3D printing of intracranial artery stenosis based on the source images of magnetic resonance angiograph
Introduction
In the past decades, neuroimaging has dominated the clinical practice as well as scientific research of neurological diseases. The information of brain disease of a patient can be displayed on films or screens, facilitating the diagnosis. This, however, may be changed by the emerging techniques of three dimensional (3D) printing and/or rapid prototyping. These techniques deposit materials, such as plastic or metal, onto one another by layers to construct 3D objects. Several studies have reported that 3D printing can fabricate human organs with high resolution based on medical images (1-3). It means the information of a patient is available ‘by touch’.
The brain is the least accessible organ in the human body. To the best of our knowledge, 3D printing techniques for brain diseases have not been widely studied. In this work, we attempted to ‘print’ intracranial arteries from magnetic resonance imaging. We hypothesize research 3D printing is a useful technique, which is feasible in the field of cerebrovascular diseases.
Methods
Patients
Two Chinese adult patients were enrolled from Peking Union Medical College Hospital in 2014. One had unilateral middle cerebral artery (MCA) stenosis on magnetic resonance angiography (MRA). The other had a MCA stenosis and contra-lateral MCA occlusion.
MRA source images
Three dimensional MRA was performed on each patient. The parameters were as follows: TR/TE 27/6.9, flip angle (FA): 20°, FOV 24 cm × 16 cm, thickness 1.4 mm, matrix 384×320, number of excitations (NEX): 1. ZIP technique was used to improve images resolution. Source images were reconstructed from the raw data with resolution: 0.4 mm × 0.3 mm, thickness 1.4 mm.
3D vascular model
The MRA data were stored in the standard DICOM (Digital Imaging and Communication in Medicine) format. The data were then processed in a personal computer (Hewlett-Packard 8180 with Intel i7 processor, USA). Boundaries of cerebral arteries on each slide of MRA image was segmented by a semi-automated software, Mimics (a suite of software, Materialise NV, Belgium) and then manually examined and refined by an expert with clinical training. We then applied our scale-adaptive vascular modeling method (3,4) to reconstruct the 3D geometry of vascular surfaces with high-accuracy. Please refer to our previous work (3,4) for detailed description. The resultant models of 3D vascular surface were converted to STL (STereoLithography; surface data as an aggregation of triangular meshes) format.
3D printing
The STL files were loaded in Magics (version 9.51, a suite of software, Materialise NV, Belgium) to select the regions of interest (ROI) with cerebral stenosis. Figure 1 shows the 3D vascular models and the selected ROI in two patients. Stenosis is indicated by the circles and arrows in Figure 1B and 1D, respectively. In order to allow for a visual inspection by neurologists, the models were magnified by 10 times in dimension resulting in sizes of 108 mm × 59 mm × 69.5 mm for patient 1 and 121 mm × 54 mm × 65 mm for patient 2, respectively and then sent to a 3D printer (Objet350 Connex, Stratasys, USA) for printing, which allows for jetting multiple model materials simultaneously. It offers the ability to print parts and assemblies made of multiple model materials, with different mechanical or physical properties. We selected the transparent ABS resin (VeroClear, Stratasys, USA) for the blood vessel and a white ABS resin (VeroWhite Plus, Stratasys, USA) for the supports, respectively. The latter is required to enhance the strength of the 3D model of blood vessel. The printing resolution is 30 μm and the fabrication time are 5:24 h and 5:39 h for patient 1 and patient 2, respectively. The model was formed together with automatically generated base and supports, which was removed after completion of the 3D printing.
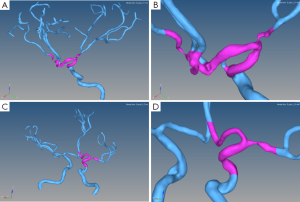
Quality evaluations
The 3D printing results were presented to eight clinicians (seven senior neurologists and one senior radiologist). A survey was performed to evaluate the accuracy of 3D printing results as compared with MRA findings (4 grades, grade 1: consistent with MRA and provide additional visual information; grade 2: consistent with MRA; grade 3: not consistent with MRA; grade 4: not consistent with MRA and provide probable misleading information). If a 3D printing vessel segment was ideally matched to the MRA findings (grade 2 or 1), a successful 3D printing was defined.
Results
Figure 2 showed the 3D printing results based on MRA source images. Seven responders marked “grade 1” to 3D printing results, while one responder marked “grade 4”. Therefore, 87.5% of the clinicians considered the 3D printing were successful.
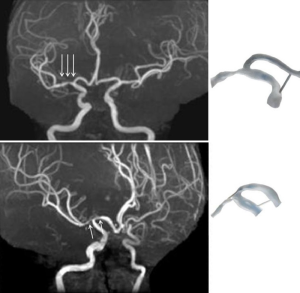
Discussion
Intracranial stenosis is highly prevalent in stroke patients of Asian, Hispanic and African ancestry. While there has been enormous progress in the treatments of extra-cranial occlusive diseases, the effective managements of intracranial stenosis remain lacking. The pathophysiology and hemodynamics of intracranial stenosis has not been well studied. An important barrier for the investigations of intracranial stenosis is the difficulty in establishing ideal in vivo and in vitro models.
In this study, based on MRA images, the 3D printing technique was developed for printing intracranial vessels of individual patients. Most of the clinicians in our survey agreed the results of 3D printing models were consistent with the findings of MRA. This is encouraging, though only segments of stenotic vessels were printed. There are at least two potential applications of current 3D printing intracranial vessels. Firstly, 3D printing intracranial vessels are promising to establish in vitro intracranial stenosis model based on in vivo information. The individual intracranial hemodynamics can therefore be evaluated in vitro. For example, the intra-lumen sheer stress assessment is available when the measurements of lumen stenosis and artificial blood flow are both available in vitro. However, it should be noted that current 3D technique is still evolving to be able to ultimately simulate model of intracranial vessels, given ‘the lumen of 3D printing vessels’ cannot be evaluated in this study. And the physical properties of the printed ‘vessel wall’ are different from the human vessels. Secondly, 3D printing vessels can be very helpful for medical was education. Most of the survey participants agreed that there additional visual information, suggesting the vessel ‘in touch’ may be more intuitive. For interventional therapy, the 3D printing intracranial stenotic vessels may be even more meaningful for surgical planning.
In conclusion, our pilot study confirms the feasibility of using 3D printing technique in the studies of intracranial artery diseases. Further investigations are warranted to optimize this technique and translate it into research and clinical practice.
Acknowledgements
Funding: This work was supported by National Natural Science Foundation of China (No. 61272328).
Disclosure: The authors declare no conflict of interest.
References
- Gerstle TL, Ibrahim AM, Kim PS, et al. A plastic surgery application in evolution: three-dimensional printing. Plast Reconstr Surg 2014;133:446-51. [PubMed]
- Mashiko T, Otani K, Kawano R, et al. Development of Three-Dimensional Hollow Elastic Model for Cerebral Aneurysm Clipping Simulation Enabling Rapid and Low Cost Prototyping. World Neurosurg 2013. [Epub ahead of print].
- Schubert C, van Langeveld MC, Donoso LA. Innovations in 3D printing: a 3D overview from optics to organs. Br J Ophthalmol 2014;98:159-61. [PubMed]
- Wu J, Wei M, Li Y, et al. Scale-adaptive surface modeling of vascular structures. Biomed Eng Online 2010;9:75. [PubMed]