Ultrasonographic hemodynamics for prediction of poor liver regeneration induced by severe portal vein stenosis in rats
Introduction
Because of the severe shortage of donor’s livers, living donor liver transplantation (LDLT) has undergone rapid development with the advancement in modern surgical techniques (1-3). Liver regeneration post-LDLT is a vital process of liver recovery, and the portal vein blood flow, accounting for 70–80% of the hepatic blood supply, provides a fundamental basis and a prerequisite for successful liver regeneration and survival. Insufficient portal vein blood flow, such as portal vein stenosis (PVS), is a significant complication after LDLT, especially in children. Mild PVS is conventional but does not affect liver function and regeneration. In patients with severe PVS, poor regeneration may occur, complicated with portal hypertension, small-for-size syndrome, or even liver failure. Early diagnosis and prompt treatment are critical (4-8).
As a non-invasive, cost-effective, and non-radioactive technique with bedside availability, ultrasound (US) serves as a first-line imaging modality to diagnose PVS in the early postoperative period and long-term follow-up. In general, PVS is suggested if the portal vein is regional stenosis, with blood flow aliasing and acceleration at the stenotic site. Most of the researchers believe that evident PVS can be diagnosed if the stenotic diameter is <2.5–3.5 mm or the diameter stenosis ratio (DSR)>50%, blood flow velocity (PVV) at the stenotic site (PVVs) >150 cm/s or the accelerating velocity ratio (AVR) ≥3 Jeny(9-11). However, to date, unlike the criteria for grading carotid artery stenosis (12), there is still no consensus on the ultrasound diagnostic criteria for evaluating the degree of PVS. Moreover, which degree of PVS could induce poor liver regeneration still is unclear. In the clinic, the treatments are usually provided when evident PVS was detected, accompanied by apparent symptoms such as abnormal transaminase level, blood coagulation disorders, portal hypertension, and so on. But in that case, irreversible damages of hepatocytes may already occur, and consequently, the grafts would be in the state of poor regeneration for a long-time even treatments are applied. Liver regeneration can be assessed by pathological and immunohistochemical tests such as the expression of proliferating cell nuclear antigen (PCNA), a kind of nucleoprotein only synthesized and expressed in proliferative cells, but these examinations are invasive and more costly (13,14). Therefore, there is a growing demand for the correct assessment of the degree of PVS, early predicting the possible poor liver regeneration induced by severe PVS noninvasively.
Due to the diverse causes of hepatic diseases and the limitation in ethics, most research studies liver regeneration based on experimental animals. A rat model of 70% partial hepatectomy (PH) is a widely accepted liver regeneration research model, which is famous for its regeneration induction without causing fulminant hepatic failure (15). Partial ligation of the portal vein is commonly used for producing the PVS model (16). Therefore, this study used a combination of 70% PH and portal vein partial ligation to set up (PH + PVS) rat models. Based on the assessment of liver regeneration in the different degrees of PVS, the degree of PVS inducing poor liver regeneration was determined. Moreover, a comparative analysis was performed to evaluate the value of Ultrasound parameters PVVs, DSR, and AVR for predicting poor liver regeneration induced by severe PVS. These findings will supply experimental evidence for further investigating PVS diagnosis and grading in the clinic.
Methods
Study subjects
One hundred and two healthy male Sprague-Dawley rats (200–400 g, 7–14 weeks of age, SPF grade) were purchased from Chengdu Dashuo Biotechnology Co., Ltd., and acclimatized for at least 7 days to laboratory conditions with a constant temperature and a 12 h light-dark cycle at the animal experiment center of West China Hospital.
The rats were randomly divided into five groups as follows: sham operation rats (SOR group, n=6), PH group (group A, n=24), and PVS groups with mild, moderate, and severe PVS after PH (group B, C, and D, n=24 respectively). Also, rats in A–D groups were further divided into four subgroups, based on the time after surgery (Jeny1, 3, 7, and 14 d), with 6 rats in each subgroup. The animal ethics committee approved all the rats and procedures of West China Hospital, Sichuan University. Rats were kept warm and had free access to food and water throughout the experiment.
Construction of rat models
Construction of 70% PH rat model
Rats were placed in the supine position after ether anesthesia, and then a 70% hepatectomy model was constructed based on methods described by Higgins et al. (15). Briefly, the middle lobe and left lateral lobe (about 68% of the total volume of the liver) were excised and weighed.
Construction of PVS model after PH
After PH, the portal vein was separated. A microvascular caliper was used to measure the portal vein diameter (PVD) at the site of pre-ligation, which was below the porta hepatis, and about 3 mm above the joint of the splenic vein and superior mesenteric vein. Based on pre-defined stenosis degrees, the diameter of the stenotic segment was calculated from the formula SR = (1 − Dstenosis/PVD) × 100%, and then the corresponding gauge of a medical needle was selected. The separated portal vein and the needle were ligated together by 3-0 silk suture, and afterward, the needle was removed slowly. The diameter of the stenotic segment of the portal vein was equaled to the outer diameter of the needle. Different degrees of PVS was induced using this method. In the end, 32,000 units penicillin and 5 mL NaCl (0.9%) was injected intraperitoneally in each rat before closing the abdominal cavity.
For the SOR group, neither hepatectomy nor portal vein ligation was performed during surgery. For the PH group (group A), only 70% hepatectomy was conducted. For the PVS groups (groups B–D), different degrees of PVS were conducted following PH.
Ultrasound examination
Ultrasound was used to check the portal vein hemodynamic changes by a Philips iu22 ultrasound machine with a 5.0–12.0 MHz linear transducer. The rats were ether-anesthetized and placed in supine positions. The abdomen was shaved with an electric hair remover to minimize ultrasound attenuation. Ultrasound examination was performed by the same experienced physician, with rats supporting stable anesthesia.
In the SOR group and PH group (group A), PVD and PVV were measured by grayscale and Doppler ultrasound at preoperative and postoperative 1, 3, 7, and 14 d. In PVS groups (groups B–D), the PVD and PVV at the stenotic (PVDs, PVVs) and pre-stenotic (PVDpre, PVVpre) sites were measured, and then the DSR and AVR were subsequently calculated as follows: DSR = (PVDpre − PVDs)/PVDpre × 100%, and AVR = (PVVs − PVVpre)/PVVpre. When measuring the velocities, based on the vessel diameter and direction, the Doppler sample volume should be adjusted to 0.5 mm, and the angle of insolation should remain constant at less than 60°. PVVs should be detected at the stenotic site where the blood flow disturbance could be seen. All observation data were measured three times and averaged as the resultant values.
Evaluation of liver regeneration
Assessment of the expression of PCNA
The rats in A-D groups were sacrificed at 1, 3, 7, and 14 d post-surgery, and the liver tissue specimens (2.0×1.0×1.0 cm3) were obtained. Then, the liver tissues were fixed in 10% neutral formalin, embedded in paraffin, made into slices at 5 µm. Immunohistochemical staining was performed to assess PCNA expression under high magnification based on the paraffin sections, and hepatocytes with a brown-yellow nucleus were considered positive for staining. Besides, about 100 mg of liver tissue was also harvested when rats were sacrificed. The adipose tissue and connective tissue on the surface were removed as much as possible, and then the liver tissue was placed in cryogenic vials and stored in liquid nitrogen. Western blotting was performed for semi-quantification of PCNA expression in liver tissues. For the SOR group, the rats were sacrificed at 14 d post-surgery, with its liver tissues obtained for PCNA examination.
Assessment of liver regeneration rate (LRR)
The residual liver was excised and weighed when rats sacrificed at 14 d post-surgery. LRR was calculated based on the equation: LRR = D/E×100%, in which D represents the liver weight per 100 g of the body weight when sacrificed, and E represents the preoperative liver weight per 100 g of the body weight, which was calculated by (the excised liver weight/0.68) (17).
Statistical analysis
The analysis of variance (ANOVA) test was employed to study the differences in LRR, PCNA expression, and ultrasound parameters PVD, PVV, DSR, and AVR among groups. The least significant difference (LSD) test was used to compare the results pairs of groups with even variance, and the Dunnett-t3 test was used to test the data with uneven variance. Differences were considered significant if the P value <0.05. The receiver operating characteristic (ROC) curve was drawn, and the area under the curve (AUC), Std. Error, asymptotic Sig. (b), asymptotic 95% confidence interval, best cut-off, sensitivity, and specificity were calculated to evaluate the value of PVVs, DSR, and AVR in diagnosing severe PVS, predicting the resulting poor liver regeneration. All statistical analyses were performed utilizing SPSS 25.0 and GraphPad Prism 8.
Results
Model construction
In this study, 72 rat models of different degrees of PVS were successfully set up following 70% PH. The SRs of each PVS group were 45.16%±3.44%, 59.21%±3.83%, and 69.56%±2.16%, respectively. In each PVS group, no statistical difference of SRs was observed at postoperative 1, 3, 7, 14 d (all P>0.05, Figure 1).
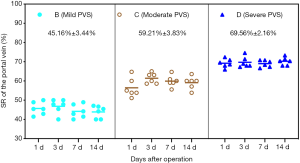
LRR
The residual liver weight of the rats increased with time after PH. The LRR of 70% PH group and mild PVS group with SR ≤50% on the 14th day both reached above 90%, which means the regenerated liver weight per 100 g bodyweight almost recovered to its original weight before surgery. Poor liver regeneration could be significantly induced when PVS was greater than 65% (group D), with the LRR of less than 80%. That was significantly lower compared to 70% PH group (group A) and PVS groups with SR ≤50% (group B) and SR >50–65% (group C), respectively (all P<0.05, Figure 2).
The expression of PCNA
Immunohistochemical staining of PCNA demonstrated that only a few positive cells, with brown-stained nuclei, were seen in the SOR group. In the 70% PH group, the positive expression of cells increased at 1 d post-surgery, presenting in the portal areas. At 3 d, the levels of PCNA-positive expression were still relatively high, with more expression in the central areas. At 7 d, the number of PCNA-positive cells decreased (Figure 3), while at 14 d, it was comparable to the levels in normal liver tissues. In PVS groups, the expression of PCNA showed similar trends with time. The negative reaction was observed in karyokinesis.
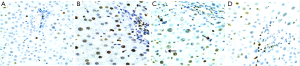
According to western blot analysis, PCNA expression appeared to be extremely low in the SOR group. In groups A-D, the expression had a similar trend with time. In brief, PCNA expression increased at 1 d after surgery, peaked at 3 d, and then gradually decreased at 7 and 14 d. At 3 d, the expression of PCNA in groups B and C were the highest, followed by group A, and then group D. More precisely, significant differences were detected between groups A and B and between groups A and C (both P<0.05). The expression of group D was significantly lower compared to group C at 1 d and groups A, B, and C at 3 d (all P<0.05). At 7 and 14 d, there were no statistical differences among groups (all P>0.05, Figures 4 and 5).
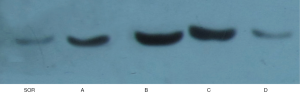
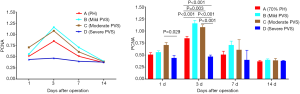
Ultrasound examination
PVD and PVV in 70% PH group
In the 70% PH group, the residual liver and blood flow in the portal vein could be proved clearly by ultrasound. PVD started to increase at 1 d, peaked at 3 d, decreased gradually at 7 d, and recovered to the normal levels at 14 d. PVD at 3 d was significantly higher than 14 d PVD (P<0.05). In contrast, PVV decreased to the lowest level at 3 d, increased gradually at 7 d, and then recovered similarly to normal levels at 14 d. PVV at 3 d was significantly lower than 1d and 14 d PVV (both P<0.05). The results are shown in Figure 6.
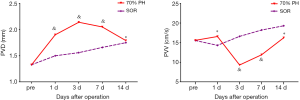
Ultrasound PVVs, DSR, and AVR in PVS groups
PVVs: gray-scale ultrasound could prove the portal vein stenosis clearly, and Doppler ultrasound showed blood flow disturbance at the stenotic site with accelerated flow velocity (Figure 7). After surgery, PVVs increased dramatically to the peak at 1 d, and then gradually decreased at 3 and 7 d. The PVV of 70% PH group was significantly lower compared to PVVs of groups B–D at 1, 3, and 7 d and PVVs of group D at 14 d (all P<0.05). Among PVS groups, PVVs of group D was significantly higher than that of groups B and C at 1 d, and PVVs of group C and D were significantly higher than that of group B at 3 d (all P<0.05).
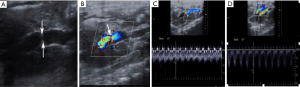
DSR: DSR in PVS groups were by the stenosis degree. Briefly, the DSR of group D was the highest, followed by group C, and then group B. At 1, 3, 7 d post-surgery, DSR of group C and D were significantly higher than that of group A (all P<0.05), while at 14 d, no significant difference was observed among PVS groups (all P>0.05).
AVR: at 1 d post-surgery, AVR reached the peak, and then gradually decreased to the lowest levels until 7–14 d. AVR of group D was significantly higher than that of groups B and C at 1 and 3 d after surgery (all P<0.05), while there were no statistical differences among PVS groups at 7 and 14 d (all P>0.05).
All the results of PVVs, DSR, and AVR in the distinct groups are showed in Table 1 and Figure 8.
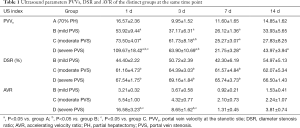
Full table
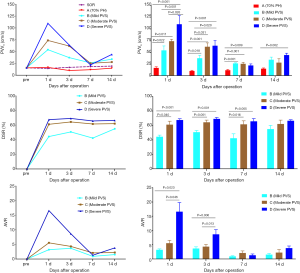
ROC analysis
In this study, poor liver regeneration could be significantly induced by severe PVS (SR >65–99%). According to the comparative analysis of portal vein hemodynamic changes in different degrees of PVS, ultrasound parameters PVVs, DSR, and AVR of severe PVS group were significantly different from mild or moderate PVS groups, especially at 1 and 3 d post-surgery. Therefore, the ROC analysis was applied to assess the value of PVVs, DSR, and AVR in differentiating severe PVS from mild-moderate PVS at 1 and 3 d, with mild-moderate PVS, defined as negative results and severe PVS defined as a positive diagnosis.
As is shown in Figure 9 and Table 2, at postoperative 1 d, the AUC of PVVs in diagnosing severe PVS was 0.84 (P<0.05 vs. AUC =0.50), and the best cut-off points were 81.20 cm/s, with a specificity up to 91.67% and a sensitivity of 66.67%. While at 3 d, it was unable to differentiate from severe or mild-moderate PVS by PVVs (P>0.05 vs. AUC =0.50). Comparatively, the AUC of DSR and AVR at 1 and 3 d in differentiating severe PVS from mild-moderate PVS were all greater than 0.80 (P<0.05 vs. AUC =0.50), much better in AVR (AUC >0.95). The best cut-off points of DSR at 1 and 3 d were 57.43% and 66.06%, with the sensitivity and specificity respectively 100%, 75% at 1 d, and 83.33%, 83.33% at 3 d. About AVR, the best cut-off points at 1 and 3 d were 6.91 and 5.36, with the sensitivity and specificity respectively 100%, 91.67% at 1 d, and 100%, 83.33% at 3 d.
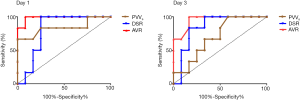
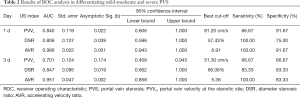
Full table
Discussion
The 70% partial hepatectomy (PH) model developed by Higgins et al. is the classical model used for liver regeneration research (15). It is easy to construct and have high survival rates. Partial ligation of the portal vein is commonly used to induce portal vein stenosis (PVS) model (16). In this study, portal vein partial ligation was performed using different gauges of medical needles to induce rat models with different degrees of PVS following 70% PH. This (PH + PVS) model is easy to construct, stable, and ease of hemodynamic monitoring (18).
Liver regeneration occurs shortly after PH in rats, including regeneration of hepatocytes and reconstruction of hepatic tissue structures. Hepatocytes are in the G0 phase under normal physiological conditions. After PH, an orderly proliferation of residual liver cells can be triggered by injury-related factors. Hepatocytes start to enter the cell cycle firstly, with DNA synthesis peaking at 1 d, and afterward, cell division peaking at 3 d. After 1–2 cell cycles, the liver weight can return to a normal level at 7–14 d. The reconstruction of hepatic tissue structures depends on the proliferation of hepatic non-parenchymal cells, such as Kupffer cells and sinusoidal endothelial cells. In these cells, DNA synthesis generally peaks after 48 h post-PH. Proliferating cell nuclear antigen (PCNA) is a kind of nucleoprotein only synthesized and expressed in proliferative cells, and to aid in the assessment of the cell proliferation status in liver regeneration. In our study, the expression of PNCA after PH increased to the peak at 3 d, and the weight of regenerated liver recovered to near normal level at 14 d, following the regularity of liver regeneration (13,14,19-21).
In this study, PVD after PH started to increase at 1 d, peaked at 3 d, decreased gradually at 7–14 d, while PVV decelerated to the lowest at 3 d and recovered gradually at 7–14 d. The hemodynamic changes may be associated with hepatocyte proliferation status and the changes of hepatic sinusoidal blood flow resistance in liver regeneration. After PH, the total blood volume to the whole liver was infused into the residual liver tissues. The reduction of sinusoidal capillary beds and the resulting blood flow resistance augmentation caused portal hypertension, with an increase in PVD and a decrease in PVV. At 3 d, blood flow resistance in the portal vein augmented further with peaks of hepatocytes mitosis and hepatic sinusoidal construction, resulting in PVD increasing to its highest and PVV decelerating to its lowest. At 7 and 14 d, with the completion of liver regeneration and restoration of liver volume, portal hypertension was alleviated, and so, the PVD and PVV gradually recovered to normal levels. Also, the changes in the blood flow volume of the portal vein during liver regeneration influenced PVD and PVV.
Enough blood flow in the portal vein is critical for liver regeneration. When severe PVS occurs, the blood supply to the liver can be reduced dramatically, leading to poor liver regeneration or even liver atrophy (4-8). However, which degree of PVS can induce poor liver regeneration still is unclear. In this study, poor liver regeneration was significantly induced when PVS was greater than 65%, with the LRR at postoperative 14 d respectively lower compared to 70% PH group and PVS groups with SR ≤50% and SR >50–65%, as well as the expression of PCNA at 3 d. The levels of liver regeneration were not significantly low when PVS >50–65%, which may because the reduction of portal vein blood flow had a negative correlation with liver regeneration in a particular range, with more active liver regeneration than non-PVS rats. Additionally, unlike the carotid artery, of which the mild, moderate and severe stenosis were respectively defined as <50% stenosis, 50–69% stenosis, and ≥70 stenosis to near occlusion (12), there is no explicit standard for grading PVS to date. In this study, it was found that the rats could hardly survive when PVS was greater than 75%. Based on the liver regeneration in different degrees of PVS, the severe PVS was defined as SR >65–99%, which could induce significantly poor liver regeneration. Logically, the mild and moderate PVS were defined as SR ≤50% and SR >50–65%, respectively.
Imaging techniques play a vital role in the diagnosis of PVS. Angiography is the gold standard, but it is invasive and complex. Computed tomography (CT) causes radiation effects, and magnetic resonance imaging (MRI) is more costly (22,23). Ultrasound has been considered the primary noninvasive imaging modality to detect PVS, and the availability at the bedside and the absence of radiation hazards make it an ideal first-line examination. PVS can be proved by Ultrasound, with regional stenosis of PVD and blood flow aliasing and acceleration at the stenotic site. The PVV at the stenotic site (PVVs), the DSR, and the accelerating velocity ratio (AVR) are the most common measure to find PVS. Stenoses greater than 50% are considered hemodynamically significant. The more severe the stenosis is, the higher the PVVs becomes (9-11). However, there is still no consensus on the ultrasound criteria for grading PVS, especially in diagnosing the severe PVS, which can induce significantly poor liver regeneration. In this study, the differences in PVVs, DSR, and AVR of mild, moderate, and severe PVS varied by time. At 1 d post-surgery, PVVs and AVR of severe PVS were significantly higher compared to mild and moderate PVS. At 3 d, PVVs of moderate and severe PVS were both higher than that of mild PVS, and AVR of severe PVS still supported at a relatively higher level compared to mild and moderate PVS. At 7 and 14 d, there were no statistical differences among PVS groups.Similarly, DSR of moderate and severe PVS was higher than that of mild PVS at 1, 3, and 7d, while at 14 d, no significant difference was observed among PVS groups. For these changes, besides the stenosis degree was a critical affected factor, the changes of blood flow resistance in different levels of liver regeneration may also influence the portal vein hemodynamics, as well as the blood flow volume. With the progress of liver regeneration, the differences between PVVs, DSR, and AVR among PVS groups gradually diminished. Therefore, Ultrasound grading of PVS might better be performed in the early postoperative period, especially within three days, as showed in our experimental study. Also, PVVs accelerated significantly higher than PVV of non-PVS rats,but the differences among PVS groups fluctuated dramatically with time.
Moreover, based on ROC analysis, it was unable to differentiate from mild-moderate or severe PVS by PVVs at 3 d. Therefore, the PVVs could be regarded as a useful index for diagnosing PVS but were not applicable for evaluating the stenosis degree. Comparatively, the DSR and AVR proved to be useful in diagnosing severe PVS (SR >65%) and predicting the resulting poor liver regeneration, especially AVR (AUC >0.95). At 1 d, AVR >6.91 was proposed as the diagnostic criterion for severe PVS, the sensitivity and specificity were respectively up to 100% and 91.67%. Similarly, at 3 d, AVR >5.36 was regarded as the diagnostic criterion for severe PVS, the sensitivity and specificity were respectively up to 100% and 83.33%.
Conclusions
Poor liver regeneration could be significantly induced when PVS was greater than 65%. Ultrasound can well show the changes in portal vein hemodynamics in different degrees of PVS in rats. The parameters PVVs could be regarded as a useful index for diagnosing PVS but were not applicable for evaluating the stenosis degree. Comparatively, the parameters DSR and AVR, especially AVR, proved to be useful for differentiating severe PVS (>65%) in the early postoperative period, predicting the resulting poor liver regeneration.
Acknowledgments
Funding: This study was supported by the National Natural Science Foundation of China (No. 81671702 and No. 81701702).
Footnote
Conflicts of Interest: All authors have completed the ICMJE uniform disclosure form (available at http://dx.doi.org/10.21037/atm.2020.04.21). The authors have no conflicts of interest to declare.
Ethical Statement: The authors are accountable for all aspects of the work in ensuring that questions related to the accuracy or integrity of any part of the work are appropriately investigated and resolved. The animal ethics committee approved all the rats and procedures of West China Hospital, Sichuan University (No. 2019054A).
Open Access Statement: This is an Open Access article distributed in accordance with the Creative Commons Attribution-NonCommercial-NoDerivs 4.0 International License (CC BY-NC-ND 4.0), which permits the non-commercial replication and distribution of the article with the strict proviso that no changes or edits are made and the original work is properly cited (including links to both the formal publication through the relevant DOI and the license). See: https://creativecommons.org/licenses/by-nc-nd/4.0/.
References
- Tulla KA, Jeon H. Living Donor Liver Transplantation: Technical Innovations. Gastroenterol Clin North Am 2018;47:253-65. [Crossref] [PubMed]
- Bozkurt B, Dayangac M, Tokat Y. Living Donor Liver Transplantation. Chirurgia (Bucur) 2017;112:217-28. [Crossref] [PubMed]
- Kim SH, Park J, Park SJ. Impact of ABO-incompatibility on hepatic artery thrombosis in living donor liver transplantation. Ann Transl Med 2019;7:625. [Crossref] [PubMed]
- Cheng YF, Huang TL, Chen TY, et al. Liver Graft Regeneration in Right Lobe Adult Living Donor Liver Transplantation. Am J Transplant 2009;9:1382-8. [Crossref] [PubMed]
- Perkins JD. Incidence of portal vein complications following liver transplantation. Liver Transpl 2008;14:1813-5. [PubMed]
- Pérez-Saborido B, Pacheco-Sánchez D, Barrera-Rebollo A, et al. Incidence, management, and results of vascular complications after liver transplantation. Transplant Proc 2011;43:749-50. [Crossref] [PubMed]
- Youssef D, Niazi A, Alkhouri N. Long Term Complications in Pediatric Liver Transplant Recipients: What Every Pediatrician Should Know. Curr Pediatr Rev 2016;12:209-21. [Crossref] [PubMed]
- Taniguchi M, Shimamura T, Todo S, et al. Small-for-size Syndrome in Living-Donor Liver Transplantation Using a Left Lobe Graft. Surg Today 2015;45:663-71. [Crossref] [PubMed]
- Mullan CP, Siewert B, Kane RA, et al. Can Doppler sonography discern between hemodynamically significant and insignificant portal vein stenosis after adult liver transplantation. AJR Am J Roentgenol 2010;195:1438-43. [Crossref] [PubMed]
- Hsu HW, Huang TL, Cheng YF, et al. Sonographic evaluation of post-transplantation portal vein stenosis in pediatric living-donor liver transplant recipients with left-liver grafts. Transplant Proc 2016;48:1162-5. [Crossref] [PubMed]
- Ma L, Lu Q, Luo Y. Vascular complications after adult living donor liver transplantation: Evaluation with ultrasonography. World J Gastroenterol 2016;22:1617-26. [Crossref] [PubMed]
- Grant EG, Benson CB, Moneta GL, et al. Carotid artery stenosis: gray-scale and Doppler US diagnosis--Society of Radiologists in Ultrasound Consensus Conference. Radiology 2003;229:340-6. [Crossref] [PubMed]
- Ren YS, Qian NS, Tang Y, et al. Beneficial effects of splenectomy on liver regeneration in a rat model of massive hepatectomy. Hepatobiliary Pancreat Dis Int 2012;11:60-5. [Crossref] [PubMed]
- Okay E, Simsek T, Subasi C, et al. Cross effects of resveratrol and mesenchymal stem cells on liver regeneration and homing in partially hepatectomized rats. Stem Cell Rev Rep 2015;11:322-31. [Crossref] [PubMed]
- Higgins GM, Anderson RM. Experimental Pathology of the liver. Restoration of the liver of the white rat following partial surgical removal. ARCH Pathol 1931;12:186-202.
- Van Thiel DH, Gavaler JS, Slone FL, et al. Is feminization in alcoholic men due in part to portal hypertension: a rat model. Gastroenterology 1980;78:81-91. [Crossref] [PubMed]
- Qiao Jian-Liang, Sun Juan, Li Jun, et al. Liver Dual Arterial Blood Supply Maintains Liver Regeneration: Analysis of Signaling Pathways in Rats. Mol Med Rep 2018;17:979-87. [PubMed]
- Yang L, Luo Y, Ma L, et al. Establishment of a novel rat model of different degrees of portal vein stenosis following 70% partial hepatectomy. Exp Anim 2016;65:165-73. [Crossref] [PubMed]
- Koniaris LG, McKillop IH, Schwartz SI, et al. Liver regeneration. J Am Coll Surg 2003;197:634-59. [Crossref] [PubMed]
- Nobuoka T, Mizuguchi T, Oshima H, et al. Portal blood flow regulates volume recovery of the rat liver after partial hepatectomy: molecular evaluation. Eur Surg Res 2006;38:522-32. [Crossref] [PubMed]
- Garnol T, Kucera O, Stankova P, et al. Does simple steatosis affect liver regeneration after partial hepatectomy in rats? Acta medica (Hradec Kralove) 2016;59:35-42. [Crossref] [PubMed]
- Singh AK, Nachiappan AC, Verma HA, et al. Postoperative imaging in liver transplantation: what radiologists should know. Radiographics 2010;30:339-51. [Crossref] [PubMed]
- Roberts JH, Mazzariol FS, Frank SJ, et al. Multimodality imaging of normal hepatic transplant vasculature and graft vascular complications. J Clin Imaging Sci 2011;1:50. [Crossref] [PubMed]