Wogonoside inhibits inflammatory cytokine production in lipopolysaccharide-stimulated macrophage by suppressing the activation of the JNK/c-Jun signaling pathway
Introduction
Inflammation is an underlying presence in the pathogenesis of various pulmonary diseases, including sepsis, acute lung injury (ALI), chronic obstructive pulmonary disease (COPD), cystic fibrosis, and asthma (1,2). Pulmonary inflammation arises following exposure to pathogens, pollutants, allergens, irritants, and other detrimental microorganisms (3,4). The initial response to infection is mainly mediated by macrophages, which recruit leukocytes to the lung tissues and induce the production of a series of inflammatory factors, such as cyclooxygenase-2 (COX-2), inducible nitric oxide synthase (iNOS), interleukin (IL)-1β, tumor necrosis factor (TNF)-α, and IL-6 (5,6).
The activation of macrophages is carried out by pattern recognition receptors (PRRs), which recognize extracellular antigens (7,8). After binding to toll-like receptor 4 (TLR4), lipopolysaccharide (LPS) recruits the cytoplasmic adaptor proteins, before activating nuclear factor-κB (NF-κB) and three well-defined mitogen-activated protein kinase (MAPK) signaling pathways (the c-Jun N-terminal kinase (JNK), p38 MAPK, and extracellular signal-regulated kinase 1/2 (ERK1/2) pathways), which ultimately results in inflammation (9,10). After the NF-κB and MAPK signaling pathways are activated, the downstream transcription factor NF-κB and activator protein-1 (AP-1) translocate to the nucleus, and promote pro-inflammatory cytokine expression (11,12).
c-Jun is an important member of the AP-1 transcription factor family, whose activation contributes to the expression of associated inflammatory genes in macrophages (13,14). Therefore, therapeutic interventions that target the inflammatory response of macrophages and the activation of associated signaling pathways may provide a novel alternative strategy for managing pulmonary inflammatory diseases.
Due to its therapeutic effects and relatively low toxicity, the active component of herbal medicine has received considerable attention (15). The anti-inflammatory, anti-allergic and immunomodulatory effects exerted by flavonoids have been evidenced by a growing number of studies (16,17). Wogonoside, a metabolite of wogonin, is a bioactive flavonoid derived from the root of Scutellaria baicalensis (18). Emerging evidence has shown that wogonoside mitigates spinal cord injury (SCI)-induced inflammation and provides protection against experimental colitis induced by dextran sulfate sodium (DSS) (19,20). Furthermore, wogonoside has been verified to alleviate LPS-induced ALI by blocking the release of the inflammatory cytokines (21,22). However, the mechanism underlying the anti-inflammatory influence of wogonoside remains unclear. This study aimed to uncover more about the effects of wogonoside on LPS-stimulated production of inflammatory factors and to determine its value as a potential therapy for pulmonary diseases.
Methods
Materials
Wogonoside (Wog) was acquired from Biopurify Phytochemicals Ltd. (Sichuan, China). LPS (0111:B4) was acquired from Sigma-Aldrich (Shanghai, China). Griess reagent was supplied by Promega Biotech Co (Madison, WI, USA), and TRIzol reagent was acquired from Invitrogen Co. (Carlsbad, CA, USA). Dichlorofluorescein diacetate (DCFH-DA) was obtained from Solarbio Technology Inc. (Beijing, China). RT SuperMix and Universal SYBR qPCR Master were both supplied by Vazyme Biotech (Nanjing, China). SP600125, brefeldin A, and CCK-8 were obtained from Selleck Chemicals (Houston, USA). An ECL kit and PVDF membrane were purchased from Millipore Company (Shanghai, China). The anti-COX-2 antibody was purchased from Proteintech (Wuhan, China). The anti-IL-1β, anti-phospho-p65, anti-TNF-α, anti-p65, anti-IL-6 antibodies, anti-phospho-MAPK family, and anti-MAPK family antibody samplers were supplied by Cell Signaling Technology, Inc. (Boston, MA, USA). HRP-goat anti-rabbit/mouse IgG antibody, anti-c-Jun, and anti-iNOS antibodies were obtained from Abcam (Cambridge, MA, USA). GAPDH antibody was acquired from TransGen Biotech (Beijing, China). Alexa Fluor 488-labeled and 594-labeled secondary antibodies were purchased from Invitrogen (Shanghai, China).
Cell culture and drug treatments
RAW264.7 cells were purchased from the Shanghai Institute of Cell Biology. The cells were grown in complete DMEM medium containing 10% FBS and 1% antibiotics under an incubator with 5% CO2 at 37 °C, and were treated with wogonoside (25, 50, or 100 µM) or SP600125 (20 µM) 2 h prior to stimulation with 1 µg/mL LPS.
Animal experiments
Six-to-seven-week-old BALB/c mice were supplied by Guangzhou Experimental Animal Center (Guangzhou, China). All mice were kept in cages at 22±1 °C with access to food and water. All of the experiments in this study received approval from the Animal Ethics Committees of Jinan University and were performed according to the recommendations of the Guide for the Care and Use of Laboratory Animals of Jinan University.
Isolation of murine alveolar macrophage (AM)
AMs were obtained from bronchoalveolar lavage fluid (BALF) as previously reported (23). In brief, the mice were anesthetized with pentobarbital sodium, the upper trachea was cannulated, and BALF was collected by washing with sterilized phosphate buffer solution (PBS). The BALF was centrifuged at 800 ×g at 4 °C for 10 min to acquire the sedimented cells. Red blood cells (RBCs) were removed using RBC lysis. Subsequently, the cells were repelleted and resuspended in DMEM medium. One surface antigen (F4/80) and an intracellular antigen (CD68) were used to identify the AM population.
Cell viability assay
The cells were cultured in 96-well plates, before incubation with various concentrations of wogonoside for a period of 24 h. The effect of wogonoside on cell viability was determined with CCK-8 assay, as previously reported (24).
Nitric oxide (NO) assay
RAW264.7 cells (2×105 cells/mL) were cultured in 24-well plates and incubated with either SP600125 at 20 µM or wogonoside at 25, 50, or 100 µM for 2 h, and then exposed to LPS for a further 24 h. Griess reagent was utilized to quantify nitric oxide formation, as previously reported (25).
Reactive oxygen species (ROS) analysis
RAW264.7 cells in 6-well plates at 1×106 cells per well were pre-treated with either wogonoside or SP600125 (at the concentrations mentioned above) for 2 h and subsequently exposed to LPS for 6 h. After incubation with DCFH-DA (2 µM) for 30 min, ROS was determined with the FITC channel using Beckman CytoFLEX FCM (Beckman Coulter, USA) (26).
Western blot analysis
To determine cytokine expression using Western blot, brefeldin A (500 ng/mL) was added for 3 h before protein extraction to block the protein from translocating from the endoplasmic reticulum to the cell outside (27). Total protein was obtained from RAW264.7 cells with lysis buffer containing protease and phosphatase inhibitor. Protein samples were loaded per lane, fractionated by 4–20% gradient SDS-PAGE precast gel and then electrotransferred onto PVDF membranes. Then, the membranes were incubated with the primary and HRP-conjugated second antibody after blocking with 5% skim milk (28). Protein bands were visualized with enhanced ECL reagent and determined using Syngene GNOME XRQ NPC (Syngene, Cambridge, UK).
Quantitative real-time PCR
The cells were seeded in 6-well plates at a density of 1×106 and incubated with either SP600125 or wogonoside at different concentrations for 2 h, before exposure to LPS for 2 or 6 h. Total RNA was extracted from cells with TRIzol reagent. Then, RNA was reverse-transcribed into cDNAs. PCR amplification was carried out with the Bio-Rad CFX Connect using SYBR qPCR Master Mix (29). Primers were obtained from Sangon Biotech (Shanghai, China). The following primer sequences were used in the experiments: COX-2-F: TGAGCAACTATTCCAAACCAGC, COX-2-R: GCACGTAGTCTTCGATCACTATC, iNOS-F: GGCAGCCTGTGAGACCTTTG, iNOS-R: GCATTGGAAGTGAAGCGTTTC, IL-1β-F: CAGAGTTCCCCAACTGGTACATC, IL-1β-R: GGGAAGGCATTAGAAACAGTCC, TNF-α-F: GCTCTGTGAAGGGAATGGGTGTT, TNF-α-R: GTCCAGGTCACTGTCCCAGCATC, IL-6-F: ACTTCCATCCAGTTGCCTTCTTG, IL-6-R: TGTTGGGAGTGGTATCCTCTGTG, GAPDH-F: AGGTCGGTGTGAACGGATTTG, GAPDH-R: TGTAGACCATGTAGTTGAGGTCA
Immunofluorescence analysis
AMs (2×104 cells/mL) were cultured in 48-well plates before incubation with wogonoside for 2 h and exposure to LPS for 6 h. The cells were then washed with PBS, fixed with 4% cold paraformaldehyde for 30 min, permeabilized with 0.1% Triton X-100 for 15 min before blocking with 3% BSA, and incubated with a primary antibody against TNF-α, CD68, or F4/80 overnight. Then, the cells were incubated again with Alexa Fluor 488 or 594-conjugated secondary antibody for 2 h. The samples were observed using an inverted fluorescence microscope (DMi8 manual, Leica, Germany) (26).
ChIP assay
The cells were seeded at a density of 1×107 in a 10 cm-dish, incubated with wogonoside for 2 h, and then exposed to LPS for another 30 min. The cells were fixed with 1% formaldehyde for 10 min at RT and harvested with ChIP lysis buffer (Invitrogen, Carlsbad, CA, USA). Lysed cells were sonicated and lysates were immunoprecipitated with an anti-c-Jun antibody overnight at 4 °C. Protein A/G plus magnetic beads (Invitrogen, Carlsbad, CA) were used to capture the protein-antibody complex for 3 h at 4 °C. After washing, the DNA was purified by phenol-chloroform in the presence of 1 µg glycine after reverse-crosslinking for 12 h at 65 °C and the proteins were removed with proteinase K (30). Immunoprecipitated DNA was analyzed by qPCR. The following primer sequences were used in the experiment: COX-2-F: GAGCAGGGGTCTTGCTGTGTTAC, COX-2-R: CTGTACGTTCTTGGCGTGAATGGAT, iNOS-F: CACCTAACCTCCAGGCCCATGG, iNOS-R: GGCTTGTGTAGCACCCACAACCC, IL-1β-F: GTCCAGGGGGAAATAATGCCCATTTC, IL-1β-R: GGAGAAGCTTGATGGGAATTCTTAGG, TNF-α-F: TCTCCGCTGCCAGGACAGCC, TNF-α-R: TGGGCAAGTAGGACAAAAGGCAGG, IL-6-F: CTGGAGACAGGTGGACAGAAAACC, IL-6-R: CTGAAGGTGGTACGTCTCACTCTG
Statistical analysis
The results in the bar graphs are shown as the mean ± standard error of the mean (SEM), based on at least three independent experiments. All data were analyzed with a one-way ANOVA followed by Dunnett’s multiple comparison test using GraphPad Prism 6.0 software. Image J quantified the average intensity of fluorescence. P<0.05 indicated a statistically significant difference.
Results
Wogonoside inhibited inflammatory cytokine expression in LPS-stimulated macrophages
Immunofluorescence was performed to identify and characterize the primary AM. As shown in Figure 1A, the cells isolated from the mouse lung BALF had high expressions of the macrophage-specific markers F4/80 and CD68. The Western blot analysis showed that following pre-treatment with wogonoside, the expression levels of TNF-α and IL-1β were remarkably decreased in AMs after LPS exposure (Figure 1B,C). Meanwhile, immunofluorescence analysis also confirmed that wogonoside significantly suppressed TNF-α expression after LPS-induced activation of AMs (Figure 1D,E).
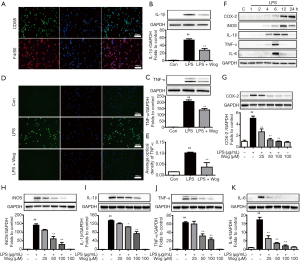
As well as analyzing the effects of wogonoside on AMs, the macrophage cell line RAW264.7 was utilized to further investigate its anti-inflammatory role and mechanism. Before the study, the effect of wogonoside on cell viability was checked at concentrations of 25 to 200 µM. As illustrated in Figure S1A, the highest concentration of wogonoside did not impact cell viability. Wogonoside significantly countered LPS-induced increases in nitric oxide (NO) release and ROS production in a concentration-dependent manner (Figure S1B,C,D). The time course of inflammatory cytokine expression induced by LPS was determined using Western blot. After 6 h, the expressions of IL-6, IL-1β, and TNF-α had been significantly enhanced by the addition of LPS to the culture medium, while after 12 h, iNOS and COX-2 were significantly upregulated (Figure 1F). Meanwhile, wogonoside dramatically inhibited LPS-induced protein expression of IL-1β, iNOS, TNF-α, COX-2, and IL-6 in a concentration-dependent manner (Figure 1G,H,I,J,K). mRNA levels of the aforementioned inflammatory factors were also remarkably decreased by wogonoside in LPS-exposed RAW264.7 cells. (Figure S2A,B,C,D,E).
Wogonoside inhibited LPS-induced phosphorylation of JNK in macrophages
To further explore how wogonoside decreases the expression of inflammatory factors in LPS-stimulated macrophages, we determined the phosphorylation of p65, p38, ERK, and JNK. As indicated in Figure 2A, the phosphorylation of p65, p38, ERK and JNK were observably increased in a time-dependent manner after LPS stimulation and reached a peak at 30 min. Wogonoside barely affected the phosphorylation of p65 and partially reduced the phosphorylation of p38 and ERK in LPS-exposed RAW264.7 cells (Figure 2B,C,D). However, wogonoside dramatically suppressed phosphorylation of JNK in LPS-exposed RAW264.7 cells and AMs (Figure 2E,F).
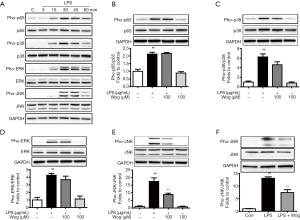
JNK inhibitor SP600125 suppressed NO release and ROS production in LPS-stimulated macrophages
Due to the difficulty in acquiring a larger number of AMs, further experiments were only conducted using the RAW264.7 cell line. To further explore the potential suppressive effect of wogonoside on LPS-induced increases in NO release and ROS production, which mainly occurs via JNK signaling, a specific inhibitor of JNK, SP600125, was utilized. As shown in Figure 3A,B,C, SP600125 alone significantly suppressed the LPS-induced increases in NO release and ROS production. The combination of wogonoside and SP600125 was no more effective at decreasing LPS-stimulated NO release and ROS production than wogonoside alone. However, treatment with wogonoside alone resulted in stronger inhibition of NO compared with SP600125 treatment in LPS-exposed RAW264.7 cells (Figure 3A).
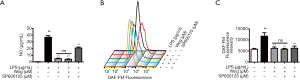
JNK inhibitor SP600125 suppressed protein expression of inflammatory factors in LPS-stimulated macrophages
We further investigated if wogonoside inhibits LPS-induced pro-inflammatory cytokine expression principally via JNK signaling. As illustrated in Figure 4A,B,C,D,E,F, LPS-induced phosphorylation of JNK and iNOS, COX-2, IL-1β, TNF-α, and IL-6 protein expression were significantly decreased after pre-treatment with SP600125 alone. Compared to wogonoside, a combination of wogonoside and SP600125 was found to have a stronger inhibitory effect on JNK phosphorylation in LPS-stimulated RAW264.7 cells. However, there were no significant differences in the suppression of iNOS, COX-2, IL-1β, TNF-α, and IL-6 protein expression between the wogonoside and combined groups. These data indicated that the suppression of JNK activation seen with wogonoside makes a substantial contribution to blocking the expression of the inflammatory factors. Additionally, SP600125 treatment partially suppressed iNOS expression, while wogonoside largely abolished iNOS expression (Figure 4B).
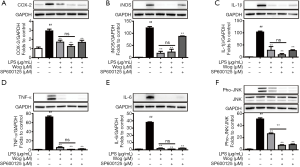
JNK inhibitor SP600125 suppressed mRNA levels of inflammatory factors in LPS-stimulated macrophages
To further confirm the transcriptional inhibitory property of wogonoside, we performed qPCR and found that SP600125 also significantly reduced the LPS-induced mRNA levels of iNOS, COX-2, IL-1β, TNF-α, and IL-6. Consistent with the Western blot analysis above, the inhibitory effect of wogonoside on the mRNA levels of the aforementioned inflammatory cytokines following LPS stimulation was almost equivalent to that of combined wogonoside and SP600125 (Figure 5A,B,C,D,E). Moreover, in comparison to wogonoside, SP600125 did not significantly suppress the level of iNOS mRNA, which indicated that there might be some other signaling pathways involved in the suppressive influence of wogonoside on iNOS expression and NO release (Figure 5B).
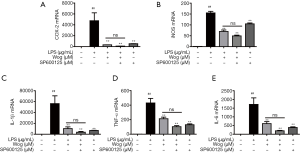
Wogonoside decreased c-Jun deposition at pro-inflammatory cytokine promoters in LPS-stimulated macrophages
We further explored the effect of wogonoside in suppressing the increase of pro-inflammatory cytokines at the mRNA level following LPS exposure. c-Jun, a JNK substrate, was investigated using ChIP analysis. After 30 min of LPS stimulation, binding between c-Jun and inflammatory factor promoters was significantly increased (Figure 6A,B,C,D,E). Pre-treatment with wogonoside observably reduced c-Jun enrichment in the promoters of iNOS, COX-2, IL-1β, TNF-α, and IL-6 in LPS-stimulated RAW264.7 cells.
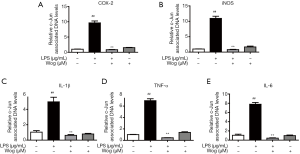
Discussion
In this study, we demonstrated that wogonoside significantly hampered the LPS-induced expression of inflammatory factors in AMs and RAW264.7 cells. Furthermore, we found that wogonoside lessened the expression of inflammatory factors by blocking JNK phosphorylation and c-Jun enrichment in pro-inflammatory cytokine promoters. Additionally, when used in combination, wogonoside and SP600125 offered no added benefit in preventing the expression of inflammatory factors. The above results indicate that wogonoside’s inhibitive effect on JNK/c-Jun signaling activation contributed to the suppression of the inflammatory factors produced in LPS-stimulated macrophages.
Respiratory tract inflammation is involved in the pathological processes of pulmonary diseases (1). The macrophages in the lung are the first line of defense in immune response against infections (6). According to their response to cytokines, macrophages are categorized as either classically activated (M1 subtype) or alternatively activated (M2 subtype) macrophages. M1 macrophages are typically induced by LPS, interferon (IFN)-γ, and TNF-α, while M2 macrophages are activated by IL-13 and IL-4 (31). Excessive activation of M1 macrophages contributes to the development of inflammatory diseases by upregulating the expression of inflammatory factors (32). Wogonoside has been reported to suppress the release of the pro-inflammatory cytokines IL-6, IL-1β, and TNF-α in the LPS-induced ALI model (21). However, the specific mechanism and targeted cells that exert anti-inflammatory effects in response to wogonoside in pulmonary inflammatory diseases have not yet been established.
In our study, we showed that wogonoside reduced the elevation of M1-associated genes and protein expression in LPS-stimulated RAW264.7 cells and AMs (Figures 1,S1,S2), suggesting that wogonoside suppresses the release of inflammatory factors by hampering M1-like macrophages activation, thus protecting the lung from injury.
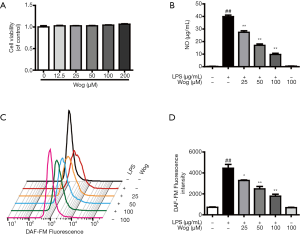
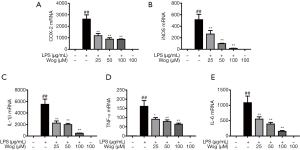
The NF-κB signaling pathway has been demonstrated to be closely associated with the inflammatory response (9). Degradation of phosphorylated IκBα increases the phosphorylation of NF-κB p65, triggering the activation of various inflammation-associated genes (26). Recently, Sun’s study revealed that wogonoside inhibited the phosphorylation of NF-κB p65 in lung and colonic tissues (20). Therefore, based on previous research, the effect of wogonoside on NF-κB p65 phosphorylation in macrophages stimulated by LPS was investigated. However, our results showed that wogonoside did not affect LPS-induced NF-κB p65 phosphorylation in macrophages (Figure 2B). One possible explanation for this could be that wogonoside might pause microenvironment-driven NF-κB activation.
MAPKs also play a significant role in regulating inflammation (33). Macrophage exposure to LPS has been well-known to activate the p38, ERK1/2, and JNK MAPKs signaling pathways (10). Wogonoside has been reported to inhibit LPS-induced angiogenesis by suppressing MAPK activation (34). Based on previous reports, we next investigated whether wogonoside exhibits an anti-inflammatory effect via the MAPK pathways. Our results demonstrated that wogonoside remarkably suppressed the LPS-stimulated increase of JNK phosphorylation in RAW264.7 cells and AMs (Figure 2E,F). However, wogonoside had a little effect on the phosphorylation of p38 and ERK (Figure 2C,D).
It is well-known that the upstream of JNK are MAPK kinases 4 (MKK4) and MKK7, which activate JNK via phosphorylation of its threonine and tyrosine residues (35). The activation of MKK4 and MKK7 is mediated via phosphorylation on two serine residues by MAPK kinases kinase (33). Moreover, knockdown of MKK4 has been shown to block the release of IL-6 and TNF-α by inhibiting the phosphorylation of JNK in LPS-stimulated RAW264.7 cells (36). Another study demonstrated that the de-SUMOylation of MKK7 increased inflammatory cytokine production induced by LPS in macrophages by potentiating the phosphorylation of JNK (37). According to the literature mentioned above, we speculated that wogonoside might inhibit JNK phosphorylation by regulating the phosphorylation, SUMOylation or expression of MKK4 and MKK7 in LPS-stimulated macrophages.
To further confirm the role of JNK in the anti-inflammatory activity of wogonoside, the JNK inhibitor SP600125 was used. JNK inhibitor SP600125 has been reported to notably reduce IL-6 and TNF-α in BALF from ALI mice (38). In our studies, SP600125 treatment alone significantly reduced NO release and ROS production, and also relieved the augmentation of protein and mRNA levels of inflammatory factors after LPS stimulation. This indicated that inhibition of JNK phosphorylation could exert an anti-inflammatory effect (Figures 3-5). Moreover, in comparison to wogonoside, SP600125 treatment alone exhibited stronger inhibition of JNK phosphorylation but weaker inhibition of NO release and iNOS expression (Figures 3A,4B,4F,5B). Meanwhile, wogonoside partially prevented the phosphorylation of p38 and ERK (Figure 2C,D). Taken together, this indicates that, besides JNK, wogonoside may regulate NO and iNOS production via the p38 and ERK pathways. Compared with wogonoside alone, the combination of wogonoside with SP600125 notably inhibited the phosphorylation of JNK. However, there was no significant difference between wogonoside and a combination of wogonoside with SP600125 in suppressing the increase in the protein and mRNA levels of the inflammatory factors. Our results indicated that wogonoside remarkably suppresses the expression of inflammatory factors mainly via blocking JNK activation.
c-Jun, a substrate of JNK, is a critical regulator of the transcription factor AP-1 complex (39). Activation of JNK could phosphorylate c-Jun on residues Ser63 and Ser73 and then inhibit the ubiquitination and degradation of c-Jun (40). Recruitment of c-Jun to specific DNA sequences located in promoters induces the expression of its downstream-regulated inflammatory genes, resulting in the subsequent inflammatory response (13,41). Previous findings have shown that c-Jun deposition at promoters of inflammatory cytokines (PTGS2, IL-6, TNF-α) and chemokines (CCL20, CCL4, CCL3) was significantly increased at 30 min after LPS stimulation in primary monocytes (30). Hsieh’s research found that the binding of the activated c-Jun with the COX-2 promoter led to COX-2 gene transcription (42). According to the above literature, we determined whether wogonoside downregulated the expression of inflammatory factors via the modulation of the c-Jun recruitment to the pro-inflammatory cytokine promoters. ChIP analysis showed that pre-treatment of wogonoside remarkably reduced the binding affinity of c-Jun to promoters of iNOS, COX-2 IL-1β, IL-6, and TNF-α in LPS-stimulated macrophages (Figure 6). Zhu’s observations that flavonoid compound luteolin decreased IL-6 production by suppressing JNK phosphorylation and AP-1 activation in LPS-induced microglia and had no effect on the binding activity of NF-κB and IL-6 promoters were also partly consistent with those of our studies (43). Our data demonstrated that wogonoside suppressed LPS-induced macrophage inflammatory response mainly through the inhibition of the JNK/c-Jun signaling pathway.
Conclusions
In summary, our results indicate that pre-treatment with wogonoside significantly represses LPS-induced increases in the expression and release of inflammatory factors by macrophages, primarily by inactivating of the JNK/c-Jun signaling pathways. Our findings show that wogonoside is a potential therapeutic agent against macrophage-associated pulmonary diseases.
Acknowledgments
Funding: This work was supported by the National Natural Science Foundation of China (NSFC:81770028), the Natural Science Foundation of Guangdong (2018A030310011), the Project of Shenzhen Basic Research Plan (JCYJ20170307095633450), the Clinical Research of Health and Family Planning Commission of Shenzhen Municipality (SZLY2017024), and the Key Laboratory of Shenzhen Respiratory Diseases (ZDSYS201504301616234).
Footnote
Conflicts of Interest: All authors have completed the ICMJE uniform disclosure form (available at http://dx.doi.org/10.21037/atm.2020.04.22). The authors have no conflicts of interest to declare.
Ethical Statement: The authors are accountable for all aspects of the work in ensuring that questions related to the accuracy or integrity of any part of the work are appropriately investigated and resolved. All experiments were approved by the Animal Ethics Committees of Jinan University (No. 20180226031) and performed in adherence with the Guide for the Care and Use of Laboratory Animals of Jinan University.
Open Access Statement: This is an Open Access article distributed in accordance with the Creative Commons Attribution-NonCommercial-NoDerivs 4.0 International License (CC BY-NC-ND 4.0), which permits the non-commercial replication and distribution of the article with the strict proviso that no changes or edits are made and the original work is properly cited (including links to both the formal publication through the relevant DOI and the license). See: https://creativecommons.org/licenses/by-nc-nd/4.0/.
References
- Racanelli AC, Kikkers SA, Choi AMK, et al. Autophagy and inflammation in chronic respiratory disease. Autophagy 2018;14:221-32. [Crossref] [PubMed]
- Yamasaki K, Eeden SFV. Lung Macrophage Phenotypes and Functional Responses: Role in the Pathogenesis of COPD. Int J Mol Sci 2018;19:582. [Crossref] [PubMed]
- D'Amato G, Vitale C, Lanza M, et al. Climate change, air pollution, and allergic respiratory diseases: an update. Curr Opin Allergy Clin Immunol 2016;16:434-40. [Crossref] [PubMed]
- Yao QW, Wang XY, Li JC, et al. Ophiopogon japonicus inhibits radiation-induced pulmonary inflammation in mice. Ann Transl Med 2019;7:622. [Crossref] [PubMed]
- Shapouri-Moghaddam A, Mohammadian S, Vazini H, et al. Macrophage plasticity, polarization, and function in health and disease. J Cell Physiol 2018;233:6425-40. [Crossref] [PubMed]
- Puttur F, Gregory LG, Lloyd CM. Airway macrophages as the guardians of tissue repair in the lung. Immunol Cell Biol 2019;97:246-57. [Crossref] [PubMed]
- Rosadini CV, Kagan JC. Early innate immune responses to bacterial LPS. Curr Opin Immunol 2017;44:14-9. [Crossref] [PubMed]
- Hamidzadeh K, Christensen SM, Dalby E, et al. Macrophages and the Recovery from Acute and Chronic Inflammation. Annu Rev Physiol 2017;79:567-92. [Crossref] [PubMed]
- Liu T, Zhang L, Joo D, et al. NF-kappaB signaling in inflammation. Signal Transduct Target Ther 2017;2:17023. [Crossref] [PubMed]
- Lloberas J, Valverde-Estrella L, Tur J, et al. Mitogen-Activated Protein Kinases and Mitogen Kinase Phosphatase 1: A Critical Interplay in Macrophage Biology. Front Mol Biosci 2016;3:28. [Crossref] [PubMed]
- Park J, Kwak CH, Ha SH, et al. Ganglioside GM3 suppresses lipopolysaccharide-induced inflammatory responses in rAW 264.7 macrophage cells through NF-kappaB, AP-1, and MAPKs signaling. J Cell Biochem 2018;119:1173-82. [Crossref] [PubMed]
- McNelis JC, Olefsky JM. Macrophages, immunity, and metabolic disease. Immunity 2014;41:36-48. [Crossref] [PubMed]
- Hannemann N, Jordan J, Paul S, et al. The AP-1 Transcription Factor c-Jun Promotes Arthritis by Regulating Cyclooxygenase-2 and Arginase-1 Expression in Macrophages. J Immunol 2017;198:3605-14. [Crossref] [PubMed]
- Yang J, Huang M, Zhou L, et al. Cereblon suppresses the lipopolysaccharide-induced inflammatory response by promoting the ubiquitination and degradation of c-Jun. J Biol Chem 2018;293:10141-57. [Crossref] [PubMed]
- Arulselvan P, Fard MT, Tan WS, et al. Role of Antioxidants and Natural Products in Inflammation. Oxid Med Cell Longev 2016;2016:5276130.
- Leyva-López N, Gutierrez-Grijalva EP, Ambriz-Perez DL, et al. Flavonoids as Cytokine Modulators: A Possible Therapy for Inflammation-Related Diseases. Int J Mol Sci 2016;17:921. [Crossref] [PubMed]
- Gandhi GR, Neta M, Sathiyabama RG, et al. Flavonoids as Th1/Th2 cytokines immunomodulators: A systematic review of studies on animal models. Phytomedicine 2018;44:74-84. [Crossref] [PubMed]
- Chen Y, Hui H, Yang H, et al. Wogonoside induces cell cycle arrest and differentiation by affecting expression and subcellular localization of PLSCR1 in AML cells. Blood 2013;121:3682-91. [Crossref] [PubMed]
- Zhu Y, Zhu H, Wang Z, et al. Wogonoside alleviates inflammation induced by traumatic spinal cord injury by suppressing NF-kappaB and NLRP3 inflammasome activation. Exp Ther Med 2017;14:3304-8. [Crossref] [PubMed]
- Sun Y, Zhao Y, Yao J, et al. Wogonoside protects against dextran sulfate sodium-induced experimental colitis in mice by inhibiting NF-kappaB and NLRP3 inflammasome activation. Biochem Pharmacol 2015;94:142-54. [Crossref] [PubMed]
- Zhang L, Ren Y, Yang C, et al. Wogonoside ameliorates lipopolysaccharide-induced acute lung injury in mice. Inflammation 2014;37:2006-12. [Crossref] [PubMed]
- Wei M, Xie X, Chu X, et al. Dihydroartemisinin suppresses ovalbumin-induced airway inflammation in a mouse allergic asthma model. Immunopharmacol Immunotoxicol 2013;35:382-9. [Crossref] [PubMed]
- Zhang X, Goncalves R, Mosser DM. The isolation and characterization of murine macrophages. Curr Protoc Immunol 2008;Chapter 14:Unit-14.1.
- Ryu HW, Lee SU, Lee S, et al. 3-Methoxy-catalposide inhibits inflammatory effects in lipopolysaccharide-stimulated RAW264.7 macrophages. Cytokine 2017;91:57-64. [Crossref] [PubMed]
- Zhang Y, Guo H, Cheng BC, et al. Dingchuan tang essential oil inhibits the production of inflammatory mediators via suppressing the IRAK/NF-kappaB, IRAK/AP-1, and TBK1/IRF3 pathways in lipopolysaccharide-stimulated RAW264.7 cells. Drug Des Devel Ther 2018;12:2731-48. [Crossref] [PubMed]
- Yuan R, Huang L, Du LJ, et al. Dihydrotanshinone exhibits an anti-inflammatory effect in vitro and in vivo through blocking TLR4 dimerization. Pharmacol Res 2019;142:102-14. [Crossref] [PubMed]
- Jiang H, Khan S, Wang Y, et al. SIRT6 regulates TNF-alpha secretion through hydrolysis of long-chain fatty acyl lysine. Nature 2013;496:110-3. [Crossref] [PubMed]
- Somensi N, Rabelo TK, Guimaraes AG, et al. Carvacrol suppresses LPS-induced pro-inflammatory activation in RAW 264.7 macrophages through ERK1/2 and NF-kB pathway. Int Immunopharmacol 2019;75:105743. [Crossref] [PubMed]
- Qin Y, Sun X, Shao X, et al. Lipopolysaccharide Preconditioning Induces an Anti-inflammatory Phenotype in BV2 Microglia. Cell Mol Neurobiol 2016;36:1269-77. [Crossref] [PubMed]
- Maurer K, Ramen S, Shi L, et al. Rapid induction of expression by LPS is accompanied by favorable chromatin and rapid binding of c-Jun. Mol Immunol. 2018;95:99-106. [Crossref] [PubMed]
- Saradna A, Do DC, Kumar S, et al. Macrophage polarization and allergic asthma. Transl Res 2018;191:1-14. [Crossref] [PubMed]
- Arora S, Dev K, Agarwal B, et al. Macrophages: Their role, activation and polarization in pulmonary diseases. Immunobiology 2018;223:383-96. [Crossref] [PubMed]
- Kyriakis JM, Avruch J. Mammalian MAPK signal transduction pathways activated by stress and inflammation: a 10-year update. Physiol Rev 2012;92:689-737. [Crossref] [PubMed]
- Chen Y, Lu N, Ling Y, et al. Wogonoside inhibits lipopolysaccharide-induced angiogenesis in vitro and in vivo via toll-like receptor 4 signal transduction. Toxicology 2009;259:10-7. [Crossref] [PubMed]
- Liu Y, Shepherd EG, Nelin LD. MAPK phosphatases--regulating the immune response. Nat Rev Immunol 2007;7:202-12. [Crossref] [PubMed]
- Lai L, Song Y, Liu Y, et al. MicroRNA-92a negatively regulates Toll-like receptor (TLR)-triggered inflammatory response in macrophages by targeting MKK4 kinase. J Biol Chem 2013;288:7956-67. [Crossref] [PubMed]
- Lao Y, Yang K, Wang Z, et al. DeSUMOylation of MKK7 kinase by the SUMO2/3 protease SENP3 potentiates lipopolysaccharide-induced inflammatory signaling in macrophages. J Biol Chem 2018;293:3965-80. [Crossref] [PubMed]
- Shen H, Wu N, Wang Y, et al. JNK Inhibitor SP600125 Attenuates Paraquat-Induced Acute Lung Injury: an In Vivo and In Vitro Study. Inflammation 2017;40:1319-30. [Crossref] [PubMed]
- Li JK, Nie L, Zhao YP, et al. IL-17 mediates inflammatory reactions via p38/c-Fos and JNK/c-Jun activation in an AP-1-dependent manner in human nucleus pulposus cells. J Transl Med 2016;14:77. [Crossref] [PubMed]
- Ip YT, Davis RJ. Signal transduction by the c-Jun N-terminal kinase (JNK)--from inflammation to development. Curr Opin Cell Biol 1998;10:205-19. [Crossref] [PubMed]
- Lukey MJ, Greene KS, Erickson JW, et al. The oncogenic transcription factor c-Jun regulates glutaminase expression and sensitizes cells to glutaminase-targeted therapy. Nat Commun 2016;7:11321. [Crossref] [PubMed]
- Hsieh H-L, Lin C-C, Chan H-J, et al. c-Src-dependent EGF receptor transactivation contributes to ET-1-induced COX-2 expression in brain microvascular endothelial cells. Journal of neuroinflammation 2012;9:152. [Crossref] [PubMed]
- Jang S, Kelley KW, Johnson RW. Luteolin reduces IL-6 production in microglia by inhibiting JNK phosphorylation and activation of AP-1. Proc Natl Acad Sci U S A 2008;105:7534-9. [Crossref] [PubMed]