Metabolomic alterations associated with Kallmann syndrome
Introduction
Kallmann syndrome (KS) (congenital hypogonadotropic hypogonadism with anosmia) results from the failure of the gonadotropic releasing hormone (GnRH)-expressing neurons to migrate into the preoptic area from the olfactory placode, resulting in deficient sex hormone production and inadequate gametogenesis when puberty should occur and anosmia/hyposmia. Newborn males may present with micropenis or cryptorchidism (1). KS has more than 20 susceptible genes, including KAL1, FGFR1, PROK2, and PROKR2.2, with significant genetic heterogeneity. The genetic pattern of KS varies greatly, representing autosomal patterns, X-linked patterns, and oligomeric patterns, leading to defects in adolescence, sexuality, reproduction, and olfactory development (2,3). In many cases, the disease is difficult for doctors to identify when performing random pedigree analysis, as there are challenges to addressing the pathways of logical genes and matching genetic mutations to phenotypes, especially when considering atypical symptoms (4,5).
Metabolomics is an emerging “omics” science, which refers to the method of holistic analysis of all low molecular weight metabolites in an organism (6). Due to its high sensitivity, metabolomics methods have been used to develop diagnostic methods for a variety of diseases such as diabetes, multiple sclerosis, cancer by detecting changes in affected metabolites (7-11). There are few metabolomics studies of KS. Seminal plasma is a relatively easy-to-obtain and informative biological sample. In this study, seminal plasma metabolites in KS were used to clarify the relationship between metabolites and sperm function. This metabolomics technique could be a promising screening tool for detecting the causative factors of KS.
Methods
Patients and controls
Semen volunteers were enrolled from Peking Union Medical College Hospital (PUMCH) between March 2018 and March 2019 for metabolomics profiling between April 2018 and March 2019. All participants underwent clinical evaluations and reviewed hospital records (Table 1). KS is a condition that is characterized by hypogonadotropic hypogonadism and reduced or absent olfactory sense due to an anatomical defect of the olfactory nerve. If anosmia is accompanied by absence of pubertal development, Kallman syndrome should be suspected (17/17). The diagnosis is made by means of MRI of the head, as the olfactory sulcus and bulb are bilaterally absent in these patients. Decreased levels of follicle-stimulating hormone and luteinizing hormone should raise suspicion of KS. KS is characterized by reproductive features centered around a lack of sexual maturation during the years of puberty. These signs can include a lack of testicular development as determined by testicular volume in men poorly defined secondary sexual characteristics can include a lack of pubic hair and underdeveloped mammary glands. Micropenis may also be present in a small portion of male cases, while cryptorchidism or undescended testicles may have been present at birth. All of these traits are related to low levels of luteinizing hormone and follicle stimulating hormone, which consequentially results in low testosterone in males.
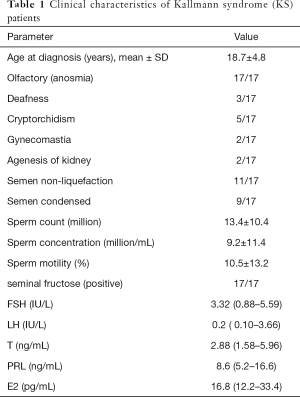
Full table
This study was conducted on the recommendation of the PUMCH institutional committee to protect human subjects. All subjects gave informed consent based on the Helsinki Declaration. The protocol has been approved by the institutional committee that protects human subjects from PUMCH. All methods are implemented in accordance with relevant guidelines and regulations.
Sample preparation for metabolomics profiling
A 40 µL seminal plasma was added to sterile 0.6 mL Eppendorf, and 120 µL of cold methanol/water (9/1) was added. The sample was vortexed at room temperature for 60 seconds, and then placed at −20 °C for 20 minutes to extract metabolites. These samples were centrifugated at 12,000 rpm for 10 minutes and the supernatant was transferred to a new tube. The supernatant was freeze-dried under vacuum and resuspended in 50 µL of methanol/water (1/1), and then centrifuged at 12,000 rpm for 10 minutes at 4 °C. This liquid was used for metabolomic profiling.
Quality control
Quality control sample was made by combining 5 µL of each seminal plasma, stored at −80 °C, and injected every 10 samples according to the HPLC-MS detection sequence.
UPLC-QTOF-MS analysis
The chromatographic and mass spectrometric parameters were used referring to literature (12). The ultra-high performance liquid chromatography (UPLC) column eluate was directly introduced into the mass spectrometer by electrospray. For metabolomics analysis, an ACQUITY UPLC system (Waters Corp., Milford, MA) was used to inject 2 µL of sample into a reverse-phase Waters HSS T3 column (Waters Corp., Milford, MA). Mass spectrometry was performed on XEVO G2 QTOF (Waters) in both positive and negative modes. To maintain accurate mass, the LockSpray interface of sulfadimethoxine (311.0814 [M+H]+ or 309.0658 [M−H]−) was introduced at a rate of 150 µL/min in a 50% ACN aqueous solution at a concentration of 250 pg/µL The mobile phase consists of water (A) and acetonitrile (B) containing 0.1% (v/v) formic acid, using a gradient elution procedure (0.0–18.0 minutes, 95–0% A; 18.1–20.0 minutes, 95% application A). The flow rate was 0.45 mL /min. The column temperature was 45 °C. All chromatograms and mass spectrum data were acquired using MassLynx software (Waters Corp., Milford, MA, USA). In positive ion acquisition mode, MassLynx software collects primary and secondary mass spectral data based on the MSE function of the sample. Capillary voltage: 2.5 kV, cone hole voltage 24 V, ion source temperature 100 °C, desolvent gas flow rate 800 L/h, cone hole gas flow rate 50 L/h, scan ions with m/z 50–1,500 Da within 20 min, 0.2 sec/cycle.
In negative ion acquisition mode, MassLynx software collects primary and secondary mass spectral data based on the MSE function of the sample. Capillary voltage: 2.5 kV, taper hole voltage 25 V, ion source temperature 100 °C, desolvent gas flow rate 600 L/h, taper hole gas flow rate 10 L/h, scan ion with m/z 50–1,500 Da in 14 min, 0.2 sec/cycle.
Data processing and multivariate data analysis
Raw mass spectrometric data were imported into Progenesis QI (Waters) software for data preprocessing, alignment, deconvolution, peak extraction, multivariate statistical analysis and identification. After importing the original data, the automatic mode was selected for data processing, mass fraction correction was performed by Lockmass, and QC samples were used as the reference group for peak alignment.
Statistical analysis and putative ion identification on the postprocessed data were conducted using MetaboLyzer (13). In this experiment, metabolites with a CV value of less than 30%, VIP >1, and P value <0.05 in the QC samples were selected as differential metabolites which utilizes the Human Metabolome Database (HMDB), the Kyoto Encyclopaedia of Genes and Genomes (KEGG) database for searching. The m/z values were compared with the exact mass of small molecules in the databases, all metabolites were identified by “Score”, “Fragmentation Score”, “Mass Error (ppm)” and “Isotope Similarity” Scoring to pick the best matching metabolite name. Pathways associated with these differential metabolites in the positive and negative ion mode of each group obtained by statistical analysis were annotated by MetaboAnalyst 4.0. The results of the pathway analysis are presented in the form of graphics and detailed tables.
MetaboAnalyst (Xia Lab @ McGill Sweden) was used for Partial Least Squares Discrimination Analysis (PLS-DA). The heat map displaying the relative levels of differential metabolites was generated using MetaboAnalyst (14).
Statistical analysis
Experimental values are expressed as mean ± SD. Statistical analysis was performed using MetaboAnalyst. The significance of metabolite levels was determined using t test. A P value of less than 0.05 is considered significant.
Results
Baseline clinical characteristics of KS patients
All the 17 KS patients were Han Chinese population (100%). Their average age was 18.7±4.8 years old. Their clinical characteristics are shown in Table 1.
Seminal plasma metabolomics
We analyzed the metabolomics data of patients with KS and HC using the UPLC-QTOF-MS method, aiming to discover metabolomics changes related to KS. MetaboAnalyst software was used to generate a partial least squares discriminant analysis (PLS-DA) plots and to analyze the differences (Figure 1). Individuals and variables with similar contours in the partial least squares discriminant analysis (PLS-DA) graph are grouped together in the graph. The red and green spots in this PLSDA chart represent two separate HC (healthy control) and KS patients. The results showed significant differences in metabolomics data between the KS and HC groups. And the difference ions between these two groups are statistically significant, as shown in the volcano plot (Figure 2). In the volcano plot, pink dots indicate the presence of significantly different (P values less than 0.05) levels of ions between patients with KS and HC. As mentioned earlier, putative molecules for these differential ions are specified by screening for accurate masses in the metabolite database. RF algorithms were used to generate a heat map of these differential metabolites for these two groups (Figure 3). KEGG pathway analysis results indicate metabolic pathways related to differential metabolites. The prominent path for which the P value of the false discovery rate (FDR) correction is less than 0.25 is shown in Figure 4.
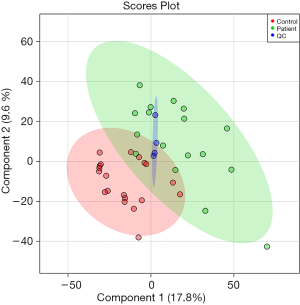
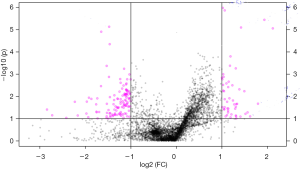
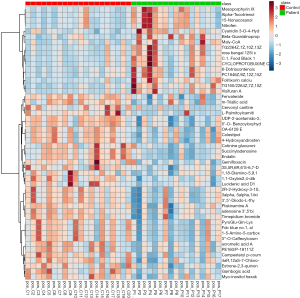
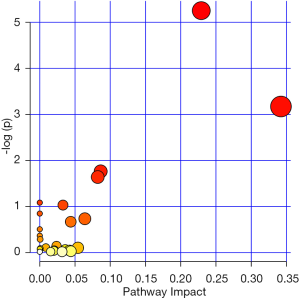
Discussion
KS is a rare disease that is difficult to diagnose early due to the lack of specific biomarkers. This study aimed to explore the potential diagnostic markers of seminal plasma in KS using a metabolomics approach. Our results suggest that changes in linoleic acid (LA) metabolism and glycerophospholipid (GPC) metabolism may serve as indicators of KS diagnosis (Table 2).
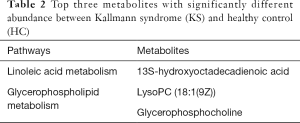
Full table
LA is an essential fatty acid that cannot be synthesized by the body. In the human body, LA has four main destinies. It can be used as an energy source, like all fatty acids. Esterification can occur to form neutral and polar lipids such as phospholipids, triacylglycerols and cholesterol esters. As part of membrane phospholipids, LA maintains membrane fluidity as a structural component. In addition, when released from membrane phospholipids, derivatives oxidized by enzymes can participate in cell signaling. LA can also be metabolized via multiple pathways to form several proinflammatory eicosanoids, such as prostaglandins, thromboxane, and leukotrienes (15-17). The number of sperm is the main indicator of fertility. The total number of spermatozoa per healthy adult male is at least 39×106 per ejaculation. Sperm concentration refers to the number of spermatozoa in a unit volume. The concentration of spermatozoa per healthy adult male is at least 15×106/mL. Sperm must have a certain amount of exercise ability to achieve natural fertility. Healthy adult males should have no less than 32% forward motile spermatozoa (range of 31% to 34%), and no less than 40% forward motile and non-forward motile spermatozoa (range 38% to 42%). In this study, the KS patients had lower sperm count [(13.4±10.4)×106], sperm concentration [(9.2±11.4)×106/mL] and sperm motility (10.5%±13.2%), which may be associated with the changes in prostaglandins. Besides, the changed inflammatory eicosanoids, such as prostaglandins, thromboxane, and leukotrienes, may also have some effects on the function of plasmin, causing the semen non-liquefaction (11/17) and semen condensed (9/17).
13-Hydroxyoctadecadienoic acid (13-HODE) is the major lipoxygenation product synthesized in the body from LA. 13-HODE is considered an intermediate in LA metabolism. It is generated from 13(S)-HPODE via the enzyme lipoxygenase. 13-HODE has been shown to be involved in cell proliferation and differentiation in a number of systems.
LysoPC (18:1(9Z)) is a lysophospholipid (LyP) and also a monoglycerol phospholipid, consists especially of the oleic acid chain at the C-1 position. Most tissues contain a small amount of lysophosphatidylcholine, which is formed by phospholipase A2 hydrolysis of phosphatidylcholine. In plasma, a large amount of lysophosphatidylcholine is formed by a specific enzyme system (lecithin: cholesterol acyltransferase, LCAT) which is secreted from the liver. The enzyme catalyzes the transfer of the fatty acid at the sn-2 position of phosphatidylcholine to free cholesterol in plasma to form cholesterol ester and lysophosphatidylcholine. Lysophospholipids play a role in lipid signaling by acting on the lysophospholipid receptor (LPL-R) (18,19).
Glycerylphosphorylcholine (GPC) is one of the two main forms of choline storage in the cytoplasm (together with choline phosphate). GPC as an osmolytes, is one of the four main organic permeants [glyceryl phosphate choline (GPC), betaine, inositol, and sorbitol] in renal myeloid cells. Changes in intracellular permeate concentration and extracellular tension can offset the effects of urea on enzymes and other macromolecules. By increasing uptake (inositol and betaine) and synthesis (sorbitol, GPC), reducing degradation (GPC), and reducing the release of permeate, the intracellular content of these permeate can be increased. GPC is formed during the decomposition of phosphatidylcholine (PtC). Recent studies have demonstrated the potential diagnostic role of GPC in semen plasm for infertile men (20-24).
Conclusions
The main different metabolic pathways focus on LA metabolism, Glycerophospholipid metabolism, suggesting that Glycerophospholipids and LA may provide insights for the diagnosis of KS. Since KS is a rare disease, all patients in this study were from a single center and the sample size was relatively small, we also need independent validation cohorts to further evaluate the performance of these biomarkers. We cannot rule out that the results of this experiment have certain limitations to the Chinese population. Therefore, it is better to have multi-center studies to strengthen the conclusions of this study.
Acknowledgments
Funding: This work was supported in part by the National Nature Science Foundation of China (No. 81671618, 81871302), the CAMS Initiative for Innovative Medicine (2017-I2M-3-001), the CAMS Initiative for Innovative Medicine (2017-I2M-B&R-01).
Footnote
Conflicts of Interest: All authors have completed the ICMJE uniform disclosure form (available at http://dx.doi.org/10.21037/atm.2020.04.03). The authors have no conflicts of interest to declare.
Ethical Statement: The authors are accountable for all aspects of the work in ensuring that questions related to the accuracy or integrity of any part of the work are appropriately investigated and resolved. This study was carried out in accordance with the recommendations of the institutional committee for the Protection of Human Subjects from PUMCH. All methods were performed in accordance with the relevant guidelines and regulations. Written informed consent was obtained from the patient for publication of this study and any accompanying images.
Open Access Statement: This is an Open Access article distributed in accordance with the Creative Commons Attribution-NonCommercial-NoDerivs 4.0 International License (CC BY-NC-ND 4.0), which permits the non-commercial replication and distribution of the article with the strict proviso that no changes or edits are made and the original work is properly cited (including links to both the formal publication through the relevant DOI and the license). See: https://creativecommons.org/licenses/by-nc-nd/4.0/.
References
- Schwanzel-Fukuda M, Bick D, Pfaff DW. Luteinizing hormone-releasing hormone (LHRH)-expressing cells do not migrate normally in an inherited hypogonadal (Kallmann) syndrome. Brain Res Mol Brain Res 1989;6:311-26. [Crossref] [PubMed]
- Pitteloud N, Meysing A, Quinton R, et al. Mutations in fibroblast growth factor receptor 1 cause Kallmann syndrome with a wide spectrum of reproductive phenotypes. Mol Cell Endocrinol 2006;254-255:60-9. [Crossref] [PubMed]
- Maione L, Dwyer A, Francou B, et al. Genetic counseling for congenital hypogonadotropic hypogonadism and Kallmann syndrome: new challenges in the era of oligogenism and next generation sequencing. Eur J Endocrinol 2018;178:R55-R80. [Crossref] [PubMed]
- Caronia LM, Martin C, Welt CK, et al. A genetic basis for functional hypothalamic amenorrhea. N Engl J Med 2011;364:215-25. [Crossref] [PubMed]
- Kaplan JD, Bernstein JA, Kwan A, et al. Clues to an early diagnosis of Kallmann syndrome. Am J Med Genet A 2010;152A:2796-801. [Crossref] [PubMed]
- Nicholson JK, Lindon JC. Systems biology: metabonomics. Nature 2008;455:1054-6. [Crossref] [PubMed]
- Zhou L, Wang Q, Yin P, et al. Serum metabolomics reveals the deregulation of fatty acids metabolism in hepatocellular carcinoma and chronic liver diseases. Anal Bioanal Chem 2012;403:203-13. [Crossref] [PubMed]
- Wu H, Xue R, Tang Z, et al. Metabolomic investigation of gastric cancer tissue using gas chromatography/mass spectrometry. Anal Bioanal Chem 2010;396:1385-95. [Crossref] [PubMed]
- Xu J, Zhang J, Cai S, et al. Metabonomics studies of intact hepatic and renal cortical tissues from diabetic db/db mice using high-resolution magic-angle spinning 1H NMR spectroscopy. Anal Bioanal Chem 2009;393:1657-68. [Crossref] [PubMed]
- Lian JS, Liu W, Hao SR, et al. A serum metabolomic analysis for diagnosis and biomarker discovery of primary biliary cirrhosis and autoimmune hepatitis. Hepatobiliary Pancreat Dis Int 2015;14:413-21. [Crossref] [PubMed]
- Reinke SN, Broadhurst DL, Sykes BD, et al. Metabolomic profiling in multiple sclerosis: insights into biomarkers and pathogenesis. Mult Scler 2014;20:1396-400. [Crossref] [PubMed]
- Li HH, Tyburski JB, Wang YW, et al. Modulation of fatty acid and bile acid metabolism by peroxisome proliferator-activated receptor alpha protects against alcoholic liver disease. Alcohol Clin Exp Res 2014;38:1520-31. [Crossref] [PubMed]
- Mak TD, Laiakis EC, Goudarzi M, et al. MetaboLyzer: a novel statistical workflow for analyzing postprocessed LC-MS metabolomics data. Anal Chem 2014;86:506-13. [Crossref] [PubMed]
- Laiakis EC, Hyduke DR, Fornace AJ. Comparison of mouse urinary metabolic profiles after exposure to the inflammatory stressors gamma radiation and lipopolysaccharide. Radiat Res 2012;177:187-99. [Crossref] [PubMed]
- Khanapure SP, Garvey DS, Janero DR, et al. Eicosanoids in inflammation: biosynthesis, pharmacology, and therapeutic frontiers. Curr Top Med Chem 2007;7:311-40. [Crossref] [PubMed]
- Bogatcheva NV, Sergeeva MG, Dudek SM, et al. Arachidonic acid cascade in endothelial pathobiology. Microvasc Res 2005;69:107-27. [Crossref] [PubMed]
- Monk JM, Turk HF, Fan YY, et al. Antagonizing arachidonic acid-derived eicosanoids reduces inflammatory Th17 and Th1 cell mediated inflammation and colitis severity. Mediators Inflamm 2014;2014:917149.
- Pietiläinen KH, Róg T, Seppänen-Laakso T, et al. Association of lipidome remodeling in the adipocyte membrane with acquired obesity in humans. PLoS Biol 2011;9:e1000623. [Crossref] [PubMed]
- Wymann MP, Schneiter R. Lipid signalling in disease. Nat Rev Mol Cell Biol 2008;9:162-76. [Crossref] [PubMed]
- Vorkas PA, Isaac G, Holmgren A, et al. Perturbations in fatty acid metabolism and apoptosis are manifested in calcific coronary artery disease: an exploratory lipidomic study. Int J Cardiol 2015;197:192-9. [Crossref] [PubMed]
- Vouk K, Ribič-Pucelj M, Adamski J, et al. Altered levels of acylcarnitines, phosphatidylcholines, and sphingomyelins in peritoneal fluid from ovarian endometriosis patients. J Steroid Biochem Mol Biol 2016;159:60-9. [Crossref] [PubMed]
- Zhao YY, Wang HL, Cheng XL, et al. Metabolomics analysis reveals the association between lipid abnormalities and oxidative stress, inflammation, fibrosis, and Nrf2 dysfunction in aristolochic acid induced nephropathy. Sci Rep 2015;5:12936. [Crossref] [PubMed]
- Ried JS, Baurecht H, Stuckler F, et al. Integrative genetic and metabolite profiling analysis suggests altered phosphatidylcholine metabolism in asthma. Allergy 2013;68:629-36. [Crossref] [PubMed]
- Deepinder F, Chowdary HT, Agarwal A. Role of metabolomic analysis of biomarkers in the management of male infertility. Expert Rev Mol Diagn 2007;7:351-8. [Crossref] [PubMed]