in cardiac chambers and disseminated intravascular coagulation there is a significant risk of thrombosis mandating therapeutic anticoagulation. In addition to the requirement for anticoagulation, there is consumption of clotting factors and thrombocytopenia which results in a significant incidence of bleeding—as many as 41.9% of patients require re-exploration. The thrombocytopaenia is related to the injury through the circuit, but in addition as many as 8.3% of patients on VA-ECMO are found to have heparin-induced thrombocytopenia (30). Bleeding can occur in organs including the brain and gastrointestinal tract and occurs at the cannulation sites. The incidence of CVA is significant in patients on VA-ECMO. Reported incidence ranges from 3.3–17.6% for ischaemic CVA and 1.6–5% for haemorrhagic CVA (9,29).
Limb ischaemia is also a major complication of VA-ECMO, related to the large femoral arterial cannula used in peripheral VA-ECMO. As many as 10.3% are reported to develop limb ischaemia and require fasciotomy, with up to 4.7% requiring amputation. The risk of limb ischaemia can be reduced by placement of a small antegrade perfusion catheter into the superficial femoral artery to perfuse the leg distal to the primary cannula (31). Limb ischaemia can also develop following arterial cannula removal due to displacement of clot developing around the cannulae.
Haemolysis is also an issue and is more prevalent in VA-ECMO than with the Impella or TandemHeart, reported to occur in 18% of patients (29). This is associated with the shear forces due to the centrifugal pump and high resistance flow through the oxygenator (32). Similarly, infection is an important complication that should be monitored for and patients treated accordingly.
Acute kidney injury (AKI) occurs in around half of patients supported by ECMO. As such, continuous renal replacement therapy has evolved into an adjunct support in patients on ECMO with the advantage of permitting correction of metabolic and fluid disturbances and may suppress inflammation by counteracting the systemic inflammatory response.
There are some complications unique to VA-ECMO: LV distension and Harlequin syndrome. Left ventricular distension can occur due to the continuous retrograde flow of blood within the ascending aorta, presenting increased afterload against which a failing LV is unable to eject. In the absence of aortic valve opening there is a risk of distension which can lead to the development of pulmonary oedema and even development of intracardiac thrombus (33,34). LV distension can occur with VA-ECMO and insertion of an LV vent is recommended especially in the presence of intracardiac stasis.
Harlequin syndrome is a complication of peripheral VA-ECMO with femoral arterial cannulation and leads to the coronary and cerebral circulations being exposed to deoxygenated blood. This occurs if there is native cardiac output with aortic valve opening. In this situation there will be a ‘mixing zone’ where ejected native anterograde and reinfused retrograde blood meet. This can cause a problem if, in the context of respiratory failure, the blood exiting the LV is deoxygenated. As native cardiac function recovers and left ventricular ejection increases, if the mixing zone is within the descending aorta, the coronary and cerebral circulations may be exposed to deoxygenated blood (31,35,36).
For survivors of ECMO, there has been the suggestion that there is an increase in neurological deficits and respiratory morbidities—and for the neonatal/paediatric population neurodevelopmental deficits and behavioural problems (37). However, more recent data comparing non-ECMO arms of randomised controlled trials have observed no between-group differences for these outcomes. Patients who survive ECMO require prolonged rehabilitation and multidisciplinary follow-up after discharge from hospital (37,38).
VAD
VAD are an alternative to VA-ECMO for patients with refractory cardiogenic shock. A VAD comprises an inflow, a pump and an outflow. Unlike VA-ECMO, there is no oxygenator and a VAD therefore only supports cardiac function. VAD can be used to support most commonly the left ventricle (LVAD), the right ventricle (RV; RVAD) or can be used in a bi-ventricular configuration (BI-VAD) depending on cannula placement. For LV support, the inflow cannula is placed either into the left atrium or ventricle and the outflow cannula placed to return blood to the ascending aorta. For RV support, the inflow cannula is placed into the right atrium or ventricle and the outflow cannula placed in the pulmonary artery.
VAD can be used as a temporary form of MCS, with central placement of the cannula, but also are available as implantable pumps that can be used as longer term support. VAD pump technology has evolved significantly over the last three decades. First generation devices were pneumatic or electrical membrane pumps which generated pulsatile flow. They were bulky and noisy devices with high rates of malfunction. These pumps additionally had problems with haemolysis and thrombus formation due to shear stress, friction with heat generation, turbulent flow, and stasis (39). Now, continuous flow pumps are most commonly used are remarkably smaller and quieter devices.
One commonly used system for temporary support is the Levitronix CentriMag (Abbott Laboratories, Abbott Park, IL, USA). The bearing-less impeller-based pump has the ability to provide flows up to 10 litres/minute with good durability in the medium-term. The pump contains a magnetically levitated impeller that is contact-free without mechanical bearings or seals. It is designed to minimize friction and heat generation, and to reduce shear force on RBCs to preventing haemolysis (40,41).
In terms of implantable LVAD devices the current, third generation, devices most frequently implanted include the HeartWare HVAD (Medtronic, Minneapolis, MN, USA) (42) and the HeartMate 3 (Abbott, St Paul, MN, USA) (43). The HeartWare is a centrifugal pump and the HeartMate 3 has a fully magnetically levitated, self-centering rotor. Both are designed for full intrapericardial implantation obviating the need for creation of a ‘pump pocket’. Although implantation often involves a full sternotomy and CPB, minimally invasive and off-pump implantation techniques have been successfully utilised (44).
Most commonly, VADs are used for LV support, bypassing the LV and offloading it. This leads to reduced preload and myocardial oxygen consumption. The LV can continue to contract, but with significantly reduced work as a result of reduced preload and afterload. Offloading the LV will result in a reduction in the RV afterload and usually improves RV function. RV failure has been reported in 5–44% in patients undergoing LVAD implantation and this is dependent on the baseline function of the RV. With impaired RV function, RV failure can be very difficult to predict preoperatively and can be severe enough requiring mechanical support.
The indications for LVAD therapy are well defined, and similar to VA-ECMO, patients with non-recoverable cardiac failure who are not potential transplant candidates should not be commenced on this therapy (unless it is being used as a destination therapy) (6,45). An LVAD can be used as a bridge to recovery in acute situations in which there is expectation of myocardial recovery—such as would potentially be the case in myocarditis or post-cardiotomy cardiogenic shock for example. They can also be used as a bridge to decision in patients for whom transplant candidacy is unclear at the time of presentation—for example unknown neurological status, whilst further assessments are made. The most common indication for VADs is as a bridge to transplantation/candidacy—both for temporary and durable VADs. However, durable, implantable LVADs are increasingly being utilised as a destination therapy for selected patients in some countries, and is likely to become the commonest indication in the future (46). In patients with severe biventricular failure in need of mechanical support, total artificial heart is used in specialist centres as a bridge to transplant.
Complications
The complications of temporary and implantable continuous flow VADs are similar, having a similar basis, although for patients with long-term implantable devices there is longer exposure to the device and on-going risk of these complications for the duration of therapy. The incidence of complications following temporary VAD support have been summarised in a recent meta-analysis (Table 4) (40). For implanted LVADs the Interagency Registry for Mechanically Assisted Circulatory Support (INTERMACS) regularly report registry outcomes, and the most recent report summarises the incidence of adverse events for almost 18,000 patients (Table 5) (46). The incidence of complications has reduced significantly with evolution of the devices, leading to the increased utilisation of LVAD as a destination therapy. Ongoing device development aims to further reduce the incidence of associated complications. Below, the common complications of continuous-flow VADs are discussed.
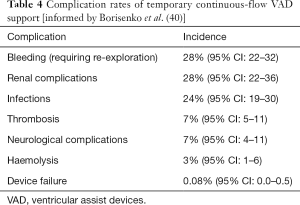
Full table
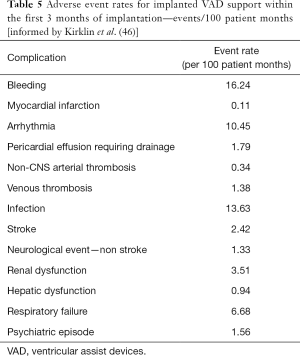
Full table
Bleeding
Bleeding is an important complication in patients with VADs. It can occur peri-operatively or later after implantation. There are several reasons why patients are predisposed to bleed:
- Patients require therapeutic anticoagulation due to the interaction of blood and non-biological surface which is a strong activator of the coagulation cascade, and may also be treated with anti-platelet medication.
- Patients in cardiogenic shock, or who can develop RV impairment post-procedure can develop hepatic congestion contributing to a coagulopathy.
Patients almost universally develop acquired von Willebrand disease. It is believed that the shear stress attributed to the continuous flow devices leads to an unfolding of von Willebrand factor (vWf) which is then targeted for proteolysis. vWf is an important mediator of platelet aggregation (47).
Important bleeding problems for these patients include gastrointestinal bleeding and haemorrhagic CVA. Gastrointestinal bleeding occurs in 20–30%, with up to 40% of these patients experiencing recurrent bleeds which can be a major source of morbidity (48). Gastrointestinal bleeding appears to be a particular problem with continuous-flow devices due to the combination of vWf deficiency and the development of arteriovenous malformations. It is thought that the increased intraluminal pressure and decreased pulsatility lead to distension of the submucosal vessels leading to intestinal mucosal hypoperfusion and angiodysplasia (49).
Thrombosis
Pump thrombosis or development of thrombus within the extracorporeal circuit is a serious complication. Thrombus formation is multifactorial. The non-biological surfaces are a strong activator of the coagulation cascade. As such there is a tight balance between the risk of thrombosis vs. the risk of bleeding when managing patients anticoagulation. Pump thrombosis can manifest clinically as haemolysis and this should be assessed regularly. Thrombosis can result in embolic CVA, peripheral or visceral embolism and heart failure and may require a change of device with significant risk of morbidity and mortality (45,49). The emergence of the newest generation devices has resulted is a remarkable reduction in this complication—occurring in only 1.4% of patients with Heartmate 3 compared to 13.9% with Heartmate II in the Momentum 3 study.
Neurological complications
Neurological complications are devastating and increase significantly mortality, but also can render a patient no longer suitable for transplantation. Neurological complications include transient ischaemic attack and haemorrhagic or ischaemic CVA and are associated with bleeding and thrombotic complications described above.
Infections
Infection is an important source of morbidity in patients with VADs. For patients with temporary circuits, the perioperative risk of infection is present, with the risk of infection at the subcutaneous exit site of the cannulae. For patients with implanted LVADs, the driveline is an important source of infections—with a direct communication between the environment and the implanted pump. This occurs in over a quarter of patients within the first 2 years of support. As such patients should be taught to recognise the early signs of infection, and infections must be treated aggressively to reduce the risk of life-threatening complications. The presence of infections have been associated with development of vasoplegia at the time of transplantation which may be associated with inferior outcomes (50).
Aortic insufficiency
Aortic insufficiency is a problem that affects 11% of patients with implanted LVAD over time (51). The development of aortic insufficiency is thought to be a consequence of the haemodynamics imposed by continuous-flow devices: (I) persistent aortic valve closure due to persistent high pressure leading to structural remodelling of the valve leaflets and commissural fusion; and (II) aortic root dilatation that occurs due to aortic wall remodelling in the media, due to the absence of pulsatility.
Right ventricular failure
Patients with significant LV dysfunction often also have an element of RV impairment. Patients who are treated with an LVAD can subsequently develop RV failure. Patients treated with temporary VADs often have a biventricular configuration (BI-VAD) in order to avoid this complication. For patients having an implanted LVAD, right heart failure complicates up to 40% of patients depending on selection and the definitions used (52). It is believed that this relates to alteration in the mobility and position of the interventricular septum. With an unloaded LV, the septum bows towards the LV and this is thought to worsen tricuspid regurgitation and lead to reduced right ventricular stroke volume (53). Furthermore, the increased LV output may lead to greater strain being placed on the RV due to increased venous return.
Ventricular arrhythmias
In patients with an implanted LVAD the development of ventricular arrhythmias can be a serious complication. It is thought that arrhythmias can develop for a range of reasons, including development of scar tissue at the apical entry point of the device, and secondary to the inflow cannula interacting with the septal and lateral wall endocardium. In many patients, ventricular arrhythmias are present prior to LVAD implantation due to pre-existing myocardial substrate. These have been shown to increase morbidity and mortality in LVAD patients.
Acknowledgments
Funding: None.
Footnote
Provenance and Peer Review: This article was commissioned by the Guest Editor (Kamen Valchanov) for the Series “Perioperative Management of Patients with undergoing Mechanical Circulatory Support” published in Annals of Translational Medicine. The article was sent for external peer review organized by the Guest Editor and the editorial office.
Conflicts of Interest: All authors have completed the ICMJE uniform disclosure form (available at http://dx.doi.org/10.21037/atm.2020.03.152). The series “Perioperative Management of Patients with undergoing Mechanical Circulatory Support” was commissioned by the editorial office without any funding or sponsorship. The authors have no other conflicts of interest to declare.
Ethical Statement: The authors are accountable for all aspects of the work in ensuring that questions related to the accuracy or integrity of any part of the work are appropriately investigated and resolved.
Open Access Statement: This is an Open Access article distributed in accordance with the Creative Commons Attribution-NonCommercial-NoDerivs 4.0 International License (CC BY-NC-ND 4.0), which permits the non-commercial replication and distribution of the article with the strict proviso that no changes or edits are made and the original work is properly cited (including links to both the formal publication through the relevant DOI and the license). See: https://creativecommons.org/licenses/by-nc-nd/4.0/.
References
- Stretch R, Sauer CM, Yuh DD, et al. National trends in the utilization of short-term mechanical circulatory support: incidence, outcomes, and cost analysis. J Am Coll Cardiol 2014;64:1407-15. [Crossref] [PubMed]
- Ali JM, Abu-Omar Y. The intra-aortic balloon pump and other methods of mechanical circulatory support. Surgery 2018;36:68-74.
- Michels G, Wengenmayer T, Hagl C, et al. Recommendations for extracorporeal cardiopulmonary resuscitation (eCPR): consensus statement of DGIIN, DGK, DGTHG, DGfK, DGNI, DGAI, DIVI and GRC. Clin Res Cardiol 2019;108:455-64. [Crossref] [PubMed]
- Hajjar LA, Teboul JL. Mechanical Circulatory Support Devices for Cardiogenic Shock: State of the Art. Critical care 2019;23:76-10. [Crossref] [PubMed]
- Werdan K, Gielen S, Ebelt H, et al. Mechanical circulatory support in cardiogenic shock. Eur Heart J 2014;35:156-67. [Crossref] [PubMed]
- Schramm R, Morshuis M, Schoenbrodt M, et al. Current perspectives on mechanical circulatory support. Eur J Cardiothorac Surg 2019;55:i31-7. [Crossref] [PubMed]
- Kantrowitz A, Tjonneland S, Freed PS, et al. Initial clinical experience with intraaortic balloon pumping in cardiogenic shock. JAMA 1968;203:113-8. [Crossref] [PubMed]
- Ferguson JJ, Cohen M, Freedman RJJ, et al. The current practice of intra-aortic balloon counterpulsation: results from the Benchmark Registry. J Am Coll Cardiol 2001;38:1456-62. [Crossref] [PubMed]
- Subramaniam AV, Barsness GW, Vallabhajosyula S, et al. Complications of Temporary Percutaneous Mechanical Circulatory Support for Cardiogenic Shock: An Appraisal of Contemporary Literature. Cardiol Ther 2019;8:211-28. [Crossref] [PubMed]
- van Nunen LX, Noc M, Kapur NK, et al. Usefulness of Intra-aortic Balloon Pump Counterpulsation. Am J Cardiol 2016;117:469-76. [Crossref] [PubMed]
- Rastan AJ, Tillmann E, Subramanian S, et al. Visceral arterial compromise during intra-aortic balloon counterpulsation therapy. Circulation 2010;122:S92-9. [Crossref] [PubMed]
- Glazier JJ, Kaki A. The Impella Device: Historical Background, Clinical Applications and Future Directions. Int J Angiol 2019;28:118-23. [Crossref] [PubMed]
- Kar B, Basra SS, Shah NR, et al. Percutaneous circulatory support in cardiogenic shock: interventional bridge to recovery. Circulation 2012;125:1809-17. [Crossref] [PubMed]
- Burzotta F, Trani C, Doshi SN, et al. Impella ventricular support in clinical practice: Collaborative viewpoint from a European expert user group. Int J Cardiol 2015;201:684-91. [Crossref] [PubMed]
- Thiele H, Jobs A, Ouweneel DM, et al. Percutaneous short-term active mechanical support devices in cardiogenic shock: a systematic review and collaborative meta-analysis of randomized trials. Eur Heart J 2017;38:3523-31. [Crossref] [PubMed]
- Lauten A, Engström AE, Jung C, et al. Percutaneous left-ventricular support with the Impella-2.5-assist device in acute cardiogenic shock: results of the Impella-EUROSHOCK-registry. Circ Heart Fail 2013;6:23-30. [Crossref] [PubMed]
- Badiye AP, Hernandez GA, Novoa I, et al. Incidence of Hemolysis in Patients with Cardiogenic Shock Treated with Impella Percutaneous Left Ventricular Assist Device. ASAIO J 2016;62:11-4. [Crossref] [PubMed]
- Ouweneel DM, de Brabander J, Karami M, et al. Real-life use of left ventricular circulatory support with Impella in cardiogenic shock after acute myocardial infarction: 12 years AMC experience. Eur Heart J Acute Cardiovasc Care 2019;8:338-49. [Crossref] [PubMed]
- Meyns B, Dens J, Sergeant P, et al. Initial experiences with the Impella device in patients with cardiogenic shock - Impella support for cardiogenic shock. Thorac Cardiovasc Surg 2003;51:312-7. [Crossref] [PubMed]
- Saffarzadeh A, Bonde P. Options for temporary mechanical circulatory support. J Thorac Dis 2015;7:2102-11. [PubMed]
- Chera HH, Nagar M, Chang NL, et al. Overview of Impella and mechanical devices in cardiogenic shock. Expert Rev Med Devices 2018;15:293-9. [Crossref] [PubMed]
- Kar B, Adkins LE, Civitello AB, et al. Clinical experience with the TandemHeart percutaneous ventricular assist device. Tex Heart Inst J 2006;33:111-5. [PubMed]
- Schwartz BG, Ludeman DJ, Mayeda GS, et al. Treating Refractory Cardiogenic Shock With the TandemHeart and Impella Devices: A Single Center Experience. Cardiol Res 2012;3:54-66. [PubMed]
- Burkhoff D, Cohen H, Brunckhorst C, et al. A randomized multicenter clinical study to evaluate the safety and efficacy of the TandemHeart percutaneous ventricular assist device versus conventional therapy with intraaortic balloon pumping for treatment of cardiogenic shock. Am Heart J 2006;152:469.e1-8. [Crossref] [PubMed]
- Tempelhof MW, Klein L, Cotts WG, et al. Clinical experience and patient outcomes associated with the TandemHeart percutaneous transseptal assist device among a heterogeneous patient population. ASAIO J 2011;57:254-61. [Crossref] [PubMed]
- Ali J, Vuylsteke A. Extracorporeal membrane oxygenation: indications, technique and contemporary outcomes. Heart 2019;105:1437-43. [PubMed]
- Huang SC, Wu ET, Wang CC, et al. Eleven years of experience with extracorporeal cardiopulmonary resuscitation for paediatric patients with in-hospital cardiac arrest. Resuscitation 2012;83:710-4. [Crossref] [PubMed]
- Tramm R, Ilic D, Davies AR, et al. Extracorporeal membrane oxygenation for critically ill adults. Cochrane Database Syst Rev 2015;1:CD010381. [PubMed]
- Cheng R, Hachamovitch R, Kittleson M, et al. Complications of extracorporeal membrane oxygenation for treatment of cardiogenic shock and cardiac arrest: a meta-analysis of 1,866 adult patients. Ann Thorac Surg 2014;97:610-6. [Crossref] [PubMed]
- Sokolovic M, Pratt AK, Vukicevic V, et al. Platelet Count Trends and Prevalence of Heparin-Induced Thrombocytopenia in a Cohort of Extracorporeal Membrane Oxygenator Patients. Crit Care Med 2016;44:e1031-7. [Crossref] [PubMed]
- Bisdas T, Beutel G, Warnecke G, et al. Vascular complications in patients undergoing femoral cannulation for extracorporeal membrane oxygenation support. Ann Thorac Surg 2011;92:626-31. [Crossref] [PubMed]
- Zangrillo A, Landoni G, Biondi-Zoccai G, et al. A meta-analysis of complications and mortality of extracorporeal membrane oxygenation. Crit Care Resusc 2013;15:172-8. [PubMed]
- Russo JJ, Aleksova N, Pitcher I, et al. Left Ventricular Unloading During Extracorporeal Membrane Oxygenation in Patients With Cardiogenic Shock. J Am Coll Cardiol 2019;73:654-62. [Crossref] [PubMed]
- Truby LK, Takeda K, Mauro C, et al. Incidence and Implications of Left Ventricular Distention During Venoarterial Extracorporeal Membrane Oxygenation Support. ASAIO J 2017;63:257-65. [Crossref] [PubMed]
- Lawler PR, Silver DA, Scirica BM, et al. Extracorporeal membrane oxygenation in adults with cardiogenic shock. Circulation 2015;131:676-80. [Crossref] [PubMed]
- Abrams D, Combes A, Brodie D. Extracorporeal membrane oxygenation in cardiopulmonary disease in adults. J Am Coll Cardiol 2014;63:2769-78. [Crossref] [PubMed]
- Bennett CC, Johnson A, Field DJ, et al. UK collaborative randomised trial of neonatal extracorporeal membrane oxygenation: follow-up to age 4 years. Lancet 2001;357:1094-6. [Crossref] [PubMed]
- Peek GJ, Mugford M, Tiruvoipati R, et al. Efficacy and economic assessment of conventional ventilatory support versus extracorporeal membrane oxygenation for severe adult respiratory failure (CESAR): a multicentre randomised controlled trial. Lancet 2009;374:1351-63. [Crossref] [PubMed]
- Nagpal AD, Singal RK, Arora RC, et al. Temporary Mechanical Circulatory Support in Cardiac Critical Care: A State of the Art Review and Algorithm for Device Selection. Can J Cardiol 2017;33:110-8. [Crossref] [PubMed]
- Borisenko O, Wylie G, Payne J, et al. Thoratec CentriMag for temporary treatment of refractory cardiogenic shock or severe cardiopulmonary insufficiency: a systematic literature review and meta-analysis of observational studies. ASAIO J 2014;60:487-97. [Crossref] [PubMed]
- Chan CHH, Pieper IL, Hambly R, et al. The CentriMag centrifugal blood pump as a benchmark for in vitro testing of hemocompatibility in implantable ventricular assist devices. Artif Organs 2015;39:93-101. [Crossref] [PubMed]
- Slaughter MS, Pagani FD, McGee EC, et al. HeartWare ventricular assist system for bridge to transplant: combined results of the bridge to transplant and continued access protocol trial. J Heart Lung Transplant 2013;32:675-83. [Crossref] [PubMed]
- Mehra MR, Uriel N, Naka Y, et al. A Fully Magnetically Levitated Left Ventricular Assist Device - Final Report. N Engl J Med 2019;380:1618-27. [Crossref] [PubMed]
- Ali J, Balasubramanian S, Berman M, et al. Anticoagulant-Free Off-Pump Left Ventricular Assist Device Implant. Ann Thorac Surg 2018;105:e37-9. [Crossref] [PubMed]
- Terzi A. Mechanical circulatory support: 60 years of evolving knowledge. Int J Artif Organs 2019;42:215-25. [Crossref] [PubMed]
- Kirklin JK, Pagani FD, Kormos RL, et al. Eighth annual INTERMACS report: Special focus on framing the impact of adverse events. J Heart Lung Transplant 2017;36:1080-6. [Crossref] [PubMed]
- Uriel N, Pak SW, Jorde UP, et al. Acquired von Willebrand syndrome after continuous-flow mechanical device support contributes to a high prevalence of bleeding during long-term support and at the time of transplantation. J Am Coll Cardiol 2010;56:1207-13. [Crossref] [PubMed]
- Draper KV, Huang RJ, Gerson LB. GI bleeding in patients with continuous-flow left ventricular assist devices: a systematic review and meta-analysis. Gastrointest Endosc 2014;80:435-446.e1. [Crossref] [PubMed]
- Patel H, Madanieh R, Kosmas CE, et al. Complications of Continuous-Flow Mechanical Circulatory Support Devices. Clin Med Insights Cardiol 2015;9:15-21. [PubMed]
- Sacks LD, Hollander SA, Zhang Y, et al. Vasoplegia after pediatric cardiac transplantation in patients supported with a continuous flow ventricular assist device. J Thorac Cardiovasc Surg 2019;157:2433-40. [Crossref] [PubMed]
- Jorde UP, Uriel N, Nahumi N, et al. Prevalence, significance, and management of aortic insufficiency in continuous flow left ventricular assist device recipients. Circ Heart Fail 2014;7:310-9. [Crossref] [PubMed]
- Kormos RL, Teuteberg JJ, Pagani FD, et al. Right ventricular failure in patients with the HeartMate II continuous-flow left ventricular assist device: incidence, risk factors, and effect on outcomes. J Thorac Cardiovasc Surg 2010;139:1316-24. [Crossref] [PubMed]
- Goldstein JA, Tweddell JS, Barzilai B, et al. Importance of left ventricular function and systolic ventricular interaction to right ventricular performance during acute right heart ischemia. J Am Coll Cardiol 1992;19:704-11. [Crossref] [PubMed]