Ruxolitinib protects lipopolysaccharide (LPS)-induced sepsis through inhibition of nitric oxide production in mice
Introduction
Sepsis is the most vital complication of trauma, burn, and critical surgical patients at present. If not controlled in a timely and effective manner, it can quickly lead to multiple organ damage, or even multiple organ dysfunction syndromes (MODS) (1). The occurrence of sepsis is related to wound, bacteria, or bacterial toxins such as lipopolysaccharide (LPS). However, the latest research shows that immune disorder is the primary reason for septic organ damage, and the mechanism might involve the overreaction of the body to inflammation factors (2). In other words, once the responsibilities inherent in the body and related to defense and repair mechanism is out of control, it is sometimes more harmful to the body than the wound or bacterial invasion itself. Thus, the early inhibition of inflammatory overreaction is an essential means to prevent and treat sepsis.
Macrophages have a vital role in inflammation. Toll-like receptor (TLR)-4and active macrophages (3) can recognize LPS, which is a glycolipid found in the outer membrane of Gram-negative bacteria. In macrophages, Toll-like receptor 4 (TLR4), CD14, and MD2 combined with being a cognate receptor complex when stimulated by LPS. This is followed by the TLR4 receptor complex signal transduction pathway being activated after a series of cytokines are released (3). In response to the stimulation by LPS, activated macrophages produce inflammatory cytokines such as TNF-α, IL-1β, and IL-6, with nitric oxide (NO) acting as a mediator (4).
Ruxolitinib, an inhibitor of Janus kinases (JAK) 1/2, is the only orally administered drug approved for the treatment of myelofibrosis patients (5). In these patients, including those negative for theJAK2V617F mutation, ruxolitinib exhibits notable effects in alleviating clinical symptoms (6). In a JAK2V617F-positivemyeloproliferative neoplasms (MPN) mouse model, splenomegaly was relieved by oral administration of ruxolitinib, and inflammatory cytokines were reduced in JAK2V617F mutant cells in the spleen (5). However, the exact mechanism of this ruxolitinib effect is still not completely understood. The present study was aimed at clarifying this issue and found that ruxolitinib performs yet another pharmacological activity, which could improve the survival of septic mice: the inhibition of LPS-mediated NO production.
We present the following article in accordance with the ARRIVE guideline checklist (available at http://dx.doi.org/10.21037/atm-20-2972).
Methods
Chemicals and reagents
Ruxolitinib was purchased from Aladdin (INCB018424), while dimethyl sulfoxide (DMSO) and LPS (Escherichia coli 055:B5)was obtained from Sigma Chemical Co. (St. Louis, MO, USA). Dulbecco’s modified Eagle’s medium (DMEM), fetal bovine serum (FBS), penicillin, and streptomycin were purchased from Invitrogen-Gibco (Grand Island, NY, USA). Polyclonal antibodies against inducible nitric oxide synthase (iNOS) were obtained from Abcam (ab3523) and Glyceraldehyde 3-phosphate dehydrogenase (GAPDH) was obtained from Santa Cruz Biotechnology (SantaCruz, CA, USA). Horseradish peroxidase (HRP)-conjugated anti-rabbit, and goat-mouse secondary antibodies were purchased from GE Healthcare (Buckinghamshire, UK). Polyvinylidene difluoride (PVDF) membrane was purchased from What man GmbH (Germany). Pierce ECL Western Blotting substrate was obtained from Thermo Fisher Scientific Inc. (Pierce Biotechnology Inc., Rockford, IL, USA). Mouse Cytokine Array Panel A was purchased from R&D (Cat: ARY006). All other chemicals were of reagent grade.
Cell culture and experimental animals
The murine macrophage cell line RAW 264.7 was purchased from the American Type Culture Collection (ATCC) and was cultured in DMEM supplemented with 10% heat-inactivated fetal bovine serum, 100 U/mL of penicillin, and 100 µg/mL of streptomycin. The cells were incubated in a humidified atmosphere of 5% CO2 at 37 °C and were subcultured every two days. Jihui Experimental Animal Company Shanghai, provided the male C57Bl/6 mice (20–25 g). They were maintained under controlled conditions (23±3 °C, 50%±10% humidity, and 12 h day/night rhythm) and fed standard laboratory chow. All animal experiments were approved by the Institutional Animal Care and Use Committee of the Second Military Medical University following the Guide for Care and Use of Laboratory Animals published by the U.S. National Institutes of Health (NIH) (publication No. 96-01).
Determination of NO production
NO production was determined by the amount of nitrite accumulated in the culture medium. RAW 264.7 cells were plated in 96-well plates at the destiny of 5×103 cells/well. After incubation for 24 hours, the cells were treated with 0.1 µg/mL LPS in the presence of vary in concentrations of ruxolitinib for 24 hours. An aliquot of 50 µL culture supernatant was mixed with 50 µL Griess reagent (1% sulfanilamide and 0.1% naphthyl ethylenediamine dihydrochloride in 2.5% phosphoric acid) (7), followed by incubation at room temperature for 15 min. The absorbance at 570 nm was measured. The concentrations of nitrite were calculated based on a sodium nitrite standard curve.
Western blot
RAW 264.7 cells were plated in 6-well plates at the destiny of 5×105 cells/well. After 24 hours of incubation, the cells were pretreated for 30 min with different ruxolitinib concentrations before being stimulated for 24 h with 0.1 µg/mL of LPS. The supernatants were removed, and the cold phosphate-buffered saline (PBS) was used to wash the cells twice. Then, the cells were collected and lysed in lysis buffer (50 mM Tris•HCl, pH 7.4, 150 mM NaCl, 1% Triton X-100, 1% sodium deoxycholate, 0.1% SDS, 20 mM β-glycerophosphate, 1 mm phenylmethylsulfonylfluoride, 2 mM p-nitrophenyl phosphate, and 1:50 protease inhibitor) on ice for 30 min. Ten percent SDS-PAGE resolved the lysates and proteins were moved to PVDF membranes. After being blocked with 5% bovine serum albumin (BSA) in Tris-buffered saline with Tween20 (TBST) buffer for 1 h at room temperature, the membranes were incubated with specific primary antibodies overnight at 4 °C. The membranes were washed with TBST three times, and then incubated at room temperature for 1 h with secondary antibodies. Chemiluminescence substrates detected the membranes.
Quantitative real-time polymerase chain reaction (qRT-PCR) (SYBR Green method)
Total RNA was extracted from RAW264.7 cells using Rizal. qRT-PCR was conducted using SYBR Green PCR Master Mix in a total volume of 10 µL on Step OnePlus Real-Time PCR System (Applied Biosystems) as follows: 95 µC for 30 s, 40 cycles of 95 µC for 15 s, 60 µC for 30 s, and 72 µC for 35 s. GAPDH was used for the reference genes. The relative levels of gene expression were represented as ΔCt = Ctgene − Ct reference; the fold change of gene expression was calculated using the 2−ΔΔCT method. The primer sequences were as follows:
TNF-α (Mouse): F, 5'-TGTCTCAGCCTCTTCTCATT-3',
R, 5'-AGATGATCTGAGTGTGAGGG-3'
IL-6 (Mouse): F, 5'-ATGAAGTTCCTCTCTGCAAGAGACT-3',
R, 5'-CACTAGGTTTGCCGAGTAGATCTC-3'
iNOS (Mouse): F, 5'-CTCTACAACATCCTGGAGCAAGTG-3',
R, 5'-ACTATGGAGCACAGCCACATTGA-3'
GAPDH (Mouse); F, 5'-AGAACATCATCCCTGCATCC-3',
R, 5'- TCCACCACCCTGTTGCTGTA-3'
Endotoxemia model
C57Bl/6 mice were randomized (10 per group) into a control group and treatment group. The treatment group was injected intraperitoneally with ruxolitinib (0.67 mg/Kg, diluted with 10% DMSO) 30 mins in advance while the control group was injected with isotonic saline. The two groups were then injected intraperitoneally with LPS (20 mg/kg) (Sigma-Aldrich Corp., St. Louis, MO, USA); their time of death was recorded, and their survival rate was calculated by log-rank statistical method.
Tissue and serum harvested in mice
The liver and kidney were collected 24 h after the endotoxemia model was established, and serums (200 µL) in different groups were collected in different hours. For hematoxylin and eosin (H&E) staining in paraffin, the tissue was fixed in 10% formalin for 24 hand embedded in paraffin. Five-micron-thick sections were stained with H&E and photographed under a fluorescence microscope (Leica, German).
Mouse cytokine array panel
Add 100 µL serum to each array unit, and each membrane needs to be sealed with 2 mL of corresponding array buffer and incubated on the shaking table for 1 hour. The sample needs to be diluted to 1.5 mL volume per hole with the corresponding array buffer, and incubated at room temperature for 1 h with the diluted test antibody. After that, the corresponding array unit was added and incubated with the array protein membrane at 4 °C overnight. Wash the membrane with wash buffer for three times, add streptavidin-HRP, incubate for 30 min, and shake it at room temperature. Wash the film with wash buffer for three times, add 1 mL of chemical reagent into each hole, and develop the color on the chemiluminescence imager. Data was analysed with professional software.
Statistical analysis
Data were analyzed by one-way analysis of variance (ANOVA) and Student’s t-test using SPSS v.20.0 software (SPSS Inc., Chicago, IL, USA). Differences between the groups were calculated using the least significant differences method, and the significance level was defined as P<0.05. Results are expressed as mean ± SD.
Results
Ruxolitinib acts as an inhibitor of LPS-induced NO production in RAW cells
Ruxolitinib was an inhibitor of JAK1/2; its molecular structure is shown in Figure 1A. To verify the activity of ruxolitinib on RAW cells, we determined the dose-response curve using increasing concentrations of ruxolitinib. Different concentrations of ruxolitinib (0.3, 0.6, 1.3, 2.5, and 5 µM) dramatically inhibited NO production after LPS stimulation (*, P<0.05, Figure 1B).
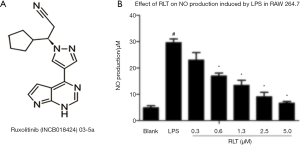
Effects of ruxolitinib on pro-cytokine production
To determine whether the inhibitory activity of ruxolitinib on NO was due to a decrease in the expression level of its biosynthetic enzyme iNOS, we used Western blot analysis to detect iNOS proteins from cell lysates. Thus, RAW264.7 cells were pretreated with ruxolitinib 30 min before they were stimulated with LPS (10 µgs/mL). As shown in Figure 2A, iNOS expression was inhibited by ruxolitinib in a dose-dependent fashion, paralleling its effect on NO production. Furthermore, cells treated with ruxolitinib (2.5 µM) exhibited a lower mRNA expression of iNOS (6.186±0.999), TNF-α (3.641±0.481), and IL-6 (4.235±0.485) compared with those in the LPS group (*, P<0.05, Figure 2B). These results suggested that the effect of Ruxolitinib on NO production was caused by its suppression of iNOS production and inhibition of pro-cytokines on the mRNA level.
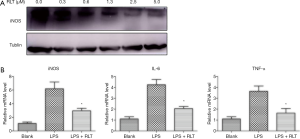
Ruxolitinib protected against LPS-induced mortality and organ histopathological damage in C57 mice
In order to evaluate the effects of ruxolitinib treatment on sepsis-induced by LPS, we established an endotoxemia model with C57 mice and recorded different times of death. The survival with ruxolitinib treatment mouse was 70% greater than that of the LPS control group (***, P<0.001, N=10, Figure 3A). It was found in the kidney and liver at 24 hours after LPS-induced injury, irregular cell morphology, serious hemorrhage and many inflammatory cells. Nonetheless, ruxolitinib treatment (Figure 3B) substantially alleviated the symptoms of this histopathological damage, indicating that ruxolitinib improved the survival of LPS-induced sepsis and relieved organ injury. According to the Cytokine Array Pane results (Figure 4A), 40 inflammatory cytokines were tested at the same time. Pro-inflammatory cytokines like TNF-α, IL-6, IL-1β, GM-SCF, IL-23 were declined in different hours after ruxolitinib treatment compared with LPS control group (Figure 4B,C,D,E,F), whereas anti-inflammatory of IL-10 increased in 18 h after ruxolitinib performed as shown (Figure 4G).
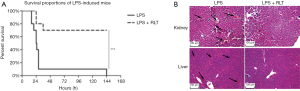
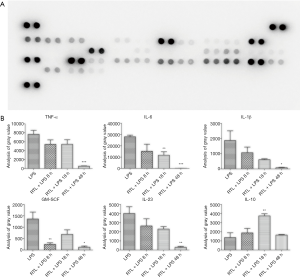
Discussion
Ruxolitinib is an inhibitor of JAK1/2 and reduces cell proliferation, increases apoptosis, and inhibits STAT3 (a substrate of JAK1 and JAK2) and STAT5 phosphorylation in cells harboring the JAK2V617F mutation. In a JAK2V617F positive MPN mouse model, splenomegaly was found to be relieved, and survival was increased by oral administration of ruxolitinib. Also, inflammatory cytokines were reduced in JAK2V617F mutant cells in the spleen. These effects are the same as those observed in clinical trials (8). Phosphorylation of STAT3 was also found to be reduced with ruxolitinib administrated both in the blood of healthy volunteers and patients with myelofibrosis (9). In this study, we first showed that ruxolitinib demonstrated inhibitory effects of LPS-induced NO production and inflammation, and then we illustrated its potential in the treatment for LPS-induced sepsis.
Macrophages have a crucial role in the inflammatory response, wound tissue repair, and secretion of inflammatory cytokines (10). NO is one of the essential inflammatory mediators who are created by LPS-stimulated macrophages and can cause tissue damage by oxidative stress and DNA damage (11). Our study showed that ruxolitinib dramatically decreased LPS-induced NO production and expression of iNOS in LPS-activated RAW 264.7 cells. Furthermore, an excessive pro-inflammatory storm can cause tissue damage, organ failure, and even death (12). Therefore, inhibiting the production of excessive pro-inflammatory cytokines is a useful measure in controlling infectious diseases (13). Our results indicate that ruxolitinib inhibited TNF-α and IL-6 in LPS-activated RAW 264.7 cells, suggesting that ruxolitinib may have potential in treating a wider variety of inflammation-related diseases beyond its currently approved use in myelofibrosis.
LPS is critical to the pathogenesis of sepsis, and it induces tissue damage and organ failure by activating immune response (14,15). Reducing the damage of excessive inflammatory cytokines can effectively prevent and treat sepsis (16). In the present study, we evaluated the anti-inflammatory activities of ruxolitinib using LPS-induced mice. Our results showed that the administration of ruxolitinib inhibited the infiltration of inflammatory cells in the liver and kidney and decreased hemorrhage and mitigated the loss of hepatic cells and renal cells in LPS-induced mice. These data indicate that ruxolitinib is capable of preventing LPS-induced acute organ injury by abating LPS-induced tissue damage through the inhibition of NO and TNF-α production.
The mortality rate of sepsis is generally about 30%, while severe sepsis and sepsis shock frequently lead to mortalities of 50% and 80%, respectively (17). The reduction of mortality sepsis-related mortality has always been a much-investigated issue in critical care medicine. The LPS challenge (20 mg/kg) resulted in a mortality rate of nearly 90% within 24 h in our experimental septic animal model. Ruxolitinib administration not only extended the survival span, but also boosted the survival rate to 70%. The decline of inflammatory cytokines in the serum demonstrated the specific anti-inflammatory action. As known, NF-κB has been shown to play a critical role in LPS-induced inflammatory cytokine and NO production (18). The specific mechanism of anti-inflammatory effects of ruxolitinib maybe associated with NF-κB signaling pathways, but this needs to be clarified with further study.
In summary, ruxolitinib has an anti-inflammatory role in inflammation caused by LPS, and has protective effects in septic shock induced by LPS. We used murine macrophages (RAW 264.7 cells) and a septic animal model to study the anti-inflammatory effects of ruxolitinib. Our results showed that ruxolitinib inhibited LPS-induced NO expression, decreased LPS-induced TNF-α, IL-6, and iNOS development, protected LPS-induced tissue damage, and increased the LPS-induced septic shock survival rates. Such findings offer a new hypothesis about the mechanism behind ruxolitinib’s anti-inflammatory activity, and can be a promising path for the production of sepsis-treatment drugs.
Acknowledgments
Funding: National Nature Science Foundation of China (81930057, 8772076, 81801914), the Innovative Cultivation Fund of Navy General Hospital (CXPY201820), and the Military Medical Program of General Hospital, PLA (QNC19025) funded this work.
Footnote
Guideline Checklist: The authors have completed the ARRIVE guideline checklist (available at http://dx.doi.org/10.21037/atm-20-2972).
Data Sharing Statement: Available at http://dx.doi.org/10.21037/atm-20-2972.
Conflicts of Interest: All authors have completed the ICMJE uniform disclosure form (available at http://dx.doi.org/10.21037/atm-20-2972). The authors have no conflicts of interest to declare.
Ethical Statement: The authors are accountable for all aspects of the work in ensuring that questions related to the accuracy or integrity of any part of the work are appropriately investigated and resolved. All animal experiments were approved by the Institutional Animal Care and Use Committee of the Second Military Medical University following the Guide for Care and Use of Laboratory Animals published by the U.S. National Institutes of Health (NIH) (publication No. 96-01).
Open Access Statement: This is an Open Access article distributed in accordance with the Creative Commons Attribution-NonCommercial-NoDerivs 4.0 International License (CC BY-NC-ND 4.0), which permits the non-commercial replication and distribution of the article with the strict proviso that no changes or edits are made and the original work is properly cited (including links to both the formal publication through the relevant DOI and the license). See: https://creativecommons.org/licenses/by-nc-nd/4.0/.
References
- Serhan CN, Savill J. Resolution of inflammation: the beginning programs the end. Nat Immunol 2005;6:1191-7. [Crossref] [PubMed]
- Shahreyar M, Fahhoum R, Akinseye O, et al. Severe sepsis and cardiac arrhythmias. Ann Transl Med 2018;6:6. [Crossref] [PubMed]
- Bode JG, Ehlting C, Haussinger D. The macrophage response towards LPS and its control through the p38(MAPK)-STAT3 axis. Cell Signal 2012;24:1185-94. [Crossref] [PubMed]
- Iwasaki A, Medzhitov R. Toll-like receptor control of the adaptive immune responses. Nat Immunol 2004;5:987-95. [Crossref] [PubMed]
- Plosker GL. Ruxolitinib: a review of its use in patients with myelofibrosis. Drugs 2015;75:297-308. [Crossref] [PubMed]
- Singh K, Sazawal S, Chhikara S, et al. Association of JAK2V617F mutation with thrombosis in Indian patients with Philadelphia negative chronic myeloproliferative neoplasms. Indian J Pathol Microbiol 2018;61:371-4. [Crossref] [PubMed]
- Liu RH, Hotchkiss JH. Potential genotoxicity of chronically elevated nitric oxide: a review. Mutat Res 1995;339:73-89. [Crossref] [PubMed]
- Quintas-Cardama A, Vaddi K, Liu P, et al. Preclinical characterization of the selective JAK1/2 inhibitor INCB018424: therapeutic implications for the treatment of myeloproliferative neoplasms. Blood 2010;115:3109-17. [Crossref] [PubMed]
- Verstovsek S, Kantarjian H, Mesa RA, et al. Safety and efficacy of INCB018424, a JAK1 and JAK2 inhibitor, in myelofibrosis. N Engl J Med 2010;363:1117-27. [Crossref] [PubMed]
- Medzhitov R. Origin and physiological roles of inflammation. Nature 2008;454:428-35. [Crossref] [PubMed]
- Guzik TJ, Korbut R, Adamek-Guzik T. Nitric oxide and superoxide in inflammation and immune regulation. J Physiol Pharmacol 2003;54:469-87. [PubMed]
- Cinel I, Opal SM. Molecular biology of inflammation and sepsis: a primer. Crit Care Med 2009;37:291-304. [Crossref] [PubMed]
- Thamphiwatana S, Angsantikul P, Escajadillo T, et al. Macrophage-like nanoparticles concurrently absorbing endotoxins and proinflammatory cytokines for sepsis management. Proc Natl Acad Sci U S A 2017;114:11488-93. [Crossref] [PubMed]
- Cohen J. The immunopathogenesis of sepsis. Nature 2002;420:885-91. [Crossref] [PubMed]
- Hung YL, Fang SH, Wang SC, et al. Corylin protects LPS-induced sepsis and attenuates LPS-induced inflammatory response. Sci Rep 2017;7:46299. [Crossref] [PubMed]
- Toner P, McAuley DF, Shyamsundar M. Aspirin as a potential treatment in sepsis or acute respiratory distress syndrome. Crit Care 2015;19:374. [Crossref] [PubMed]
- Jawad I, Luksic I, Rafnsson SB. Assessing available information on the burden of sepsis: global estimates of incidence, prevalence and mortality. J Glob Health 2012;2:010404. [Crossref] [PubMed]
- Peng H, Guerau-de-Arellano M, Mehta VB, et al. Dimethyl fumarate inhibits dendritic cell maturation via nuclear factor kappaB (NF-kappaB) and extracellular signal-regulated kinase 1 and 2 (ERK1/2) and mitogen stress-activated kinase 1 (MSK1) signaling. J Biol Chem 2012;287:28017-26. [Crossref] [PubMed]