Distinct secretion pattern of serum proinsulin in different types of diabetes
Introduction
Latent autoimmune diabetes in adults (LADA) stands for late-onset (later than 30-year-old) diabetes patients identified from type 2 diabetes mellitus (T2DM) by beta-cell autoimmunity, which is assessed by serum islet autoantibodies (1,2). LADA patients are independent from insulin treatment for at least 6 months after disease initiation, but would progress considerably faster towards total loss of pancreatic beta-cell than T2DM patients (3). Adult-onset type 1 diabetes mellitus (T1DM) and LADA patients composed of the whole adult-onset autoimmune diabetes population (4). When compared to classic T1DM, the genetic loads, beta-cell autoimmunity and beta-cell deprivation rate of adult-onset autoimmune diabetes was significantly lower in LADA patients (5-8), but studies on differences between adult-onset T1DM and LADA were scarce. Underneath mechanisms of a much slower beta-cell damage in LADA than adult-onset T1DM, especially on beta-cell intrinsic properties, were still largely unknown.
It is well-known that the development of T1DM is dominantly autoimmune-derived, whereas LADA, which accounts for a large amount in autoimmune diabetes, shares milder autoimmunity in the disease development (1,9,10). Yet, recent discoveries have elucidated alternative disruption of pancreatic beta-cells by non-autoimmune processes (11,12), especially pathological pathways intrinsic to beta-cells during the development of T1DM (13,14). Also, as a possible intermediate form between T1DM and T2DM, LADA shares features like insulin resistance with T2DM, through which overwhelmed beta-cells could suffer from excessive endoplasmic reticulum (ER) stress (12,15). The production of insulin in pancreatic beta-cells is tightly controlled by a series of organelles involving ER (16). Proinsulin (PI), the direct matrix of bio-reactive insulin, processed in ER, stands at the checkpoint of insulin production (17). PI has been largely proved to be actively participated in promoting autoimmunity (18,19). Meanwhile, disturbed beta-cells with dysfunctional ER would discharge improperly modified PI molecules and correspondingly decreased amount C-peptide (CP) and insulin, which provided a reasonable method for identifying ER stress, a sign of beta-cell disturbances, from peripheral blood by measuring serum PI to CP (PI:CP) or PI to insulin ratios (20). Studies on beta-cell lines and rodent models found that pathways involved in ER stress during PI processing were crucial for beta-cell function and survival (21,22) and early autoimmunity initiation (23). Meanwhile, disproportionately elevated PI was a sensitive biomarker for ER dysfunction (24,25). Peripheral PI concentration in human was intimately related to beta-cell stress (26). In human T1DM patients, elevated circulating PI and PI:CP ratios, abnormal expression of ER stress markers were found in newly onsets (27,28). Scientists also adduced that increased PI:CP ratio was related to T1DM progression in first-degree relatives with positive serum islet autoantibodies (24,25,29,30). In T2DM, ER stress has also shown early participation in disease initiation (11,31). In long-established T1DM, PI appeared to be an effective measurement of residual beta-cell function in individuals with undetectable insulin and CP levels (32). Aforementioned evidence indicated that, either in T1DM or in T2DM, PI:CP ratio is an early trait and a sensitive biomarker of beta-cell destruction either due to intrinsic beta-cell dysfunction or autoimmune attack. Conclusively, results on the important role of ER stress in both T1DM and T2DM enlightened corresponding research on LADA patients, to elucidate insulin production changes in the development of LADA and to evaluate how beta-cell autoimmunity has participated in this process.
Herein, we designed a study in exploring beta-cell function and dysfunction in newly diagnosed and adult-onset diabetes patients, including T1DM, LADA and T2DM. In this study, we focused on PI-related measurements, namely fasting and postprandial PI and PI:CP ratio, and in this manner may we probe into the beta-cell intrinsic pathogenesis mechanisms of different types of diabetes, especially LADA.
Methods
Study subjects
Sixty T1DM, 60 LADA and 60 T2DM patients diagnosed within 2 years were recruited in the Second Xiangya Hospital of Central South University, Changsha, China. All subjects were diagnosed with diabetes after 18-year-old. Diabetes was diagnosed on the basis of the World Health Organization (WHO) criteria for diabetes mellitus [1999] (33). Both T1DM and T2DM were diagnosed according to the criteria of American Diabetes Association (ADA) (34). LADA patients were diagnosed according to the 2005 Immunology of Diabetes Society (IDS) criteria with (I) at least one positive serum islet autoantibody [glutamate decarboxylase autoantibody (GADA), protein tyrosine phosphatase autoantibody (IA-2A) and zinc transporter 8 autoantibody (ZnT8A)]; (II) hyperglycemia controlled with insulin-independent therapy for at least 6 months after disease onset; (III) disease onset at 30-year-old or older (35). Specific medical treatments, including glucagon-like peptide 1 (GLP-1) receptor agonists and dipeptidyl peptidase 4 (DDP-4) antagonists, pancreas or islet transplantation, metabolic surgeries and immunotherapy, could directly manipulate beta-cell function and change the production of either insulin, CP or PI. Therefore, patients underwent these prescriptions were excluded. Pancreatectomy, pancreatitis, and injuries or diseases (other than diabetes) adversely affect pancreas function or immune system could possibly manipulate the beta-cell performance, and hence were also listed in our exclusion criteria.
Anthropometric and biochemical measurements
Height, body weight, waist and hip circumferences and blood pressure were measured in a standardized procedure. Based on these measurements, body mass index (BMI) and waist-hip ratio (WHR) were calculated as follow: BMI (kg/m2) = body weight [kilograms, kg] ÷ [height (meters, m)]2 and WHR = Waist circumferences [meters, m] ÷ Hip circumferences [meters, m]. Fasting serum samples were obtained from all 180 patients. Among them, 30 T1DM, 30 LADA and 30 T2DM patients underwent mixed meal tolerance tests (MMTTs), and hence 120 min postprandial serum samples were collected. Total serum PI concentration, including fasting PI (FPI) and 120 min postprandial PI (PPI), were measured with STELLUX Proinsulin Chemiluminescence enzyme-linked immunosorbent assay (ELISA) kit (ALPCO) on serum samples stored at minus 80 degrees centigrade. Upon detection, all samples underwent less than two freeze/thaw cycles. Cross-reactivity with human CP and human insulin of this ELISA kit are reported to be lower than 0.01% and 0.1%, respectively. To obtain the concentration of samples with Chemiluminescence reads (relative light units, RLU) below the lower limit of quantitation (LLOQ), we calculated with a simple linear equation based on two points, namely point “zero” and “the lowest standard concentration on the standardized curve” of each ELISA plate. Following detection were conducted with serum samples upon collection: both fasting CP (FCP) and 120 min postprandial CP (PCP) levels were measured by a chemiluminescence kit (Adiva Centaur System, Siemens, Germany); fasting and postprandial PI:CP ratios were calculated as molar ratios of PI (pmol/L) to CP (pmol/L) multiplied by 100%; fasting blood glucose (FBG), 120 min postprandial blood glucose (PBG), triglycerides (TG), total cholesterol (TC), high-density lipoprotein cholesterol (HDL), and low-density lipoprotein cholesterol (LDL) were measured by an automatic chemistry analyzer (Hiterent 7170A, Japan); fasting venous blood collected in anti-coagulate tubes were used in hemoglobin A1c (HbA1c) detection, using automated liquid chromatography (Bio-Rad VARIANT-II Hemoglobin Testing System, United States); the detection of three serum islet autoantibodies, namely GADA, IA-2A and ZnT8A, were of Diabetes Antibody Standardization Program (DASP) standard and measured by radioligand binding assay in duplicate.
Statistical analyses
Chi-square tests were applied on categorical variables, and Fisher exact tests were used for crosstabs cells with less than five expected counts. For variables with more than 2 categories, Cramer’s V value were calculated. All continuous variables, excepting BMI and WHR, are not normally distributed based on Shapiro-Wilk tests and Quantile-Quantile plots. Hence, the medians (interquartile ranges, IQRs) were calculated and compared among groups by Kruskal-Wallis tests with Bonferroni post hoc comparisons. For BMI and WHR, ANOVA tests were performed with Bonferroni post hoc comparisons. Age and BMI were not perfectly matched across groups, and data on these analyses were not adjusted for these two measurements and were therefore descriptive. Generalized linear model was applied to assess differences of PI-related parameters with skewed distribution in different groups adjusted for age, BMI, and diabetes duration. Receiver operating characteristic (ROC) curves were plotted and area under ROC curves (AUCROC) was calculated based on fasting PI-related measurements for all patients and 120 min postprandial PI-related measurements for patients underwent MMTTs. Spearman correlation analysis and Mantel-Haenszel Chi-Square tests were performed along with Spearman correlation covariates (rs) and Goodman and Kruskal’s gamma [G] values calculated to assess relationships between beta-cell autoimmunity and PI-related parameters.
All tests were considered significant with two-sided P values lower than 0.05. Statistical analysis was carried out with GraphPad Prism version 8.0.0 for Windows (GraphPad Software, California, USA) and IBM SPSS version 25.0 for Windows (IBM Corp, IBM SPSS Statistics, Armonk, NY, USA).
Results
PI-related measurements in LADA were of intermediate levels between T1DM and T2DM
Fasting measurements were compared among groups for all 180 patients, and 120 min postprandial measurements were compared among groups for 90 patients underwent MMTTs (Table 1). Anthropometric and biochemical characteristics showed in Table 1 were compared among three types of diabetes without adjustments for unmatched parameters, namely age and BMI, and were therefore descriptive. There were no differences in diabetes duration or sex among groups. Age was significantly different among groups, and T1DM patients were younger than both LADA and T2DM patients (P<0.001 for both comparisons). Under ANOVA tests, significant differences were presented among groups for both BMI and WHR (for both measurements P<0.001, and post hoc comparisons showed P=0.003 between T1DM and LADA, P<0.001 between LADA and T2DM and P<0.001 between T1DM and T2DM).
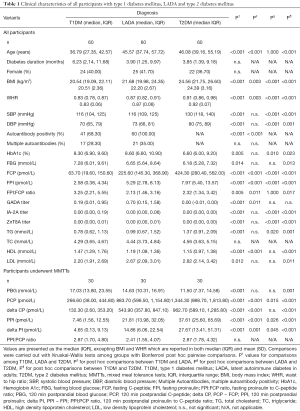
Full table
Glucose metabolism parameters measured at fasting states, including FBG and HbA1c, presented hyperglycemia of all three groups and revealed better glycemic control in T2DM than both T1DM and LADA. As expected, T1DM had the lowest levels of FCP and FPI, and these levels were significantly lower in LADA than in T2DM (P<0.001 for all comparisons, Figure 1A). The median of FPI in T1DM was 2.58, and were 5.29 and 7.97 in LADA and T2DM, respectively. Medians of FPI:FCP ratio of T1DM, LADA and T2DM were 3.25%, 2.13% and 2.32%. FPI:FCP ratio was significantly different among groups as shown in Figure 1B (P=0.005). Pairwise comparisons revealed higher FPI:FCP ratio in T1DM than LADA as well as in T1DM than T2DM (P=0.011 and P=0.017, respectively), but these differences were not sustained between LADA and T2DM. Among 90 patients underwent MMTTs, the distribution of PBG was only significant different between T1DM and T2DM (P<0.001) but not between either T1DM and LADA or between T2DM and LADA. Median of PPI and delta PI were listed as follow: in T1DM, median PPI =7.46 and median delta PI =4.65, in LADA these two levels were 21.81 and 14.86 while in T2DM they were 37.61 and 27.67. As observed in comparisons on fasting parameters, the concentration of PCP, PPI, delta CP and delta PI were higher in LADA patients than T1DM but were lower than that in T2DM (Figure 1C,D). When it came to PPI:PCP ratio, the differences among three groups did not reach statistical significance (P=0.699, Figure 1E).
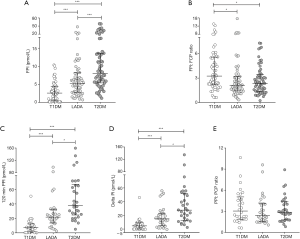
Because all eight beta-cell function measurements, including FCP, FPI, FPI:FCP ratio, PCP, PPI, delta CP, delta PI and PPI:PCP ratio, showed skewed distributions, comparisons of these parameters among groups were adjusted using generalized linear models with log-linked gamma distribution, followed by least significance differences (LSD) tests for pairwise post-hoc comparisons (Table 2). After adjustments for (I) age; (II) age and BMI; (III) age, BMI and diabetes duration, differences among groups remained significant except for (I) in model 2 adjusting age among groups, the difference of FPI:FCP ratio was not significant between T1DM and LADA (P>0.05) and (II) in model 3 adjusting age and BMI and model 4 adjusting age, BMI and diabetes duration, the differences of FPI were not significant between LADA and T2DM (P>0.05).
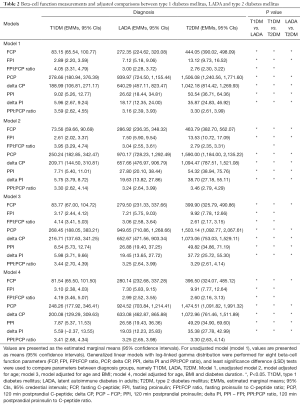
Full table
PI secretion patterns were corelated to autoimmunity in autoimmune diabetes
Islet autoimmune antibodies characterized both T1DM and LADA. As indicated in previous studies, the number of autoantibodies represented the severity of beta-cell autoimmunity, especially in LADA (36). These autoantibodies were responsible for immune attacks targeting pancreatic beta-cells and subsequent beta-cell dysfunctions. Hence, correlations between autoimmune reactions and the secreting patterns of PI were measured to evaluate possible relationships between beta-cell dysfunction and autoimmunity. We have tested these relationships in LADA patients (n=60), as well as the whole autoimmune diabetes population which encompasses T1DM and LADA (n=120). For postprandial measurements, these relationships were tested on participants underwent MMTTs [LADA patients (n=30), as well as the whole autoimmune population (n=60)].
According to Spearman correlation analysis, among autoimmune diabetes patients, the autoantibody IA-2A titer was positively correlated with FPI:FCP ratio (rs=0.202, P=0.027). Among LADA patients, rs=0.429, rs=0.413, respectively for correlations between IA-2A titer and PPI:PCP ratio and between ZnT8A titer and PPI:PCP ratio (P=0.018, P=0.023, respectively). Meanwhile, trends in autoimmune reactions by PI-related measurements quartile were observed. In these trend tests, we divided the whole autoimmune population (including T1DM and LADA patients) and LADA patients by either FPI or FPI:FCP ratio quartiles (by second, third and fourth quartile into Q1, Q2, Q3 and Q4). Ordinal to ordinal correlations were tested by Goodman and Kruskal’s gamma (G). In the whole autoimmune population, Mantel-Haenszel Chi-square tests showed a likelihood of increasing FPI from negative autoantibody to positive autoantibody (n=30 for each quartile, P=0.003, Figure 2A), and G =0.547 for correlations between FPI quartiles and autoantibody positivity (P=0.002). Trend from zero autoantibody to three positive autoantibodies by FPI quartile were also revealed in the whole autoimmune population (n=30 for each quartile, P=0.036, Figure 2B), and G =0.226 between FPI quartile and positive autoantibody numbers (P=0.036). In LADA, significant trends were observed from one positive autoantibody to three positive autoantibodies by FPI:FCP ratio quartile (n=15 for each quartile, P=0.007, Figure 2C), and G =0.447 between FPI:FCP ratio quartile and positive autoantibody numbers (P=0.010). Autoimmunity increased by PPI:PCP ratio quartile reached statistical significance in positive autoantibody number in LADA patients (Q1 n=8, Q2 n=7, Q3 n=8, Q4 n=7, P=0.034, Figure 2D), and G =0.517 for correlation between PPI:PCP ratio quartile and positive autoantibody number (P=0.021).

PI-related parameters could discriminate LADA from T1DM and T2DM
To evaluate the possibility of applying PI-related parameters in discriminating forms of diabetes, we plotted ROC curves of FPI and FPI:FCP ratio separately on all patients, and of PPI, delta PI, and PPI:PCP ratio separately on patients underwent MMTTs. These curves were established between T1DM and LADA, as well as between LADA and T2DM (Figure 3). The AUCROC of ROC curves built between LADA and T1DM, as well as between LADA and T2DM for FPI, PPI, and delta PI reached 0.700, and we calculated cut-off values choosing Youden index as the technical criterion for these discriminating tests. ROC curves between T1DM and LADA (Figure 3A) demonstrated (I) FPI AUCROC for discriminating LADA and T1DM was 0.751 (P<0.001, cut-off =3.76, sensitivity =66.67%, specificity =71.67%), (II) delta PI AUCROC for discriminating LADA and T1DM was 0.793 (P<0.001, cut-off =11.06, sensitivity =66.67%, specificity =90.00%) and (III) PPI AUCROC for discriminating LADA and T1DM was 0.838 (P<0.001, cut-off =12.45, sensitivity =80.00%, specificity =76.67%). Between LADA and T2DM (Figure 3B), ROC curves revealed (I) FPI AUCROC for discriminating LADA and T2DM was 0.685 (P<0.001). For patients underwent MMTTs, (II) delta PI AUCROC for discriminating LADA and T2DM was 0.709 (P=0.005, cut-off =23.09, sensitivity =76.67%, specificity =60.00%) and (III) PPI AUC for discriminating LADA and T2DM was 0.741 (P<0.01, cut-off =35.21, sensitivity =86.67%, specificity =56.67%).
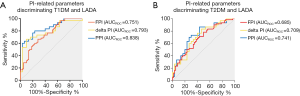
Discussion
In this cross-sectional study, we summarized PI secretion patterns among adult-onset T1DM, LADA and T2DM patients at their onsets. Specifically, LADA patients possess mid-way beta-cell properties between adult-onset T1DM and T2DM as measured by PI-related parameters. These parameters were applicable in discriminating LADA from adult-onset T1DM and LADA from T2DM. We also presented positive correlations between beta-cell autoimmunity and impaired beta-cell function among autoimmune diabetes, especially in LADA. These results indicated that beta-cell autoimmunity and beta-cell ER stress could possibly synergize beta-cell destructions.
Dysfunctional pancreatic beta-cell is the final denominator of all forms of diabetes. ER stress, which is efficiently and accurately measured by PI to insulin or PI to C-peptide ratios in peripheral blood of human, has been corroborated by an increasing number of studies to be involved in beta-cell intrinsic pathogenesis pathways related to the development of diabetes. Increased secretion of PI and the elevation of PI:CP ratio were considered signs of both early beta-cell intrinsic dysfunctionality among individuals about to progress T1DM and T2DM (24,31) and among T1DM patients at onset (27). In T1DM, ER stress intricately connected autoimmunity and inflammatory processes during beta-cell destruction (14). Dysfunctional ER provides stressing environments for protein modification and could accelerate ER stress to a terminate extend, resulting in the generation of neo-antigens and activation of immune responses (37). In turn, autoimmunity, which is responsible for insulitis, could add on inflammation and then further induce ER stress (38). In these regards, blockage of ER stress signaling pathways could reverse T1DM (39,40), and reducing ER stress by targeting key molecules in ER function has become a plausible way in relieving diabetes (22,41). When it comes to T2DM, in which insulin resistance has a dominant contribution, upregulated PI production could reveal poor beta-cell function (42), and attenuating ER stress could alleviate beta-cell damage. Considered as a milder form of autoimmune diabetes which shared T2DM traits, ER stress could be possibly involved in autoimmunity and inflammatory process in LADA. However, there has been few explorations concerning ER stress and PI-related measurements in LADA. A former study found increased PI:CP ratios and defective PI processing features in offspring of LADA patients with positive GADA (43). Hence, we, for the first time, performed this study to assess beta-cell dysfunctionality in LADA and to demonstrate differences among different types of diabetes.
We demonstrated intermediate levels of fasting PI, delta PI and postprandial PI in LADA patients between adult-onset T1DM and T2DM. Besides, fasting PI:CP ratio was significantly higher in patients in the T1DM group than either LADA or T2DM. These differences remained significant after adjustments for age, BMI and diabetes duration. Increasing PI levels from T1DM, through LADA, to T2DM observed in our study were in consistence with the increasing insulin secretion capacity in that order (6), and hence could be a revelation of remaining beta-cell function in each type. That is, T1DM patients possess the lowest residual beta-cell function, T2DM retained the highest, and LADA stood between them. When it comes to PI:CP ratio, the obviously higher ratio in T1DM than in LADA indicated severer disproportionated hyperproinsulinemia in T1DM. Possibly the already largely destructed beta-cell population in T1DM, owing to more intense genetic predispositions and abnormalities, higher levels of insulitis, severer beta-cell autoimmunity and faster disease progression, could not resist the extremely uncontrolled hyperglycemia (5,8,12,44). Consequently, excessive requirements on insulin production resulted in extra ER stress and increased amounts of un-processed PI molecule, instead of insulin and CP, to be released into peripheral blood, and hence could account for the disproportionated hyperproinsulinemia in T1DM. When it comes to LADA patients who have experienced slower less intense beta-cell destruction than T1DM, the beta-cell dysfunctionality was not as detrimental as it was in T1DM. But we could not credit similar pathogenetic mechanisms in both LADA and T2DM to the indiscrimination on PI:CP ratios between them. As demonstrated in the former study investigating PI secretion in LADA, it was under hyperglycemic clamp test, rather than oral glucose tolerance test, that the significantly increased PI and PI:CP ratios were observed between LADA offspring at high diabetes risks and individuals with normal glucose tolerance (43). Presumably, in LADA, an excessively intense beta-cell stress, which could have resulted from metabolic loads stronger than MMTTs, was required for uncovering the beta-cell dysfunctionality, but further explorations focusing the beta-cell stress loads are required to confirm the speculation. Recent studies also observed severer impaired PI processing in non-obese T2DM than obese T2DM, indicating still unknown ER dysfunction mechanisms in T2DM other than insulin resistance, which has long been proposed to be responsible for disproportionated hyperproinsulinemia in T2DM (45). Thus, these possible reasons could explain comparable PI:CP in LADA and T2DM.
We further carried out correlation analysis between beta-cell autoimmunity and PI secretion patterns, and positive correlations between IA-2A titers and FPI:FCP ratios, between IA-2A titers and PPI:PCP ratios and between ZnT8A and PPI:PCP ratios were observed among the whole autoimmune diabetes population, including T1DM and LADA. While in LADA patients, besides the aforementioned correlations between autoantibody titers and PI:CP ratios, positive correlations were also observed between both fasting and postprandial PI:CP ratios and numbers of positive autoantibody. These results indicated a negative correlation between the intensity of autoimmunity and the capability of beta-cells in resisting stresses: the severer the immune responses, the heavier that beta-cell were stressed, and hence the production of PI could be adversely affected, to which the disproportionated hyperproinsulinemia was led. Also, both IA-2A and ZnT8A were pancreatic beta-cell-specific autoantibodies and were directly involved in beta-cell destructions, and hence possibly these autoantibodies could be sensitive biomarkers for beta-cell function and stress (46-49). Based on this, we may presume that PI secretion patterns in T1DM and LADA was intimately manipulated by immune attacks, but different levels of autoimmunity should be responsible for their differences in PI secretion patterns. Besides, these correlations indicated fundamentally different underneath mechanisms for beta-cell destructions in LADA when compared to T2DM.
In T1DM, former studies suggested synergized effects from both beta-cell autoimmunity and beta-cell intrinsic dysfunction (14). These two mechanisms were possibly connected and could have formed a vicious cycle, which actively involved in the destruction of pancreatic beta-cells: autoimmunity could promote inflammations which will further accelerate ER stress and successive pro-death processes (50-52), and ER stress were intimately involved in neo-antigen generation and self-recognition through posttranslational modifications, protein degeneration processes, assisted antigen presenting, etc. (53-56). These may account for the relationship between beta-cell autoimmunity and ER stress found in our study. But beta-cell autoimmunity is far more complicated than what we could observe from islet autoantibodies: there were possible fusional mechanisms for the pathogenesis of autoimmune diabetes through which beta-cells were attacked by synergized beta-cell failure from within beta-cells and immune responses from without. Intrinsic and eccentric retaliations supported each other and were both responsible for beta-cell death in autoimmune diabetes. Thus, future exploration need focuses on the crosstalk and contribution of both autoimmune attack and intrinsic beta-cell failure to elucidate the identity of autoimmune diabetes and to develop effective prevention and treatment.
Scientists have demonstrated significantly different PI:CP ratio in T1DM patients at onset than healthy control subjects with normal glucose tolerance (27), indicating PI-related measurements could be applicable biomarkers for identifying diabetes patients and evaluating ER stress among them. To further evaluate the possibility of applying PI-related parameters in discriminating forms of diabetes, we performed AUC plots. Though AUCROC of PPI, delta PI and FPI were all reached or nearly reached 0.700, the greatest AUCROC was observed in PPI, indicating higher discrepancies between groups after meal. This was possibly resulted from different ER stress resulted from metabolism stress related to carbohydrates, proteins and fat loads (57,58). Thus, applying PI measurements on discriminating forms of diabetes was applicable, especially after meal loads, but whether a standard meal test is a requirement still need further investigation.
There were several limitations in our study. First, the cross-sectional design restricted the temporal and causal association assessments and the exact molecular mechanism underlying the beta-cell destruction remains unknown. Further, in this study, we did not include a healthy control group with normal glycemic control since former results have already proved different PI secretion pattern and ER stress in diabetes patients compared to healthy individuals. Also, since BMI and age were not perfectly matched among groups, we could not eliminate the influences on PI secretion from either age or BMI. Last, we did not assess possibly related ER stress protein expression and cytokine-related changes in this study, and thus further studies involving other ER stress markers and cytokines are warranted to further explore underneath mechanisms contributing to the different PI secretion patterns among types of diabetes.
Conclusions
To sum up, this study confirmed intermediate serum levels of PI in LADA between T1DM and T2DM, and the fasting PI:CP ratio in T1DM is significantly higher than LADA and T2DM. Positive correlations between PI secretion and islet autoantibody titers and numbers indicated that, in LADA, beta-cell function and ER stress is intimately manipulated by autoimmunity. Our results provided further evidence for the heterogeneity of diabetes, especially autoimmune diabetes. Significantly, we demonstrated that peripheral PI could be applied as a serum biomarker in discriminating different forms of diabetes, which may further assist in precision diagnosis for diabetes.
Acknowledgments
We thank all members in the National Science and Technology Infrastructure Program (2015BAI12B13) from the Department of Metabolism and Endocrinology, The Second Xiangya Hospital of Central South University, Changsha, China, for provision of study material and recruiting patients. We thank Shan Pan, from the Department of Metabolism and Endocrinology, The Second Xiangya Hospital of Central South University, Changsha, China, for assisting provision of study material and recruiting patients. We thank Chuqing Cao from the Department of Metabolism and Endocrinology, The Second Xiangya Hospital of Central South University, Changsha, China, for assisting data analysis and interpretation.
Funding: This work was supported by the National Natural Science Foundation of China (NSFC) [81870577, 81670772 to YX], the Science and Technology Major Project of Hunan Province [2017SK1020 to ZZ], the National Science and Technology Infrastructure Program [2015BAI12B13 to XY] and National Key Research and Development Program of China [2018YFC2001005 to LY].
Footnote
Conflicts of Interest: All authors have completed the ICMJE uniform disclosure form (available at http://dx.doi.org/10.21037/atm.2020.03.189). The authors have no conflicts of interest to declare.
Ethical Statement: The authors are accountable for all aspects of the work in ensuring that questions related to the accuracy or integrity of any part of the work are appropriately investigated and resolved. This study, involving human subjects, human material and human data, was carried out in agreement with the guidelines in the Declaration of Helsinki and approved by the Human Ethics Committee of The Second Xiangya Hospital of Central South University, Changsha, China. All patients in this study have understood and signed the informed consent form. The study outcome will not affect the future management of patients. Patient data in this study were retrieved from the hospital medical record system and patient’s personal data have been secured.
Open Access Statement: This is an Open Access article distributed in accordance with the Creative Commons Attribution-NonCommercial-NoDerivs 4.0 International License (CC BY-NC-ND 4.0), which permits the non-commercial replication and distribution of the article with the strict proviso that no changes or edits are made and the original work is properly cited (including links to both the formal publication through the relevant DOI and the license). See: https://creativecommons.org/licenses/by-nc-nd/4.0/.
References
- Liu B, Xiang Y, Liu Z, et al. Past, present and future of latent autoimmune diabetes in adults. Diabetes Metab Res Rev 2020;36:e3205. [Crossref] [PubMed]
- Mishra R, Hodge KM, Cousminer DL, et al. A Global Perspective of Latent Autoimmune Diabetes in Adults. Trends Endocrinol Metab 2018;29:638-50. [Crossref] [PubMed]
- Yang L, Zhou ZG, Huang G, et al. Six-year follow-up of pancreatic beta cell function in adults with latent autoimmune diabetes. World J Gastroenterol 2005;11:2900-5. [Crossref] [PubMed]
- Buzzetti R, Zampetti S, Maddaloni E.. Adult-onset autoimmune diabetes: current knowledge and implications for management. Nat Rev Endocrinol 2017;13:674-86. [Crossref] [PubMed]
- Sabbah E, Savola K, Ebeling T, et al. Genetic, autoimmune, and clinical characteristics of childhood- and adult-onset type 1 diabetes. Diabetes Care 2000;23:1326-32. [Crossref] [PubMed]
- Hernandez M, Mollo A, Marsal JR, et al. Insulin secretion in patients with latent autoimmune diabetes (LADA): half way between type 1 and type 2 diabetes: action LADA 9. BMC Endocr Disord 2015;15:1. [Crossref] [PubMed]
- Mishra R, Akerlund M, Cousminer DL, et al. Genetic Discrimination Between LADA and Childhood-Onset Type 1 Diabetes Within the MHC. Diabetes Care 2020;43:418-25. [Crossref] [PubMed]
- Cousminer DL, Ahlqvist E, Mishra R, et al. First Genome-Wide Association Study of Latent Autoimmune Diabetes in Adults Reveals Novel Insights Linking Immune and Metabolic Diabetes. Diabetes Care 2018;41:2396-403. [Crossref] [PubMed]
- DiMeglio LA, Evans-Molina C, Oram RA. Type 1 diabetes. Lancet 2018;391:2449-62. [Crossref] [PubMed]
- Jörns AJA, Wedekind DWD, Jahne JJJ, et al. Pancreas Pathology of Latent Autoimmune Diabetes in Adults (LADA) in Patients and in a LADA Rat Model Compared to Type 1 Diabetes Mellitus. Diabetes 2020;69:db190865. [Crossref] [PubMed]
- Arunagiri A, Haataja L, Pottekat A, et al. Proinsulin misfolding is an early event in the progression to type 2 diabetes. Elife 2019;8:e44532. [Crossref] [PubMed]
- Koufakis T, Karras SN, Zebekakis P, et al. Results of the First Genome-Wide Association Study of Latent Autoimmune Diabetes in Adults further highlight the need for a novel diabetes classification system. Ann Transl Med 2018;6:S102. [Crossref] [PubMed]
- Juan-Mateu J, Alvelos MI, Turatsinze JV, et al. SRp55 Regulates a Splicing Network That Controls Human Pancreatic beta-Cell Function and Survival. Diabetes 2018;67:423-36. [Crossref] [PubMed]
- Ghosh R, Colon-Negron K, Papa FR. Endoplasmic reticulum stress, degeneration of pancreatic islet beta-cells, and therapeutic modulation of the unfolded protein response in diabetes. Mol Metab 2019;27S:S60-8. [Crossref] [PubMed]
- Carlsson S.. Etiology and Pathogenesis of Latent Autoimmune Diabetes in Adults (LADA) Compared to Type 2 Diabetes. Front Physiol 2019;10:320. [Crossref] [PubMed]
- Liu M, Wright J, Guo H, et al. Chapter Two - Proinsulin entry and transit through the endoplasmic reticulum in pancreatic beta cells. Vitam Horm 2014;95:35-62. [Crossref] [PubMed]
- Liu M, Weiss MA, Arunagiri A, et al. Biosynthesis, structure, and folding of the insulin precursor protein. Diabetes Obes Metab 2018;20:28-50. [Crossref] [PubMed]
- Verhagen J, Yusuf N, Smith EL, et al. Proinsulin peptide promotes autoimmune diabetes in a novel HLA-DR3-DQ2-transgenic murine model of spontaneous disease. Diabetologia 2019;62:2252-61. [Crossref] [PubMed]
- Wang Y, Sosinowski T, Novikov A, et al. C-terminal modification of the insulin B:11–23 peptide creates superagonists in mouse and human type 1 diabetes. Proc Natl Acad Sci U S A 2018;115:162-7. [Crossref] [PubMed]
- Sims EK, Evans-Molina C, Tersey SA, et al. Biomarkers of islet beta cell stress and death in type 1 diabetes. Diabetologia 2018;61:2259-65. [Crossref] [PubMed]
- Xu B, Allard C, Alvarez-Mercado AI, et al. Estrogens Promote Misfolded Proinsulin Degradation to Protect Insulin Production and Delay Diabetes. Cell Rep 2018;24:181-96. [Crossref] [PubMed]
- Hu H, Peng L, Jiang H, et al. Silenced CHOP protects pancreatic B-cell function by targeting peroxisome proliferator-activated receptor-gamma coactivator-1alpha through nuclear factor-kappaB signaling pathway in diabetes mellitus. J Cell Biochem 2019;120:12595-603. [Crossref] [PubMed]
- Crookshank JA, Serrano D, Wang GS, et al. Changes in insulin, glucagon and ER stress precede immune activation in type 1 diabetes. J Endocrinol 2018;239:181-95. [Crossref] [PubMed]
- Sims EK, Chaudhry Z, Watkins R, et al. Elevations in the Fasting Serum Proinsulin-to-C-Peptide Ratio Precede the Onset of Type 1 Diabetes. Diabetes Care 2016;39:1519-26. [Crossref] [PubMed]
- Van Dalem A, Demeester S, Balti EV, et al. Prediction of Impending Type 1 Diabetes through Automated Dual-Label Measurement of Proinsulin:C-Peptide Ratio. PLoS One 2016;11:e0166702. [Crossref] [PubMed]
- Mezza T, Ferraro PM, Sun VA, et al. Increased beta-Cell Workload Modulates Proinsulin-to-Insulin Ratio in Humans. Diabetes 2018;67:2389-96. [Crossref] [PubMed]
- Watkins RA, Evans-Molina C, Terrell JK, et al. Proinsulin and heat shock protein 90 as biomarkers of beta-cell stress in the early period after onset of type 1 diabetes. Transl Res 2016;168:96-106.e1. [Crossref] [PubMed]
- Marhfour I, Lopez XM, Lefkaditis D, et al. Expression of endoplasmic reticulum stress markers in the islets of patients with type 1 diabetes. Diabetologia 2012;55:2417-20. [Crossref] [PubMed]
- Rodriguez-Calvo T, Zapardiel-Gonzalo J, Amirian N, et al. Increase in Pancreatic Proinsulin and Preservation of beta-Cell Mass in Autoantibody-Positive Donors Prior to Type 1 Diabetes Onset. Diabetes 2017;66:1334-45. [Crossref] [PubMed]
- Truyen I, De Pauw P, Jorgensen PN, et al. Proinsulin levels and the proinsulin:c-peptide ratio complement autoantibody measurement for predicting type 1 diabetes. Diabetologia 2005;48:2322-9. [Crossref] [PubMed]
- Yang Y, Wang M, Tong J, et al. Impaired glucose-stimulated proinsulin secretion is an early marker of beta-cell impairment before prediabetes stage. J Clin Endocrinol Metab 2019;104:4341-6. [Crossref] [PubMed]
- Sims EK, Bahnson HT, Nyalwidhe J, et al. Proinsulin Secretion Is a Persistent Feature of Type 1 Diabetes. Diabetes Care 2019;42:258-64. [Crossref] [PubMed]
- World Health Organization. Definition, diagnosis and classification of diabetes mellitus and its complications: report of a WHO consultation. Part 1, Diagnosis and classification of diabetes mellitus. Geneva: World Health Organization; 1999.
- American Diabetes Association. 2. Classification and Diagnosis of Diabetes: Standards of Medical Care in Diabetes-2019. Diabetes Care 2019;42:S13-S28. [Crossref] [PubMed]
- Stenström G, Gottsäter A, Bakhtadze E, et al. Latent Autoimmune Diabetes in Adults. Diabetes 2005;54:S68-72. [Crossref] [PubMed]
- Maioli M, Pes GM, Delitala G, et al. Number of autoantibodies and HLA genotype, more than high titers of glutamic acid decarboxylase autoantibodies, predict insulin dependence in latent autoimmune diabetes of adults. Eur J Endocrinol 2010;163:541-9. [Crossref] [PubMed]
- Cao ZH, Wu Z, Hu C, et al. Endoplasmic reticulum stress and destruction of pancreatic beta cells in type 1 diabetes. Chin Med J (Engl) 2020;133:68-73. [Crossref] [PubMed]
- Junjappa RP, Patil P, Bhattarai KR, et al. IRE1alpha Implications in Endoplasmic Reticulum Stress-Mediated Development and Pathogenesis of Autoimmune Diseases. Front Immunol 2018;9:1289. [Crossref] [PubMed]
- Engin F, Yermalovich A, Nguyen T, et al. Restoration of the unfolded protein response in pancreatic beta cells protects mice against type 1 diabetes. Sci Transl Med 2013;5:214er11. [Crossref] [PubMed]
- Morita S, Villalta SA, Feldman HC, et al. Targeting ABL-IRE1alpha Signaling Spares ER-Stressed Pancreatic beta Cells to Reverse Autoimmune Diabetes. Cell Metab 2017;25:883-97.e8. [Crossref] [PubMed]
- Kim MJ, Min SH, Shin SY, et al. Attenuation of PERK enhances glucose-stimulated insulin secretion in islets. J Endocrinol 2018;236:125-36. [Crossref] [PubMed]
- Patel K, Levesque K, Mark V, et al. Proinsulin associates with poor beta-cell function, glucose-dependent insulinotropic peptide, and insulin resistance in persistent type 2 diabetes after Roux-en-Y gastric bypass in humans. J Diabetes 2020;12:77-86. [Crossref] [PubMed]
- Vauhkonen I, Leo N, Mikael K, et al. Subtle hyperproinsulinaemia characterises the defective insulin secretory capacity in offspring of glutamic acid decarboxylase antibody-positive patients with latent autoimmune diabetes mellitus in adults. Eur J Endocrinol 2005;153:265-73. [Crossref] [PubMed]
- Castelblanco E, Hernandez M, Castelblanco A, et al. Low-grade Inflammatory Marker Profile May Help to Differentiate Patients With LADA, Classic Adult-Onset Type 1 Diabetes, and Type 2 Diabetes. Diabetes Care 2018;41:862-8. [Crossref] [PubMed]
- Gudipaty L, Rosenfeld NK, Fuller CS, et al. Different beta-cell secretory phenotype in non-obese compared to obese early type 2 diabetes. Diabetes Metab Res Rev 2020. [Epub ahead of print]. [Crossref] [PubMed]
- Juusola M, Parkkola A, Härkönen T, et al. Positivity for Zinc Transporter 8 Autoantibodies at Diagnosis Is Subsequently Associated With Reduced β-Cell Function and Higher Exogenous Insulin Requirement in Children and Adolescents With Type 1 Diabetes. Diabetes Care 2016;39:118. [Crossref] [PubMed]
- Sorensen JS, Vaziri-Sani F, Maziarz M, et al. Islet autoantibodies and residual beta cell function in type 1 diabetes children followed for 3-6 years. Diabetes Res Clin Pract 2012;96:204-10. [Crossref] [PubMed]
- Jacobsen LM, Bocchino L, Evans-Molina C, et al. The risk of progression to type 1 diabetes is highly variable in individuals with multiple autoantibodies following screening. Diabetologia 2020;63:588-96. [Crossref] [PubMed]
- Williams CL, Long AE. What has zinc transporter 8 autoimmunity taught us about type 1 diabetes? Diabetologia 2019;62:1969-76. [Crossref] [PubMed]
- Smith JA. Regulation of Cytokine Production by the Unfolded Protein Response; Implications for Infection and Autoimmunity. Front Immunol 2018;9:422. [Crossref] [PubMed]
- Brozzi F, Nardelli TR, Lopes M, et al. Cytokines induce endoplasmic reticulum stress in human, rat and mouse beta cells via different mechanisms. Diabetologia 2015;58:2307-16. [Crossref] [PubMed]
- Thayer TC, Pearson JA, De Leenheer E, et al. Peripheral Proinsulin Expression Controls Low-Avidity Proinsulin-Reactive CD8 T Cells in Type 1 Diabetes. Diabetes 2016;65:3429-39. [Crossref] [PubMed]
- Thomaidou S, Zaldumbide A, Roep BO. Islet stress, degradation and autoimmunity. Diabetes Obes Metab 2018;20 Suppl 2:88-94. [Crossref] [PubMed]
- Marré ML, Profozich JL, Coneybeer JT, et al. Inherent ER stress in pancreatic islet beta cells causes self-recognition by autoreactive T cells in type 1 diabetes. J Autoimmun 2016;72:33-46. [Crossref] [PubMed]
- Mannering SI, Harrison LC, Williamson NA, et al. The insulin A-chain epitope recognized by human T cells is posttranslationally modified. J Exp Med 2005;202:1191-7. [Crossref] [PubMed]
- van Lummel M, Duinkerken G, van Veelen PA, et al. Posttranslational modification of HLA-DQ binding islet autoantigens in type 1 diabetes. Diabetes 2014;63:237-47. [Crossref] [PubMed]
- Cunha DA, Hekerman P, Ladriere L, et al. Initiation and execution of lipotoxic ER stress in pancreatic beta-cells. J Cell Sci 2008;121:2308-18. [Crossref] [PubMed]
- Ghemrawi R, Battaglia-Hsu SF, Arnold C. Endoplasmic Reticulum Stress in Metabolic Disorders. Cells 2018;7:63. [Crossref] [PubMed]