Large artery: an important target for cerebral small vessel diseases
Introduction
“Cerebral small vessel disease (CSVD)” is a heterogeneous entity, including the diseases affecting the perforating cerebral arterioles, capillaries, and venules (1). Clinically, CSVD may present with acute focal neurological symptoms, progressive cognitive decline, gait disturbance and dementia. On the other hand, a great number of patients with CSVD are long-term asymptomatic. The varied clinical presentations are considered due to different underlying pathologies and disease stages (1). However, given the relatively low fatality, limited autopsy studies on CSVD at different stages are available (2). While in vivo neuroimaging techniques have been highly developed, cerebral small vessel wall lesions remain invisible.
Interestingly, while CSVD is actually not well understood, it has been treated effectively. The widely accepted treatments for CSVD, such as anti-hypertension, are largely similar to those of large artery diseases (3). Large arteries are the upstream vessels of cerebral small vessels, through which blood flow is transported. Both large arteries and cerebral small vessels are exposed to systematic vascular risk factors, especially aging and hypertension. Theoretically, large artery and small vessels should correlate closely. More and more studies suggested large artery lesions are involved in CVSD, and are an important target for CVSD.
Large artery atherosclerosis correlates with lacunar infarcts
Lacunar infarcts often present with acute non-fatal neurological symptoms. The available histology data of lacunar infarcts, most from Miller Fisher’s work in 1950s-1970s, refer to the infarcts resulting from the occlusions of small perforating cerebral arteries. Fisher performed meticulous pathological dissections along the perforating vessels and identified “segmental arteriolar disorganization”, or “lipohyalinosis” (4,5). Recent in vivo imaging studies suggested the derangement of the blood-brain barrier might be the primary underlying initiating cause of these small vessel structural changes (6).
In 1979, Fisher reported his autopsy results of ten patients with 11 capsular infarcts (7). In one case, the penetrating arteries were found to be obstructed at their orifices by an atheroma in the superior division of the middle cerebral artery (MCA). This study first provide evidence that large artery atheroma can cause a lacunar infarct. This mechanism is believed to account for a small percentage of lacunar infarcts. With the development of non-invasive imaging techniques, it is known intracranial atherosclerosis play a more important role in causing strokes than expected, especially in patients with Asian, Africa, or Hispanic ancestry (8). A recent autopsy study suggested intracranial atherosclerosis was even under-estimated in Caucasian population (9). By high-resolution magnetic resonance imaging (HRMRI), our group first reported in vivo evidence that intracranial atheroma can occur in MCA without significant stenosis (10). Emerging evidence has suggested the intracranial non-stenotic atheroma may cause a lacunar stroke. Klein et al. studied 26 patients with paramedian pontine infarction and 15 with small medial pontine lesion (11). Normal basilar angiograms were revealed in 46-65% patients, but as high as 73-77% patients have basilar artery atherosclerosis on HRMRI. Atheroma was detected in 9 of another 15 patients (60%) with acute lacunar infarcts but without intracranial stenosis (12). All the ischemic lesions were ipsilateral to the atheroma and all plaques responsible for acute symptomatic lacunar infarction were enhanced in contrast-enhanced T1-weighted HRMR images, suggesting potential intra-plaque inflammation. In our recent study, of the 114 vessels of 57 consecutive patients with an acute deep brain infarct, eccentric plaques were identified on 66 slices of 26 vessels ipsilateral to and on 62 slices of 24 vessels contralateral to the ischemic lesions (13). Superior-wall plaque was more frequently observed ipsilateral than contralateral to the infarct (69.2% vs. 37.5%) (Figure 1), while ventral-wall plaque was more commonly contralateral than ipsilateral to the infarct (62.5% vs. 30.8%) (13). Given most penetrating arteries arise from dorsal-upper wall of MCA, the results of MCA plaque distribution in deep brain infarcts provide further support of the role intracranial atheroma in causing a stroke.
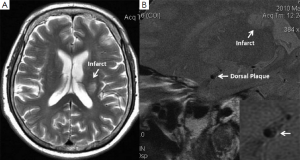
It should be noted, however, that current HRMRI couldn’t provide direct image evidence that MCA plaque blocking the orifices of criminal penetrating arteries. The plaque on HRMRI may even be an innocent bystander since co-existence of plaque within penetrating arteries or lipohyalinosis couldn’t be excluded. Nevertheless, the intracranial atheroma in patients with lacunar infarcts may at least suggest a co-existence of large artery atherosclerosis. It provides a clue accounting for why the effective treatments for lacunar stroke are basically the same with those of large-artery stroke. In clinical trials, although the recruited patients with lacunar stroke have heterogeneous underlying mechanisms, it is usually difficult to in vivo identify the pathogenesis individually before treatment. If a high percentage of lacunar stroke was caused by non-stenotic intracranial atherosclerosis as revealed in HRMRI studies, the effects of treatment may tend to be similar between lacunar stroke and large-artery stroke. HR-MRI is, therefore, valuable in stratifying patients with or without intracranial plaques and helpful for developing individual treatment strategy.
The cross-talk between large artery and small vessels in WMLs
The white matter lesions (WMLs), initially proposed as a descriptive term “leukoaraiosis” by Hachinski et al., is characterized by an age-related, abnormal appearance of the brain subcortical white matter on imaging, especially in the territory of the centrum ovale (14). Patients with WMLs can be asymptomatic, or present with cognitive decline and gait disturbance (15). The pathological findings of WML including myelin pallor, enlarged perivascular spaces, tissue infarction, gliosis, and axonal loss (2,15). Tortuous arterioles with wall thickening, periventricular venous collagenosis, and capillaries loss can be revealed in different stages of WML (2,15). Hypertension and age are the most important risk factors of WML (15).
In 2005, O’Rourke and Safar tried to establish the relationship between microvascular damage in brain and kidney and indices of age and hypertension (pulse pressure, aortic pulse wave velocity, and augmentation index) (16). They hypothesized the exposure of small vessels to highly pulsatile pressure and flow, usually induced by large artery stiffening, contributes to the pathogenesis of renal and brain diseases (16). This hypothesis has been viewed as the underlying mechanisms of WMLs and supported by several studies. In the Rotterdam Scan Study, Poels et al. analyzed the data from 1,460 participants (mean age: 58.2 years) who underwent aortic pulse wave velocity measurement and brain MRI scanning (17). The increased arterial stiffness, represented by aortic pulse wave velocity, is associated with a larger volume of WMLs. Results from the Oxford Vascular Study including patients with a transient ischemic attack or minor-stroke, suggested that MCA pulsatility was the strongest physiological correlate of leukoaraiosis, dependent on aortic and MCA stiffness but independent of age (18). These further suggest that increased arterial pulsatility due to large artery stiffening can transmit to the cerebral small vessel and thus participates in the pathogenesis of leukoaraiosis related to CSVD.More recently, in “Lothian Birth Cohort 1936 cohort study”, Aribisala et al. obtained blood pressure (BP) measurements from 694 community-dwelling subjects at mean ages 69.6 (±0.8) years and again at 72.6 (±0.7) years, plus brain MRI and internal cerebral artery ultrasound at age 73±1 years (19). WMLs were associated with history of hypertension and with higher internal cerebral artery pulsatility index after adjusting for BP and correction for multiple testing. The authors proposed that hypertension and WMLs might either associate indirectly because hypertension increases arterial stiffness that leads to WML over time, or co-associate through advancing age and stiffer vessels, or both (19). These results should be interpreted with caution because causal effects of artery stiffening in WML have not been established. Until now, there has been no cohort study observing the direct relationship between WML and large artery stiffness, and no randomized trial to test antihypertensive therapy in preventing WMLs progression by reducing large artery stiffness.
On the other hand, the effects of small vessels to large arteries should not be ignored. It is known that elevated pulse pressure can stimulate hypertrophy, increased media: lumen ratio and rarefaction in the microcirculation, leading to increased peripheral resistance. The increased peripheral resistance is the major factor responsible for an increase in mean blood pressure, which increases large artery stiffness and atherosclerosis (20). Several studies repeatedly confirmed that increased cerebral small vessel resistance, represented by pulsatility index, is increased in WMLs (18,21). In our recent HRMRI study, both MCA wall thickening and larger MCA lumen was only observed in WMLs as compared to the controls (13). The findings were similar to basilar artery dolichoectasia (visible artery elongation and dilation in autopsies and vascular imaging study), which is also associated with CSVD (22,23). In another study, Uçeyler et al. studied 87 patients with Fabry disease, a well-known inherited systematic small vessel disease often presenting with posterior circulation infarcts (24). They found male Fabry patients had a larger basilar artery and posterior cerebral artery diameter compared to male controls, independent of disease severity as measured by renal function and did not lead to changes in arterial blood flow properties. The mechanisms of intracranial artery lumen dilation in CVSD are not clear, we proposed that “intracranial artery failure” occurs secondary to systematic large artery injury and cerebrovascular resistance overload, i.e., failing to deliver blood flow into the brain efficiently and maintain brain perfusion (13). However, it is not known when and how intracranial artery dilation in relation to CVSD develops. Evidence from large sample cohort studies is required as well.
Translational concepts and future direction
While structurally and functionally connected, large arteries and cerebral small vessels are physiologically correlated. In old era, large vessel diseases and small vessel diseases are investigated separately. More and more evidence suggested the two diseases are inter-mingled and should be considered together. The cross-talks between the small and large artery may develop a vicious circle: increased small vessel damages and peripheral resistance (including the part from cerebral small vessels), increased blood pressure, increased large artery stiffness and atherosclerosis, and then, more increased small vessel damages (including CSVD) (20,25). Traditional therapy such as anti-hypertension, could have effects on both levels, reduce both peripheral resistance and central pulse pressure, and thus reduce target organ damages (20).
Based on what we have known, it is the time to change our concept and give more concerns to “pan-vessel diseases”. When a deep brain lacunar infarct occur, it is good to perform HRMRI to screen intracranial atherosclerosis, which requires more intensified treatment. It may be the appropriate strategy to keep longitudinal monitoring of the trend of large artery stiffness and give intervention such as aggressive blood pressure control to prevent WMLs occurrence or progression. The data from cohort studies are therefore required, especially the biomarkers of diseased large artery stiffness. In the future, when randomized clinical trials are performed, the end points should take both large artery and small vessel damages into consideration. The two diseases are in the same boat. In order to save one, we have to save both.
Acknowledgements
I appreciate Dr. Ming Yao for her kindly proofreading.
Funding: Supported by Peking Union Medical College (PUMC) Youth Fund and the Fundamental Research Funds for the Central Universities of China (NCET-12-0069).
Disclosure: The author declares no conflict of interest.
References
- Hutchinson C, Elbers J, Halliday W, et al. Treatment of small vessel primary CNS vasculitis in children: an open-label cohort study. Lancet Neurol 2010;9:1078-84. [PubMed]
- Brown WR, Thore CR. Review: cerebral microvascular pathology in ageing and neurodegeneration. Neuropathol Appl Neurobiol 2011;37:56-74. [PubMed]
- Kernan WN, Ovbiagele B, Black HR, et al. Guidelines for the prevention of stroke in patients with stroke and transient ischemic attack: a guideline for healthcare professionals from the american heart association/american stroke association. Stroke 2014;45:2160-236. [PubMed]
- Fisher CM. Lacunes: small, deep cerebral infarcts. Neurology 1965;15:774-84. [PubMed]
- Fisher CM. Lacunar strokes and infarcts: a review. Neurology 1982;32:871-6. [PubMed]
- Wardlaw JM, Smith C, Dichgans M. Mechanisms of sporadic cerebral small vessel disease: insights from neuroimaging. Lancet Neurol 2013;12:483-97. [PubMed]
- Fisher CM. Capsular infarcts: the underlying vascular lesions. Arch Neurol 1979;36:65-73. [PubMed]
- Wong LK. Global burden of intracranial atherosclerosis. Int J Stroke 2006;1:158-9. [PubMed]
- Mazighi M, Labreuche J, Gongora-Rivera F, et al. Autopsy prevalence of intracranial atherosclerosis in patients with fatal stroke. Stroke 2008;39:1142-7. [PubMed]
- Li ML, Xu WH, Song L, et al. Atherosclerosis of middle cerebral artery: evaluation with high-resolution MR imaging at 3T. Atherosclerosis 2009;204:447-52. [PubMed]
- Klein IF, Lavallée PC, Mazighi M, et al. Basilar artery atherosclerotic plaques in paramedian and lacunar pontine infarctions: a high-resolution MRI study. Stroke 2010;41:1405-9. [PubMed]
- Chung JW, Kim BJ, Sohn CH, et al. Branch atheromatous plaque: a major cause of lacunar infarction (high-resolution MRI study). Cerebrovasc Dis Extra 2012;2:36-44. [PubMed]
- Xu WH, Li ML, Niu JW, et al. Intracranial artery atherosclerosis and lumen dilation in cerebral small-vessel diseases: a high-resolution MRI Study. CNS Neurosci Ther 2014;20:364-7. [PubMed]
- Hachinski VC, Potter P, Merskey H. Leuko-araiosis. Arch Neurol 1987;44:21-3. [PubMed]
- Auriel E, Bornstein NM, Berenyi E, et al. Clinical, radiological and pathological correlates of leukoaraiosis. Acta Neurol Scand 2011;123:41-7. [PubMed]
- O’Rourke MF, Safar ME. Relationship between aortic stiffening and microvascular disease in brain and kidney: cause and logic of therapy. Hypertension 2005;46:200-4. [PubMed]
- Poels MM, Zaccai K, Verwoert GC, et al. Arterial stiffness and cerebral small vessel disease: the Rotterdam Scan Study. Stroke 2012;43:2637-42. [PubMed]
- Webb AJ, Simoni M, Mazzucco S, et al. Increased cerebral arterial pulsatility in patients with leukoaraiosis: Arterial stiffness enhances transmission of aortic pulsatility. Stroke 2012;43:2631-6. [PubMed]
- Aribisala BS, Morris Z, Eadie E, et al. Blood pressure, internal carotid artery flow parameters, and age-related white matter hyperintensities. Hypertension 2014;63:1011-8. [PubMed]
- Laurent S, Briet M, Boutouyrie P. Large and small artery cross-talk and recent morbidity-mortality trials in hypertension. Hypertension 2009;54:388-92. [PubMed]
- Mok V, Ding D, Fu J, et al. Transcranial Doppler ultrasound for screening cerebral small vessel disease: a community study. Stroke 2012;43:2791-3. [PubMed]
- Gutierrez J, Sacco RL, Wright CB. Dolichoectasia-an evolving arterial disease. Nat Rev Neurol 2011;7:41-50. [PubMed]
- Pico F, Labreuche J, Touboul PJ, et al. Intracranial arterial dolichoectasia and small-vessel disease in stroke patients. Ann Neurol 2005;57:472-9. [PubMed]
- Uçeyler N, Homola GA, Guerrero González H, et al. Increased arterial diameters in the posterior cerebral circulation in men with Fabry disease. PLoS One 2014;9:e87054. [PubMed]
- Park JB, Schiffrin EL. Small artery remodeling is the most prevalent (earliest?) form of target organ damage in mild essential hypertension. J Hypertens 2001;19:921-30. [PubMed]