Remifentanil attenuates cardiac dysfunction, lipid peroxidation and immune disorder in rats with isoproterenol-induced myocardial injury via JNK/NF-KB p65 inhibition
Introduction
In China, ischemic heart disease is a significant cause of death, and it carries a significant disease burden (1). Myocardial ischemia (MI) is the top cause of myocardial infarction, and in patients with acute myocardial infarction, apoptosis has been demonstrated to contribute to the death of cardiomyocytes (2). These findings supplied evidence that oxidative stress and myocardial apoptosis are worsened in the MI model (3,4). In a variety of pathological conditions, oxidative stress has been considered as a key factor in the pathogenesis of cardiac injury and the progression of cardiac dysfunction. In addition to regulating collagen synthesis, it also intensifies the inflammatory response (5). So far, the isoproterenol-induced MI model has been widely used in rats (6,7).
Owing to its titratable pharmacokinetic properties, the use of the opioid remifentanil, which is an ultra-short acting nonspecific esterase-metabolized opioid receptor agonist, is widespread and can achieve high intraoperative dosing with no impacts on recovery time (8). Remifentanil is a highly selective µ-opioid receptor agonist with strong sedation and analgesia abilities (9). Therefore, its use can be degraded rapidly by nonspecific esterases in the plasma and tissues (10). It has been used as an adjuvant analgesic for general anesthesia (11). In human keratinocytes, remifentanil improves hydrogen peroxide-induced oxidative injury (12). Recently, the anti-inflammatory effect of remifentanil has been demonstrated in vitro and in vivo by several studies (13,14), and its protective effect against hepatic ischemia-reperfusion injury has also been proved (15). Also, remifentanil maintains zinc (Zn) homeostasis at reperfusion by inhibiting Metal-responsive transcription factor 1 (MTF1) and Zn transporter 1 (ZnT1) expression, leading to the attenuation of endoplasmic reticulum stress and cardiac injury (16). Remifentanil can effectively reduce myocardial cell injury caused by myocardial ischemia-reperfusion in rats, improve cardiac function, reduce the myocardial infarction area, decrease cleaved caspase-3 in myocardial cells, and increase Bcl-2/Bax (17). Importantly, remifentanil can reduce the apoptosis rate of myocardial cells as well as ischemia-reperfusion-induced oxidative stress and inflammation by inhibiting the Fas/Fas ligand (FasL) signal transduction pathway (18). Lipopolysaccharide (LPS), a significant constituent of the bacterial cell wall, is commonly used to induce immune responses (19). LPS exposure is thought to cause severe stress in cardiomyocytes, resulting in a loss of myocardial integrity due to a combination of oxygen deficiency, calcium overload, and the overproduction of free radicals (20,21). Remifentanil protects H9C2 cardiomyocytes against LPS-induced oxidative injury, as a result of downregulating PKCβ2 activation and inhibiting autophagy (22). Remifentanil reduced LPS-induced inflammatory response through the PARP-1/NF-κB signaling pathway (23). Analyzing remifentanil and understanding whether it plays a protective role in MI, is studied in this article.
JNK, also known as C-Jun N-terminal kinase or stress-activated protein kinase (SAPK), is part of the mitogen-activated protein kinase (MAPK) superfamily. JNK protein kinase is encoded by three genes, including JNK1, JNK2, and JNK3. JNKI and JNK2 are present in various tissues of the body, while JNK3 is found in tissues such as the myocardium, heart, and testes (24). Notably, NF-κB takes part in the initiation of inflammatory response (25). Alamandine can protect rats from MI-reperfusion injury by reducing the inflammatory response via the activation of JNK phosphorylation and the inhibition of the NF-κB signaling pathway (26). However, remifentanil has not been reported to have a therapeutic effect on injury caused by MI. To evaluate the effects of remifentanil on cardiac dysfunction, lipid peroxidation, and immune disorder in rats, we established an MI rat model by subcutaneous injection of isoproterenol. We present the following article following the ARRIVE guideline checklist (available at http://dx.doi.org/10.21037/atm-20-3134).
Methods
MI model and remifentanil-treatment
Each of the animal experiments in this study was conducted according to the principles of the NIH Guide for the Care and Use of Laboratory Animals and received approval from Sichuan Provincial People’s Hospital.
Forty rats specific-pathogen-free (SPF) Sprague Dawley (SD) rats were divided at random into five groups: the control group, the Isop group, the low-dose group (10 µg/kg remifentanil), the medium-dose group (20 µg/kg remifentanil), the high-dose group (40 µg/kg remifentanil). The control rats were administered a subcutaneous saline injection for 2 days and an intraperitoneal saline injection for 7 days. The Isop group rats were subcutaneously injected with Isop (85 mg/kg/day) for 2 days and intraperitoneally injected with saline for 7 days. The Isop and remifentanil group rats were subcutaneously given isoproterenol for 2 consecutive days and intraperitoneally injected with remifentanil (10, 20, 40 µg/kg) for 7 days.
We used LPS to induce aggravated myocardial injury in rats to study further the protective effect of remifentanil on the myocardial injury. Rats were divided at random into five groups: the control group, the Isop group, the high-dose group (40 µg/kg remifentanil), the Isop + lipopolysaccharide (LPS) group, and the Isop + LPS + remifentanil (40 µg/kg) group. The Isop + LPS rats were subcutaneously given isoproterenol for 2 consecutive days and intraperitoneally injected with LPS (15 mg/kg/day) for 5 days. The Isop + LPS + remifentanil rats were subcutaneously given Isop for 2 consecutive days and intraperitoneally injected with LPS (15 mg/kg/day) for 5 days, before receiving an intraperitoneal injection of remifentanil (40 µg/kg) for 7 days. The rats were housed in a controlled environment at 25±3 °C, humidity 60%, in a 12 h light/dark cycle with free access to water.
Remifentanil was bought from Hubei Yichang Renfu Co., Ltd. It was dissolved in saline (0.9% NaCl) to a volume of 1.6 mL. Rats were intraperitoneally injected with remifentanil (10, 20, 40 µg/kg) for 7 days.
On day 3, 24 h after the second injection of Isop, Creatine kinase-MB (CK-MB) and cTnI markers were measured by taking blood samples from the tail vein of the rats. On day 10, 24 h after the final injection of remifentanil, blood samples were taken via the cardiac puncture to measure the profile of antioxidants and lipids before the rats were sacrificed.
Echocardiography
Echocardiography was performed as previously described (27). Left ventricular wall thickness (LVWT), left ventricular end systolic volume (LVESV), fraction shortening (FS), and heart rate (HR) were measured. Left ventricular ejection fraction (LVEF%) = (LVEDV−LVESV)/LVEDV ×100%.
Histology
Sodium pentobarbital (40–60 mg/kg) was intraperitoneally injected to anesthetize the rats and the heart tissue was later removed. The myocardium of rats was fixed with 4% paraformaldehyde for 24 h, embedded in paraffin, and sectioned to a thickness of about 4 µm. Hematoxylin and eosin (H&E) were used to stain the samples, and the histopathological morphology was observed under a light microscope.
Determination of myocardial enzymes, oxides index and cytokines
Blood samples from the arteries of the rats were taken and placed in a 1 mL heparinized centrifuge tube. The supernatant was collected by centrifugation at 4 °C, 3,500 rpm for 20 min and stored at −80 °C. Myocardial enzyme cTnl, CK-MB, Mb, and superoxide dismutase (SOD) were measured by enzyme-linked immunosorbent assay (ELISA) (Invitrogen, Carlsbad, CA, USA). Cytokines tumor necrosis factor-α (TNF-α), Interleukin (IL)-1β, IL-6, and interferon (IFN)-γ were detected ELISA (Invitrogen, Carlsbad, CA, USA). A colorimetric assay was used to measure lactate dehydrogenase (LDH) following the instructions of the manufacturer. The tissue was fully ground in an ice bath with sterile PBS, and centrifuged at 4 °C, 3,500 rpm for 10 min. The reactive oxidative species (ROS) and the malondialdehyde (MDA) contents in the myocardial homogenate were then measured. MDA and ROS were detected using the thiobarbituric acid chromogenic method and the chemiluminescence method, respectively.
Western blotting
The heart tissues (0.1 g) from each group of rats were collected and homogenized in a 1 mL protein extraction buffer. The supernatant was collected after centrifugation, and BCA Protein Assay Kit was used to detect protein concentrations. Total protein samples (20 µg) were loaded into 10% sodium dodecyl sulfate-polyacrylamide gel electrophoresis (SDS-PAGE) loading buffer before subsequent transferal to polyvinylidene difluoride (PVDF) membranes. The PVDF membranes were sealed with 5% skimmed milk at 37 °C for 120 min ahead of incubation with the primary antibodies: rabbit β-actin (1:1,000, #4970, Cell Signaling), rabbit anti-cleaved caspase3 (1:1,000, #9662, Cell Signaling), rabbit anti-cleaved caspase9 (1:1,000, #9509, Cell Signaling), rabbit anti-AMPKα1 (1:1,000, #2795, Cell Signaling), rabbit anti-NQO1 (1:1,000, #62262, Cell Signaling), rabbit anti-JNK (1:1,000, #9252, Cell Signaling), rabbit anti-p-JNK (1:1,000, #4668, Cell Signaling), rabbit anti-P65 (1:1,000, #8242, Cell Signaling), rabbit anti-p-P65 (1:1,000, #3033, Cell Signaling) at 4 °C overnight. Subsequently, PVDF membranes were incubated for 60 min at 37 °C with goat anti-rabbit IgG horseradish peroxidase (HRP)-conjugated secondary antibodies. Digital image analysis was conducted with Bio-Rad CFX-96 (Bio-Rad, Hercules, CA, USA) to determine and analyze the density of the bands. β-actin was used as the control.
Flow cytometry analysis
PBMCs were collected for flow cytometry. PBMCs were added to the tube containing the antibody and dye liquor, and incubated for 15 min in the dark. Next, 2 mL of 1× lysing solution was added, and incubation took place for 30 min in the dark, before centrifugation at 1,500 rap/min and removal of the supernatant. Then, once again, 2 mL of PBS was added, before centrifugation at 1,500 rap/min and removal of the supernatant. Finally, 500 µL of PBS was added. The sample was analyzed using a FACS Aria cell sorter (BD Biosciences, New York, USA). The data analysis was performed using FlowJo (Tree Star, OR).
TUNEL staining
The paraffin-embedded heart tissues from the different groups sectioned (to a thickness of 3 µm) with a microtome. The tissues were routinely deparaffinized and 50 µL 3% hydrogen peroxide solution was added. The sections were later incubated at 20 °C for 10 min and rinsed 3 times with phosphate-buffered saline (PBS). Next, the sections were incubated in 50 µL of TUNEL at 37 °C for 60 min. Then, they were incubated once more at 37 °C for 30 min after the addition of 50 µL converter-peroxidase (POD), and rinsed 3 times with PBS. Reagents A, B, and C in the Dolichosbiflorus agglutinin (DBA) kit were added for 10 min to develop the color of the sections. After that, the sections were washed 3 times with PBS, counterstained with hematoxylin for 10 sec, and then mounted in neutral balsam. A microscope was used to observe and count the apoptotic cells (yellowish-brown in color) (28). The images were taken with an OLYMPUS DX51 fluorescence microscope (Olympus, Tokyo, Japan). The integrated option density (IOD) and area (Area) of the myocardial cell area were evaluated in each tissue section, and then obtained the average optical density value (OD = IOD/area). Four slices were selected, and four fields of view were selected for each slice.
Statistical analysis
All experimental data were presented as the mean ± standard deviation (SD). SPSS Statistical analyses were performed using SPSS 22.0 software (SPSS Inc., Chicago, IL, USA). Comparisons between two groups were carried out using Student’s t-test. Differences between the groups were compared by one-way analysis of variance (ANOVA), and Duncan’s method was used as a post-hoc test. Each experiment was performed independently at least in triplicate.
Results
Effect of cardiac systolic function index after treatment with remifentanil
The results of echocardiography showed that HR, LVAP, LVEF, FS, and LVWT were significantly lower in the Isop group than in the control group (HR: 416.13±36.77 vs. 215.43±46.16, Figure 1A; LVAP: 132.11±12.25 vs. 55.18±22.54, Figure 1B; LVEF: 56.81%±8.51% vs. 22.96%±5.61%, Figure 1C; FS: 26.43%±3.99% vs. 7.86%±4.11%, Figure 1D; and LVWT: 0.81±0.06 vs. 0.51±0.05, Figure 1E, *, P<0.05). However, after treatment with remifentanil, it can be seen that HR, LVAP, LVEF, FS, and LVWT were significantly increased compared with the Isop group (#, P<0.05, ##, P<0.01), indicating that remifentanil could improve the heart contraction function.
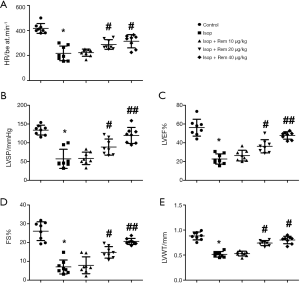
Effect of cardiac pathological and myocardial apoptosis after treatment with remifentanil
As shown in Figure 2A, H&E staining showed that in the Isop group, the cardiomyocytes were disordered, the cells were swollen, and some cells were dissolved. The leading indicators of MI injury include CK-MB, Mb, and cTnl. As shown in Figure 2B, the expression levels of CK-MB, Mb, and cTnl were enhanced compared with the control group (CK-MB: 20.65±9.73 vs. 115.72±15.36; Mb: 25.33±8.12 vs. 146.42±18.29; cTnl: 0.11±0.043 vs. 0.66±0.05, *, P<0.05). After treatment with remifentanil, the expression levels of CK-MB, Mb, and cTnl were decreased compared with the Isop group (#, P<0.05, ##, P<0.01). TUNEL assay showed that myocardial apoptotic cells were significantly downregulated after treatment with remifentanil (Figure 2C). The protein expression levels of cleaved caspase-3 and caspase-9 were markedly enhanced compared with the control group (caspase-3: 0.02±0.005 vs. 0.56±0.06, caspase-9: 0.01±0.06 vs. 0.24±0.043, *, P<0.05). However, there were significant decreases in the protein expression levels of cleaved caspase-3 and caspase-9 compared with those in the Isop group after treatment with remifentanil (#, P<0.05) (Figure 2D). These results suggested that remifentanil could relieve myocardial injury and inhibit myocardial apoptosis.
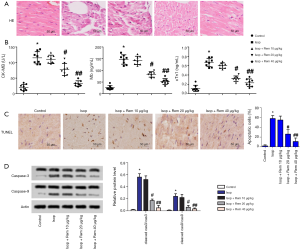
Effect of oxidative stress after treatment with remifentanil
The leading indicators of oxidative stress include ROS, SOD, MDA, and LDH. Compared with the control group, the levels of ROS, MDA, and LDH were noticeably elevated in the Isop group (ROS: 2.66±0.47 vs. 8.61±0.73; MDA: 2.28±0.35 vs. 4.46±0.51; and LDH: 794.52±165.27 vs. 2,258.73±247.93, *, P<0.05), while the level of SOD was significantly decreased (5.68±0.61 vs. 2.25±0.54, *, P<0.05). After treatment with remifentanil, the levels of ROS, MDA, and LDH were noticeably down-regulated compared with the Isop group (#, P<0.05, ##, P<0.01), whereas the level of SOD was significantly increased (Figure 3A). Compared with the control group, the protein expression levels of AMPK α1 and NQO1 were markedly reduced (AMPK α1 0.36±0.06 vs. 0.02±0.015; NQO1: 0.38±0.04 vs. 0.04±0.03, *, P<0.05). However, after treatment with remifentanil. The protein expression levels of AMPK α1 and NQO1 were significantly upregulated in contrast with the Isop group (Figure 3B). These results showed that remifentanil might alleviate the inhibition of oxidative stress.
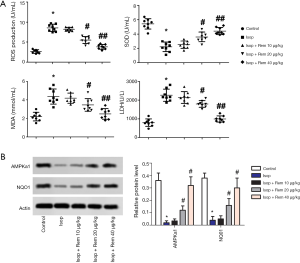
Effect of inflammatory response after treatment with remifentanil
As shown in Figure 4, the ELISA results showed that the MI rat model exhibited significantly increased expression levels of IL-6, TNF-α, and IL-1β compared with the control group (IL-6: 22.15±0.47 vs. 126.73±19.59, Figure 4A; TNF-α: 17.25±8.11 vs. 224.95±25.14, Figure 4B; and IL-1β: 26.75±17.81 vs. 99.57±27.32, Figure 4C, *, P<0.05). There was no significant difference in the expression levels of IL-6, TNF-α, or IL-1β between the remifentanil (10 µg/kg) and the Isop group (P>0.05). After intervention with remifentanil, the TNF-α, IL-1β, and IL-6 levels were decreased in the Isop group (#, P<0.05, ##, P<0.01), especially in the high concentration group. IFN-γ secretion in Th1 cells has been increasingly discussed. The levels of IFN-γ were detected by flow cytometry. As shown in Figure 4D, the results showed that the levels of IFN-γ were markedly enhanced in the Isop group compared with the control group (2.51±1.21 vs. 25.71±4.19, *, P<0.05). However, after treatment with remifentanil, the levels of IFN-γ were significantly downregulated compared with the Isop group. These results suggested that remifentanil could relieve the inflammatory response in the MI model.
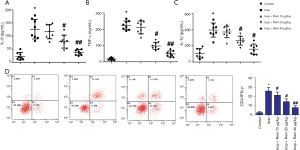
As shown in Figure 5A, the levels of p-JNK and p-p65 were detected by Western blotting. Phosphorylation of JNK and p65 were higher levels in the Isop group compared with the control group (p-JNK: 0.03±0.013 vs. 0.25±0.07; p-p65: 0.04±0.03 vs. 0.68±0.05). The levels of JNK and p65 phosphorylation were notably decreased following remifentanil treatment (#, P<0.05). Interestingly, the levels of JNK and p65 phosphorylation were notably elevated in Isop + LPS group compared with the Isop group (p-JNK: 0.46±0.07 vs. 0.93±0.09; p-p65 0.42±0.06 vs. 0.88±0.07, #, P<0.05) (Figure 5B). H&E staining showed that in the Isop + LPS group, the cardiomyocytes were disordered, the cells were swollen, and some cells were dissolved (Figure 5C). After treatment with remifentanil, the levels of IL-6, TNF-α, SOD, and MDA were downregulated in the remifentanil + Isop + LPS group compared with the Isop + LPS group (&, P<0.05) (Figure 5D,E). These results suggested that remifentanil might cause cardiac dysfunction, lipid peroxidation, and immune disorder via the JNK/NF-KB p65 pathway.
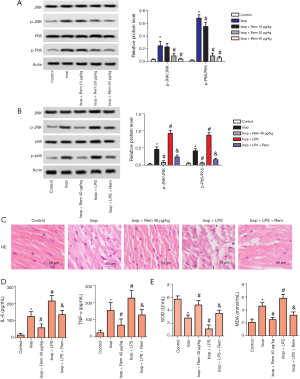
Discussion
MI can cause several heart-related diseases that endanger individuals’ health. In the present study, remifentanil attenuated cardiac dysfunction, lipid peroxidation, and immune disorder in rats with isoproterenol-induced myocardial injury via inhibition of the JNK/NF-KB p65 pathway. Studies have shown myocardial infarction to be inextricably linked to left ventricular dysfunction (29), in which oxygen kills myocardial cells in the left ventricle, leading to the eventual thinning of the ventricular wall (30). The cardiac systolic function can be reflected by HR, LVAP, LVEF, FS, and LVWT. The present results showed that remifentanil improved HR, LVAP, LVEF, FS, and LVWT in the Isop group. Feng et al. (31) showed that the cardiac EF and FS of rats with MI was significantly lower, which is consistent with our findings. Furthermore, H&E staining showed that remifentanil attenuated the pathological damage caused by MI. Therefore, remifentanil could restore the contractile function of cardiomyocytes in rats.
Mainly found in cardiac muscle, cTnI and CK-MB, which are elevated by myocardium-specific damage, are helpful markers in diagnosing MI (32,33). This study found that when remifentanil was administered, there was an ameliorative effect on myocardial injury caused by isoproterenol induced MI in the rats. A significant increase in serum cTnl, CK-MB, Mb, and LDH in the samples confirmed the rats had developed MI. Caspases are the initiators and executors of cell apoptosis. Caspase-3, which is responsible for regulating cardiomyocytes, is the most critical apoptotic protease, serving an essential role in the downstream of cascade junctions and featuring prominently in the process of cell death (28,34).
Oxidative stress is mechanistically involved in the pathogenesis of ISO-induced myocardial injury (3). Following the findings of earlier research, the oxidative stress markers are upregulated in Isop-treated rats (35). The AMP-activated protein kinase (AMPK) signaling pathway performs a crucial role in intracellular adaptation to energy stress in MI. AMPKα1 and α2 are primarily found in cardiomyocytes in the cytoplasm and nucleus, respectively (36). NAD(P)H: quinone oxidoreductase 1 (NQO1) is regarded as a highly inducible cytoprotective gene that behaves as a regulator of the reactive oxygen species (ROS) generation. Earlier research has showed that NQO1 significantly influences the autophagy process, supporting the hypothesis that autophagy takes up a protective role under oxidative stress conditions (37). The antioxidant activities of SOD reflect the cellular capacity to scavenge oxygen free radicals (38). In this study, the levels of ROS, MDA, and LDH were remarkably elevated in the Isop group in comparison to the control group, while there was a significant decrease in SOD levels. Treatment with remifentanil, the levels of ROS, MDA, and LDH were noticeably repressed compared with the Isop group, whereas the levels of SOD were significantly increased. The protein expression levels of AMPK α1 and NQO1 were significantly upregulated after treatment with remifentanil in contrast with the Isop group. These results suggested that remifentanil might alleviate oxidative stress.
Previous research showed that after short periods of MI, some signs of the activation of neutrophils and the complement system were observed (39). By activating the JNK signaling pathway, as well as the production of proinflammatory cytokines, cell death is also induced via the intrinsic and external apoptosis pathways (40). At the same time, NF-κB activation induces different proinflammatory cytokines (including TNF-α, IL-6, and IL-1β) and is involved in physiological processes including inflammation, cell proliferation, and apoptosis (41). In this study, the ELISA results showed that the MI rat model showed significantly increased expression levels of IL-6, TNF-α, IL-1β, and IFN-γ compared with the control group. However, after treatment with remifentanil, the levels of IL-6, TNF-α, IL-1β, and IFN-γ were decreased compared with the Isop group. These results suggested that remifentanil can relieve the inflammatory response.
The degree of JNK and p65 phosphorylation was notably decreased following remifentanil treatment. Notably, research has shown that remifentanil exerts a protective effect in lipopolysaccharide (LPS)-induced acute lung injury in rats via the downregulation of the nuclear factor (NF)-κB signaling pathway and acute-phase cytokines involved in inflammation (42). Interestingly, treadmill and wheel exercise protect against inflammation through the regulation of JNK/NF-kappaB signaling in experimental models of knee osteoarthritis (43). These results suggested that remifentanil could attenuate cardiac dysfunction, lipid peroxidation, and immune disorder via the JNK/NF-KB p65 pathway.
Acknowledgments
Funding: None.
Footnote
Guideline Checklist: The authors have completed the ARRIVE guideline checklist. Available at: http://dx.doi.org/10.21037/atm-20-3134.
Data Sharing Statement: Available at http://dx.doi.org/10.21037/atm-20-3134.
Conflicts of Interest: All authors have completed the ICMJE uniform disclosure form (available at: http://dx.doi.org/10.21037/atm-20-3134). The authors have no conflicts of interest to declare.
Ethical Statement: The authors are accountable for all aspects of the work in ensuring that questions related to the accuracy or integrity of any part of the work are appropriately investigated and resolved. The study was approved by Sichuan Provincial People’s Hospital.
Open Access Statement: This is an Open Access article distributed in accordance with the Creative Commons Attribution-NonCommercial-NoDerivs 4.0 International License (CC BY-NC-ND 4.0), which permits the non-commercial replication and distribution of the article with the strict proviso that no changes or edits are made and the original work is properly cited (including links to both the formal publication through the relevant DOI and the license). See: https://creativecommons.org/licenses/by-nc-nd/4.0/.
References
- Zhou M, Wang H, Zeng X, et al. Mortality, morbidity, and risk factors in China and its provinces, 1990-2017: a systematic analysis for the Global Burden of Disease Study 2017. Lancet 2019;394:1145-58. [Crossref] [PubMed]
- Ni L, Wehrens XH. Cardiac troponin I—more than a biomarker for myocardial ischemia? Ann Transl Med 2018;6:S17. [Crossref] [PubMed]
- Boshra V, Atwa A. Effect of cerebrolysin on oxidative stress-induced apoptosis in an experimental rat model of myocardial ischemia. Physiol Int 2016;103:310-20. [Crossref] [PubMed]
- Fan S, Sun JB, Li R, et al. Lycopene protects myocardial ischemia injury through anti-apoptosis and anti-oxidative stress. Eur Rev Med Pharmacol Sci 2019;23:3096-104. [PubMed]
- Wattanapitayakul SK, Bauer JA. Oxidative pathways in cardiovascular disease: roles, mechanisms, and therapeutic implications. Pharmacol Ther 2001;89:187-206. [Crossref] [PubMed]
- Houson HA, Nkepang GN, Hedrick AF, et al. Imaging of isoproterenol-induced myocardial injury with (18)F labeled Fluor glucaric acid in a rat model. Nucl Med Biol 2018;59:9-15. [Crossref] [PubMed]
- Panda S, Kar A, Biswas S. Preventive effect of Agnucastoside C against Isoproterenol-induced myocardial injury. Sci Rep 2017;7:16146. [Crossref] [PubMed]
- Yang LQ, Tao KM, Liu YT, et al. Remifentanil preconditioning reduces hepatic ischemia-reperfusion injury in rats via inducible nitric oxide synthase expression. Anesthesiology 2011;114:1036-47. [Crossref] [PubMed]
- Glass PS, Gan TJ, Howell S. A review of the pharmacokinetics and pharmacodynamics of remifentanil. Anesth Analg 1999;89:S7-14. [Crossref] [PubMed]
- Westmoreland CL, Hoke JF, Sebel PS, et al. Pharmacokinetics of remifentanil (GI87084B) and its major metabolite (GI90291) in patients undergoing elective inpatient surgery. Anesthesiology 1993;79:893-903. [Crossref] [PubMed]
- Komatsu R, Turan AM, Orhan-Sungur M, et al. Remifentanil for general anaesthesia: a systematic review. Anaesthesia 2007;62:1266-80. [Crossref] [PubMed]
- Hofbauer R, Frass M, Gmeiner B, et al. Effects of remifentanil on neutrophil adhesion, transmigration, and intercellular adhesion molecule expression. Acta Anaesthesiol Scand 2000;44:1232-7. [Crossref] [PubMed]
- Sacerdote P, Gaspani L, Rossoni G, et al. Effect of the opioid remifentanil on cellular immune response in the rat. Int Immunopharmacol 2001;1:713-9. [Crossref] [PubMed]
- Cui C, Yu F, Yin S, et al. Remifentanil Preconditioning Attenuates Hepatic Ischemia-Reperfusion Injury in Rats via Neuronal Activation in Dorsal Vagal Complex. Mediators Inflamm 2018;2018:3260256.
- Kim CH, Jeong SS, Yoon JY, et al. Remifentanil reduced the effects of hydrogen peroxide-induced oxidative stress in human keratinocytes via autophagy. Connect Tissue Res 2017;58:597-605. [Crossref] [PubMed]
- Sheng M, Zhang G, Wang J, et al. Remifentanil Induces Cardio Protection Against Ischemia/Reperfusion Injury by Inhibiting Endoplasmic Reticulum Stress Through the Maintenance of Zinc Homeostasis. Anesth Analg 2018;127:267-76. [Crossref] [PubMed]
- Li J, Hu HP, Li Y, et al. Influences of remifentanil on myocardial ischemia-reperfusion injury and the expressions of Bax and Bcl-2 in rats. Eur Rev Med Pharmacol Sci 2018;22:8951-60. [PubMed]
- Qu ZJ, Qu ZJ, Zhou HB, et al. Protective effect of remifentanil on myocardial ischemia-reperfusion injury through Fas apoptosis signaling pathway. Eur Rev Med Pharmacol Sci 2019;23:5980-6. [PubMed]
- Ronco C. Lipopolysaccharide (LPS) from the cellular wall of Gram-negative bacteria, also known as endotoxin, is a key molecule in the pathogenesis of sepsis and septic shock. Preface. Blood Purif 2014;37 Suppl 1:1. [Crossref] [PubMed]
- Neri M, Riezzo I, Pomara C, et al. Oxidative-Nitrosative Stress and Myocardial Dysfunctions in Sepsis: Evidence from the Literature and Postmortem Observations. Mediators Inflamm 2016;2016:3423450.
- Su Q, Yao J, Sheng C. Geniposide Attenuates LPS-Induced Injury via Up-Regulation of miR-145 in H9c2 Cells. Inflammation 2018;41:1229-37. [Crossref] [PubMed]
- Lei S, Zhang Y, Su W, et al. Remifentanil attenuates lipopolysaccharide-induced oxidative injury by downregulating PKCβ2 activation and inhibiting autophagy in H9C2 cardiomyocytes. Life Sci 2018;213:109-15. [Crossref] [PubMed]
- Zhang JN, Ma Y, Wei XY, et al. Remifentanil Protects against Lipopolysaccharide-Induced Inflammation through PARP-1/NF-κB Signaling Pathway. Mediators Inflamm 2019;2019:3013716.
- Win S, Than TA, Kaplowitz N. The Regulation of JNK Signaling Pathways in Cell Death through the Interplay with Mitochondrial SAB and Upstream Post-Translational Effects. Int J Mol Sci 2018. [Crossref] [PubMed]
- Pordanjani SM, Hosseinimehr SJ. The Role of NF-kB Inhibitors in Cell Response to Radiation. Curr Med Chem 2016;23:3951-63. [Crossref] [PubMed]
- Song XD, Feng JP, Yang RX. Alamandine protects rat from myocardial ischemia-reperfusion injury by activating JNK and inhibiting NF-kappaB. Eur Rev Med Pharmacol Sci 2019;23:6718-26. [PubMed]
- Zhang Q, Deng Y, Lai W, et al. Maternal inflammation activated ROS-p38 MAPK predisposes offspring to heart damages caused by isoproterenol via augmenting ROS generation. Sci Rep 2016;6:30146. [Crossref] [PubMed]
- Wang Q, Cui Y, Lin N, et al. Correlation of cardiomyocyte apoptosis with duration of hypertension, severity of hypertension and caspase-3 expression in hypertensive rats. Exp Ther Med 2019;17:2741-5. [PubMed]
- Nagaoka K, Matoba T, Mao Y, et al. A New Therapeutic Modality for Acute Myocardial Infarction: Nanoparticle-Mediated Delivery of Pitavastatin Induces Cardioprotection from Ischemia-Reperfusion Injury via Activation of PI3K/Akt Pathway and Anti-Inflammation in a Rat Model. PLoS One 2015;10:e0132451. [Crossref] [PubMed]
- Davies MJ, Thomas AC. Plaque fissuring--the cause of acute myocardial infarction, sudden ischaemic death, and crescendo angina. Br Heart J 1985;53:363-73. [Crossref] [PubMed]
- Feng X, Zhang R, Li J, et al. Syringapinnatifolia Hemsl. fraction protects against myocardial ischemic injury by targeting the p53-mediated apoptosis pathway. Phytomedicine 2019;52:136-46. [Crossref] [PubMed]
- Loh HK, Sahoo KC, Kishore K, et al. Effects of thalidomide on isoprenaline-induced acute myocardial injury: a haemodynamic, histopathological and ultrastructural study. Basic Clin Pharmacol Toxicol 2007;100:233-9. [Crossref] [PubMed]
- Rosalki SB, Roberts R, Katus HA, et al. Cardiac biomarkers for detection of myocardial infarction: perspectives from past to present. Clin Chem 2004;50:2205-13. [Crossref] [PubMed]
- Liu M, Sun L, Jiang B, et al. Effect of nucleolin on cardiac cell apoptosis in Type 2 diabetic cardiomyopathy mice. Zhong Nan Da Xue Xue Bao Yi Xue Ban 2017;42:241-5. [PubMed]
- Ribeiro DA, Buttros JB, Oshima CT, et al. Ascorbic acid prevents acute myocardial infarction induced by isoproterenol in rats: role of inducible nitric oxide synthase production. J Mol Histol 2009;40:99-105. [Crossref] [PubMed]
- Wang Y, Yang Z, Zheng G, et al. Metformin promotes autophagy in ischemia/reperfusion myocardium via cytoplasmic AMPKα1 and nuclear AMPKα2 pathways. Life Sci 2019;225:64-71. [Crossref] [PubMed]
- Kim TW, Kim YJ, Kim HT, et al. NQO1 Deficiency Leads Enhanced Autophagy in Cisplatin-Induced Acute Kidney Injury Through the AMPK/TSC2/mTOR Signaling Pathway. Antioxid Redox Signal 2016;24:867-83. [Crossref] [PubMed]
- Remião F, Carmo H, Carvalho FD, et al. Inhibition of Glutathione Reductase by Isoproterenol Oxidation Products. J Enzyme Inhib 1999;15:47-61. [Crossref] [PubMed]
- Pasqui AL, Bova G, Renzo M, et al. Activation of inflammatory/immune response after myocardial ischemia; evidence in coronary angioplasty (PTCA) and stress test. Nutrition, Metabolism and Cardiovascular Diseases 1998;8:37-45.
- Zhu J, Shen W, Gao L, et al. PI3K/Akt-independent negative regulation of JNK signaling by MKP-7 after cerebral ischemia in rat hippocampus. BMC Neurosci 2013;14:1. [Crossref] [PubMed]
- Chen S, Yin ZJ, Jiang C, et al. Asiaticoside attenuates memory impairment induced by transient cerebral ischemia-reperfusion in mice through anti-inflammatory mechanism. Pharmacol Biochem Behav 2014;122:7-15. [Crossref] [PubMed]
- Zhang Y, Du Z, Zhou Q, et al. Remifentanil attenuates lipopolysaccharide-induced acute lung injury by downregulating the NF-kappaB signaling pathway. Inflammation 2014;37:1654-60. [Crossref] [PubMed]
- Chen L, Lou Y, Pan Z, et al. Treadmill and wheel exercise protect against JNK/NF-kappaB induced inflammation in experimental models of knee osteoarthritis. Biochem Biophys Res Commun 2020;523:117-22. [Crossref] [PubMed]