Levels of plasma cytokine in patients undergoing neoadjuvant androgen deprivation therapy and external beam radiation therapy for adenocarcinoma of the prostate
Introduction
Prostate cancer (PC) is the second most commonly diagnosed malignancy (excluding non-melanoma skin cancer) and the fifth leading cause of cancer death in men worldwide (1,2).
In current medical practice, serum prostate-specific antigen (PSA) level, tumour Gleason score (GS) and clinical tumour stage are prognostic markers which predict clinical outcomes after radical therapy of clinically localised PC (3).
Radiotherapy (RT) is a non-surgical treatment modality which focusses megavoltage photon beams on the prostate gland with the aim of good local-regional control and prolonged disease-free survival (DFS) for PC patients (4). Normal tissue damage with RT leads to an increased cytokine production within the irradiated field, which then enters circulation (5). Cytokines are water soluble, low molecular weight proteins that transport signals between cells (6). Many previous studies reported that levels of inflammatory cytokines were increased during or after RT completion (7-10). Many pro-inflammatory cytokines can disturb microenvironment in radiated cell, thereby affecting PC progression, RT efficacy and toxicities (11).
RT is often administered in combination with androgen deprivation therapy (ADT). The use of ADT in combination with RT has become a popular treatment modality for localised high-risk PC (12-14). An understanding of the relationship between testosterone levels and inflammatory cytokine levels has been growing in recent times. Few clinical studies have evaluated the correlation between male hypogonadism and serum levels of pro- and/or anti-inflammatory cytokines (15,16). The immunological consequences of RT alone or in combination with ADT are not well explained in scientific literature. Therefore, there is clearly a need for more investigation into this area.
During this prospective study, we have measured plasma cytokine levels in PC patients treated with neoadjuvant ADT and definitive RT at various time points before and after treatment. The aim of this study is to assess the influence of ADT and RT on the expression of various circulatory cytokines in patient’s plasma.
Methods
Study population and patient information
Eighteen patients were recruited who had agreed to undergo curative external beam RT (EBRT) +/− ADT for intermediate to high-risk PC using the D’Amico classification (3). All patients were diagnosed between 2nd July 2015 and 21st April 2016 at Royal Darwin Hospital (RDH). Eligible patients were ≥18 years old, had histologically confirmed prostate adenocarcinoma, and had an ECOG (Eastern Cooperative Oncology Group) performance status of 0 to 1 and no history of prior surgery to the prostate. Exclusion criteria include prior prostate EBRT or brachytherapy, metastatic disease at presentation, prior history of malignancy (excluding non-melanoma skin cancer), and serious medical or psychiatric illness precluding safe administration of ADT or RT. Using the D’Amico classification, selected patients were classified into the following three groups: low risk, ≤ cT2a, PSA <10 ng/mL and GS ≤6; intermediate-risk, cT2b, PSA 10–20 ng/mL, GS =7; and high risk, ≥ cT2c, PSA >20 ng/mL, GS ≥9–10 (3).
This clinical study has been approved by the Human Research and Ethics Committee (HREC: 2015-2385) of the Northern Territory (NT) and the Department of Health and Menzies School of Health Research. The research team collected previous prostate tissue biopsies, blood samples at different time points during treatment. Medical and pathology records from RDH and Alan Walker Cancer Care Centre (AWCCC) were interrogated during this study.
Androgen deprivation therapy
ADT included administration of subcutaneous or intramuscular long-acting gonadotrophin-releasing hormone (GnRH) agonists or antagonists and oral anti-androgens. All participants were treated with GnRH agonists/antagonists.
Radiation therapy
All patients had fiducial markers inserted prior to RT and had daily cone beam imaging during treatment. Treatment planning was based on computed tomography (CT) performed with empty rectum, comfortably filled bladder, with patients in the prone position. Clinical target volume (CTV) included the prostate gland or the prostate gland plus seminal vesicles. A 1cm margin was added around the CTV to define the planning target volume (PTV), expect the boundary between the anterior rectal wall and the prostate, where a 0.7 cm margin was used.
Timing of patient blood sampling and plasma processing
Blood was drawn from patients before ADT, immediately preceding the initiation of RT, at the end of RT and 3 months from the completion of RT. At each sampling, 5–10 mL of peripheral blood was drawn into blindly coded 5–7 mL vacutainer tubes containing powdered lithium heparin (14 Units/mL blood). Blood samples were immediately placed on ice for transport to the laboratory, aliquoted into conical 15 mL tubes and centrifuged (3,000 × g × 20 min) to separate out the plasma. The platelet-free plasma layer was separated from the blood, transferred into coded cryotubes and frozen at –80 °C until they were analysed. The analysis was carried out under blind conditions and in accordance with the guidelines established by the Charles Darwin University committee for handling biohazardous material.
Assessment of plasma cytokines
The concentration of cytokines TNF-α, TGF-β1, IL-6 and IL-8 were measured on plasma samples using sandwich ELISA kits including human TNF-α ELISA Kit (KAC1751), human TGF-β1 ELISA kits (EHTGFBI), human IL-6 ELISA kits (KAC1261) and human IL-8 ELISA kit (KAC1301) (Life Technologies Australia Pty Ltd). Assay kits were chromogen-based and cytokine concentration (colour) was quantified using a Titertek Multiskan MCC/340 plate reader at the appropriate wavelength dictated by the particular kit utilized. Each assay was run against a standard curve with a full range predetermined for each cytokine and sample source (17). These kits were designed to detect cytokines levels using a target-specific antibody on pre-coated 96 well microplates.
Statistical analysis
Statistical analysis was performed using GraphPad Prism 7. The nonparametric Kruskal-Wallis test and Tukey’s HSD (honestly significant difference) tests were used to assess pre-treatment and post-treatment plasma cytokine concentrations. Pearson’s product moment correlation coefficients were used to determine the correlation between ADT and RT and cytokine levels in blood plasma. A P value of <0.05 was taken as significant.
Results
The clinical characteristics of patients in this study are summarised in Table 1. The mean age of PC patients at the time of diagnosis was 66.8 years (range, 53–80 years). The mean initial serum PSA (iPSA) level was 16.03 ng/mL (range, 4–71 ng/mL) with 33% of patients at <10 ng/mL (6/18), 50% of patients with PSA of 10–20 ng/mL (9/18) and 17% with PSA concentration >20 ng/mL (3/18). The mean GS was 7.88 (range, 6–10). Six percent of patients had a GS of 6 (1/18), 44% had GS 7 (8/19) disease, and 50% GS 8–10 (9/18). Five (28%) of 18 patients had intermediate-risk PC and 13 (72%) had high-risk PC. Twenty-three percent (4/18) of patients were found to have T1a-c disease, 33% (6/18) with T2a-c and 44% (8/18) had T3a-b disease. Only one patient (6%) was found to have one pelvic node involved prior to therapy. Moreover, patients were treated in combination with an oral anti-androgen. Neoadjuvant ADT commenced 3 to 6 months prior to RT. While all patients had neoadjuvant ADT, 12 patients ceased ADT after completion of RT and other 6 patients continued ADT as an adjuvant treatment up to 2 years based on their stage of cancer. Patients were planned and treated using IMRT. Definitive IMRT patients received a total dose of 78 Gy in 39 fractions (n=4) and 80 Gy in 40 fractions (n=14).
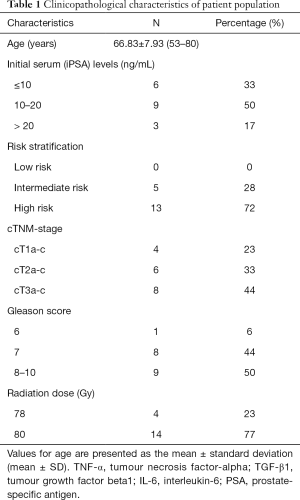
Full table
Effects of neoadjuvant ADT and RT of study cytokines levels are summarised in Figure 1. Plasma levels of TNF-α and IL-8 did not significantly alter (mean ± SD, 17.2±2.4 to 17.3±3.7 and 1.2±0.1 to 1.2±0.1, respectively) in post-ADT samples when compared to pre-ADT values. The concentration of TGF-β1 was elevated (mean ± SD, 643.7±282.7 to 765.2±426.3) and IL-6 was decreased (mean ± SD, 12.2±3.3 to 10.7±2.4) in post-ADT samples. These changes were not statistically different.
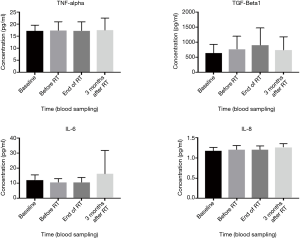
The concentration of TNF-α, IL-6 and IL-8 did not alter (mean ± SD, 17.3±3.7 to 17.2±3.7, 10.7±2.4 to 10.6±3.2 and 1.2±0.1 to 1.2±0.1 respectively) in the post-RT blood plasma when compared with pre-RT (see Figure 1). In contrast, levels of TGF-β1 increased (mean ± SD, 765.2±426.3 to 915.9±539.2) in post-RT blood plasma compared to pre-RT blood plasma. The changes in pre-RT and post-RT TGF-β1 levels were not statistically significantly different (see Table 2). The levels of IL-6, TNF-α and IL-8 did not alter, but expression levels of TGF-β1 were noticeably elevated after RT (not significantly significant).
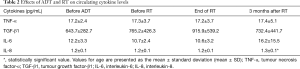
Full table
Plasma cytokine levels at completion of RT were compared with those at the 3-month follow-up period. The concentration of TNF-α did not change (mean ± SD, 17.2±3.7 to 17.4±5.1) during this time period (see Figure 1). However, TGF-β1 decreased (mean ± SD, 915.9±539.2 to 732.4±441.7) and IL-6 and IL-8 increased (mean ± SD, 10.6±3.2 to 16.2±15.5 and 1.2±0.1 to 1.3±0.1 respectively) over the same time period. Although levels of TGF-β1, IL-6 and IL-8 were found to be altered 3 months post-RT completion, only changes in IL-8 levels were found to be statistically significant (P=0.05).
Discussion
This prospective clinical study investigated the influence of neoadjuvant ADT and RT on plasma cytokine levels in patients with PC. Previous studies have reported changes in plasma cytokine levels before and after therapeutic treatments such as ADT and RT (15,16,18-22).
PC cells are androgen-dependent and ADT is a form of systemic therapy since ADT induces programmed cell death in normal, hyperplastic, pre-neoplastic, and malignant prostatic epithelial cells (23,24). Recently, there has been increasing scientific inquiry into the association between testosterone levels and inflammatory cytokines. Some previous studies have identified an association between male hypogonadism and serum levels of pro- and/or anti-inflammatory cytokines (15,16,25). Tanji et al. reported that six months of ADT significantly decreased serum fibroblast growth factor-2 (FGF-2) and vascular endothelial growth factor (VEGF) levels in serum of PC patients (15). Both FGF-2 and VEGF contribute to tumour angiogenesis in PC (26). Thus, ADT may control the concentrations of such cytokines, which suppress angiogenesis and may result in antitumor effects (27). Moreover, decreased levels of inflammatory cytokines such as IL-1, IL-6, IL-8, TNF-α, and stromal cell-derived factor 1 (SDF-1) were observed in blood samples after ADT treatment (19). One more study also identified that testosterone supplementation in hypogonadal men caused decreases in inflammatory biomarkers such as TNF-α and IL-1β (25). In this prospective study, we observed that plasma levels of TGF-β1 increased and inflammatory cytokine IL-6 decreased in post-ADT blood plasma when compared with pre-ADT plasma samples. However, these changes were not statistically different. Our findings agree with previous clinical studies that inflammatory cytokines such as IL-6 decreased after administration of ADT.
Following RT, researchers believe that gene expression changes at the messenger RNA (mRNA) level leads to increased production of some cytokines (5,17). Cytokine levels may decrease or increase depending on tumour response to RT (5,17). Several previous clinical studies reported that levels of cytokines such as interleukin (IL)-1, transforming growth factor (TGF)-β, and tumour necrosis factor (TNF)-α, Interferons- (IFN)-γ, IL-6, IL-4 and chemokine (C-C motif) ligand-2 (CCL2) were increased during or after RT completion (7-10). Further clinical studies also identified that expression levels of cytokines such as TGF-β1 and TGF-β2, IFN-γ, TNF-α and IL6 were elevated during intensity-modulated RT in the blood patients with PC (8,15,18,28). Our current study showed that expression levels of IL-6, TNF-α and IL-8 remained unchanged in post-RT plasma samples, when compared with pre-RT plasma. We did notice a trend to increasing levels of TGF-β1 post-RT, but this difference was not statistically significant.
A limited number of studies have evaluated the changes in cytokine levels after 3 months completion of therapeutic treatments. One study of head and neck cancer (HNC) reported elevated levels of serum IL-6 3 months after RT completion when compared to pre-treatment levels (29). Another study in lung cancer highlighted elevated IL-6 levels 3 months from treatment completion (17). We also have examined a time-based trend of cytokine concentrations 3 months from RT completion. Levels of TNF-α did not change after therapeutic treatments but we observed that TGF-β1 decreased, while inflammatory cytokines such as IL-6 and IL-8 increased 3 months following the administration of RT. A statically significant difference was only found between the changes in IL-8 concentration levels pre-RT and 3 months from completion of RT (P=0.05). There is a dearth of PC studies evaluating a time-based trend of cytokine concentrations 3 months from RT completion. Therefore, to evaluate the time-based trend of cytokines concentration is clearly needed in future studies and predicting the biochemical recurrence and RT-induced toxicity.
Elevated levels of the cytokines in patients with PC have been detected, suggesting that proteomic analyses of pre-treatment and post-treatment cytokine levels could be more broadly applicable for determining the response of ADT and RT. The limitations of this study included relatively small patient numbers which made interpretation of the significance of changes in cytokine levels very difficult.
Conclusions
In conclusion the changes in cytokines levels have been found after ADT and RT, which strengthen the finding of other clinical studies. Accept that small numbers of samples made difficult to attain significant results. Large clinical studies will be required to validate these findings and hopefully become useful biomarkers in the clinical setting to predict patient outcome and success of treatment received.
Acknowledgements
This clinical study was supported by College of Health and Human Sciences, Charles Darwin University, Australia. The authors would also like to acknowledge the Alan Walker Cancer Care Centre (AWCCC), RT technicians for their support in blood sampling and clinical data collection for this study, and the patients who gave their time so freely to participate in this study.
Funding: None.
Footnote
Conflicts of Interest: All authors have completed the ICMJE uniform disclosure form (available at http://dx.doi.org/10.21037/atm-19-1913). SSS serves as the unpaid editorial board member of Annals of Translational Medicine from Apr 2019 to Mar 2021. The other authors have no conflicts of interest to declare.
Ethical Statement: The author is accountable for all aspects of the work in ensuring that questions related to the accuracy or integrity of any part of the work are appropriately investigated and resolved. This clinical study has been approved by the Human Research and Ethics Committee (HREC: 2015-2385) of the Northern Territory (NT) and the Department of Health and Menzies School of Health Research.
Open Access Statement: This is an Open Access article distributed in accordance with the Creative Commons Attribution-NonCommercial-NoDerivs 4.0 International License (CC BY-NC-ND 4.0), which permits the non-commercial replication and distribution of the article with the strict proviso that no changes or edits are made and the original work is properly cited (including links to both the formal publication through the relevant DOI and the license). See: https://creativecommons.org/licenses/by-nc-nd/4.0/.
References
- Zhou CK, Check DP, Lortet-Tieulent J, et al. Prostate cancer incidence in 43 populations worldwide: an analysis of time trends overall and by age group. Int J Cancer 2016;138:1388-400. [Crossref] [PubMed]
- Wong MCS, Goggins WB, Wang HHX, et al. Global Incidence and Mortality for Prostate Cancer: Analysis of Temporal Patterns and Trends in 36 Countries. Eur Urol 2016;70:862-74. [Crossref] [PubMed]
- D'Amico AV, Whittington R, Malkowicz SB, et al. Biochemical outcome after radical prostatectomy, external beam radiation therapy, or interstitial radiation therapy for clinically localized prostate cancer. Jama 1998;280:969-74. [Crossref] [PubMed]
- Wu CT, Chen MF, Chen WC, et al. The role of IL-6 in the radiation response of prostate cancer. Radiat Oncol 2013;8:159. [Crossref] [PubMed]
- Siva S, MacManus M, Kron T, et al. A pattern of early radiation-induced inflammatory cytokine expression is associated with lung toxicity in patients with non-small cell lung cancer. PloS One 2014;9:e109560. [Crossref] [PubMed]
- George DJ, Halabi S, Shepard TF, et al. The prognostic significance of plasma interleukin-6 levels in patients with metastatic hormone-refractory prostate cancer: results from cancer and leukemia group B 9480. Clin Cancer Res 2005;11:1815-20. [Crossref] [PubMed]
- Rubin P, Johnston CJ, Williams JP, et al. A perpetual cascade of cytokines postirradiation leads to pulmonary fibrosis. Int J Radiat Oncol Biol Phys 1995;33:99-109. [Crossref] [PubMed]
- Christensen E, Pintilie M, Evans KR, et al. Longitudinal cytokine expression during IMRT for prostate cancer and acute treatment toxicity. Clin Cancer Res 2009;15:5576-83. [Crossref] [PubMed]
- Bedini N, Cicchetti A, Palorini F, et al. Evaluation of Mediators Associated with the Inflammatory Response in Prostate Cancer Patients Undergoing Radiotherapy. Dis Markers 2018;2018:9128128. [Crossref] [PubMed]
- Dirksen SR, Kirschner KF, Belyea MJ. Association of symptoms and cytokines in prostate cancer patients receiving radiation treatment. Biol Res Nurs 2014;16:250-7. [Crossref] [PubMed]
- Miao L, Holley AK, Zhao Y, et al. Redox-mediated and ionizing-radiation-induced inflammatory mediators in prostate cancer development and treatment. Antioxid Redox Signal. 2014;20:1481-500. [Crossref] [PubMed]
- DeWeese TL, Song DY. Current evidence for the role of combined androgen suppression and radiation in the treatment of adenocarcinoma of the prostate. Urology 2000;55:169-74. [Crossref] [PubMed]
- Harle LK, Maggio M, Shahani S, et al. Endocrine complications of androgen-deprivation therapy in men with prostate cancer. Clin Adv Hematol Oncol 2006;4:687-96. [PubMed]
- Basaria S, Lieb J, Tang AM, et al. Long-term effects of androgen deprivation therapy in prostate cancer patients. Clin. Endocrinol. (Oxf) 2002;56:779-86. [Crossref] [PubMed]
- Tanji N, Kikugawa T, Ochi T, et al. Circulating Cytokine Levels in Patients with Prostate Cancer: Effects of Neoadjuvant Hormonal Therapy and External-beam Radiotherapy. Anticancer Res 2015;35:3379-83. [PubMed]
- Maggio M, Blackford A, Taub D, et al. Circulating inflammatory cytokine expression in men with prostate cancer undergoing androgen deprivation therapy. J Androl 2006;27:725-8. [Crossref] [PubMed]
- Rübe CE, Palm J, Erren M, et al. Cytokine plasma levels: reliable predictors for radiation pneumonitis? PloS One. 2008;3:e2898. [Crossref] [PubMed]
- Johnke RM, Edwards JM, Evans MJ, et al. Circulating cytokine levels in prostate cancer patients undergoing radiation therapy: influence of neoadjuvant total androgen suppression. In Vivo 2009;23:827-33. [PubMed]
- Saylor PJ, Kozak KR, Smith MR, et al. Changes in biomarkers of inflammation and angiogenesis during androgen deprivation therapy for prostate cancer. Oncologist. 2012;17:212-9. [Crossref] [PubMed]
- Arpin D, Perol D, Blay J-Y, et al. Early variations of circulating interleukin-6 and interleukin-10 levels during thoracic radiotherapy are predictive for radiation pneumonitis. J Clin Oncol 2005;23:8748-56. [Crossref] [PubMed]
- Citrin DE, Hitchcock YJ, Chung EJ, et al. Determination of cytokine protein levels in oral secretions in patients undergoing radiotherapy for head and neck malignancies. Radiat Oncol 2012;7:64. [Crossref] [PubMed]
- Zhao L, Wang L, Ji W, et al. Elevation of plasma TGF-β1 during radiation therapy predicts radiation-induced lung toxicity in patients with non-small-cell lung cancer: a combined analysis from Beijing and Michigan. Int J Radiat Oncol Biol Phys 2009;74:1385-90. [Crossref] [PubMed]
- Feldman BJ, Feldman D. The development of androgen-independent prostate cancer. Nat Rev Cancer 2001;1:34-45. [Crossref] [PubMed]
- Montironi R, Mazzucchelli R, Lopez-Beltran A, et al. Mechanisms of disease: high-grade prostatic intraepithelial neoplasia and other proposed preneoplastic lesions in the prostate. Nat Clin Pract Urol 2007;4:321-32. [Crossref] [PubMed]
- Malkin CJ, Pugh PJ, Jones RD, et al. The effect of testosterone replacement on endogenous inflammatory cytokines and lipid profiles in hypogonadal men. J Clin Endocrinol Metab 2004;89:3313-8. [Crossref] [PubMed]
- Sugamoto T, Tanji N, Sato K, et al. The expression of basic fibroblast growth factor and vascular endothelial growth factor in prostatic adenocarcinoma: correlation with neovascularization. Anticancer Res 2001;21:77-88. [PubMed]
- Kaczmarek P, Pokoca L, Niemirowicz J, et al. Effect of luteinizing hormone-releasing hormone (LHRH) analogue treatment on a cytokine profile in prostate cancer patients. Pharmacol Rep 2008;60:399-403. [PubMed]
- Hurwitz MD, Kaur P, Nagaraja GM, et al. Radiation therapy induces circulating serum Hsp72 in patients with prostate cancer. Radiother Oncol 2010;95:350-8. [Crossref] [PubMed]
- Druzgal CH, Chen Z, Yeh NT, et al. A pilot study of longitudinal serum cytokine and angiogenesis factor levels as markers of therapeutic response and survival in patients with head and neck squamous cell carcinoma. Head Neck 2005;27:771-84. [Crossref] [PubMed]