Changes in the expression of interleukin-10 in myocardial infarction and its relationship with macrophage activation and cell apoptosis
Introduction
Coronary atherosclerosis, also known as coronary heart disease, is caused by myocardial ischemia, hypoxia and necrosis due to stenosis or complete occlusion of the vascular lumen secondary to the atherosclerosis of the coronary artery. Myocardial infarction (MI) is the most common type of heart disease (1,2). A widespread hypothesis among scholars is that immune and inflammatory responses are important causes for the onset of coronary heart diseases. Several types of inflammatory cells secrete and release various proinflammatory factors at the site of inflammation, and these are involved in the pathogenesis of coronary heart disease (2,3). Myocardial cells undergo apoptosis in addition to ischemia and necrosis in patients with coronary heart diseases (4,5). Currently, numerous apoptotic proteins are being studied, of which the B cell lymphoma-2 (Bcl-2) protein family, being the most classical apoptotic protein, is the most commonly investigated (5,6).
Recent studies have revealed interleukin-10 (IL-10) to be an anti-inflammatory factor that contributes prominently to the stability maintenance of coronary atherosclerotic plaque, tissue repair, etc., and also plays a protective role in the occurrence and development of coronary heart disease (7-9). Most inflammatory cells (including macrophages) can synthesize and secrete IL-10 (9). Nevertheless, it is currently unclear whether IL-10 expression is internally correlated with macrophage activation and cardiomyocyte apoptosis in coronary heart disease (including acute MI).
We present the following article in accordance with the ARRIVE reporting checklist (available at http://dx.doi.org/10.21037/atm-20-3349).
Methods
Animals and experiment design
Studies were performed with 16-to-20-week-old, male C57BL/6 mice (purchased from the Medical Experimental Center of North China University of Science and Technology) fed in a temperature (23–25 °C) and humidity (40–60%)-controlled room, and kept in a 12-h light–dark cycle with access to normal chow diet and water. All animal protocols were approved by the Institutional Review and Ethics Board of North China University of Science and Technology (LX2019035). The totle of 25 mice were divided into 5 groups in the experiment at random, which included the control group (C) (n=5), the group of mice with MI for 1 day (1 d) (n=5), the group of mice with MI for 7 days (7 d) (n=5), the group of mice with MI for 14 days (14 d) (n=5) and the group of mice with MI for 28 days (28 d) (n=5).
The mice were anesthetized by intraperitoneal injection of 1% pentobarbital sodium, followed by tracheal intubation, and thus a ventilator was connected. A 2 cm incision was made at the left sternal margin to expose the heart between the third and fourth rib. The anterior descending branch of the left coronary artery was ligated to prepare the MI model while the sham group underwent the same surgical procedure except with the exclusion of coronary artery ligation. When the color of left ventricular changed from red to white, we could judge that the animal model is successful. A total of 4 mice died (2 mice due to pneumothorax and 2 mice due to hemorrhage in the thoracic cavity) during the animal experiment, and 4 mice respectively died at 3, 11, 24, 70 hours (due to hemothorax) after the operation. The survival rate of mice models was 81%.
Material collection
After the operation, all mice in model group presented distress, ruffled fur, contracture, reduced mobility and eating. Based on these behaviors, at the specified time points, the mice were anesthetized by 1% pentobarbital sodium (50 mg/kg, i.p.) and euthanized by blood collection from the eyeball. Blood was collected and stored in a refrigerator at 4 °C for 2 h. The blood was centrifuged to collect the supernatant and then stored in a refrigerator at −80 °C for later use. Exposed chest cavity, then, the heart was extracted and the left ventricle was cut into 2 parts longitudinally. One part was fixed using 4% paraformaldehyde, while the other part was stored in a refrigerator at −80 °C.
Immunohistochemistry
The heart tissues of the mice were fixed with 4% paraformaldehyde for 24 h. The tissues were then soaked and embedded in paraffin after gradient dehydration using ethanol and de-ethanolization using xylene. The paraffin tissues were cut into 4 µm slices. Pathological changes were observed by routine hematoxylin and eosin (HE) staining, and collagen deposition was observed by Masson staining. Distributions of IL-10 and arginase in myocardial tissues were observed by immunohistochemical staining. Cell apoptosis was observed under a fluorescence microscope after terminal deoxynucleotidyl transferase dUTP nick end labeling (TUNEL) staining (Millipore, USA).
Serum IL-10 determination
The expression of IL-10 in venous blood of mice was detected by enzyme-linked immunosorbent assay (ELISA) kit. Serum samples were allowed to clot 2 hours at 4 °C before centrifugation at 3,000 ×g, 4 °C for 15 min. The supernatant was harvested immediately and stored at −80 °C for later analysis. Then, the IL-10 (E-10155); (Andy Gene Biotechnology Co., Ltd., 301 University Village Drive.Richardson. TX75081. USA) concentration in the serum were detected with the ELISA kits according to the manufacturer’s instructions. Peptide concentration was expressed in pg/mL.
Western blot
A total of 50 mg of myocardial tissues were weighed, and 1 mL of RIPA lysate containing protease inhibitor was added. After that, centrifugation was performed to extract the supernatant protein. The protein standard curve was plotted by Bradford method. The required 15 µg of protein that needed to be tested was loaded, and analysis of electrophoresis and electrolytic membrane was done then performed. Primary antibodies (IL-10, tubulin, MCP-1, arginase, iNOS, Bcl-2 and Bax; dilution performed at 1:1,000) and secondary antibodies (HRP-labeled goat anti-rabbit or goat anti-mouse IgG; dilution performed at 1:5,000) were added. Color development was conducted by electrochemiluminescence (ECL) reagent. The bands were analyzed using Image J image processing software. The ratio of optical density of the target protein to the optical density of internal (tubulin) protein was used as the relative expression of the protein.
Statistical analysis
SPSS 20.0 software was used for statistical analysis. Normality was tested for quantitative data, and the data that met normal distribution were represented by mean ± standard deviation. The mean values of multiple groups were compared using one-way analysis of variance (ANOVA), while pair-wise comparison was performed using the least significance difference (LSD) test. A P value <0.05 was considered to be statistically significant.
Results
Pathomorphological changes in mice with MI
On day 1 of MI, severe degeneration and necrosis in the in the infarct areas of the left ventricle, accompanied by inflammatory cell infiltration were observed in the mice. On day 7 of MI, there were more inflammatory cell infiltrates in addition to degenerated and necrotic cardiomyocytes, the hyperplastic collagen fibers (blue in Masson staining) were extended until the infarcted myocardial tissues, and the infarcted myocardial tissues were separated. On day 14 of MI, there were still degenerated and necrotic cardiomyocytes, accompanied by more inflammatory cell infiltrates. Moreover, collagen hyperplasia was more significant, separating the infarcted areas and the myocardial tissues in the adjacent areas into “islands”. On day 28 of MI, the inflammatory cell infiltrates had reduced when compared to their extent on day 14, and the tissues in the infarct areas were replaced by hyperplastic collagen fibers, while a small amount of residual cardiomyocytes were observed during that period (the results of Masson staining and HE staining were omitted from Figure 1).
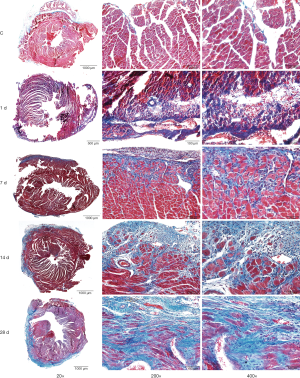
Distribution and expression of IL-10 in the heart tissues of mice
Immunohistochemical staining demonstrated the existence of a few IL-10-positive expression cells in the left ventricle in the control group. There was a small amount of cells with positive IL-10 expression in the infarcted areas on day 1 of MI, a large number of cells with positive IL-10 expression on day 7 of MI, a large number of cells with positive IL-10 expression remaining on day 14, but a reduced number of cells with positive IL-10 expression on day 28. IL-10 was mainly expressed in the inflammatory cells in the lesion areas (Figure 2).
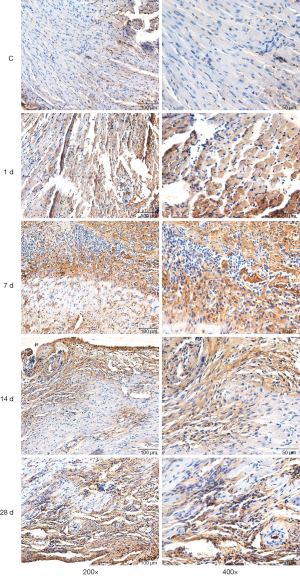
Serum IL-10 expression
The results of ELISA demonstrated that the serum IL-10 expression was significantly increased when compared to the control group on days 1, 7, and 14 of MI, which were 1.19 times, 1.22 times and 1.18 times that of the control group, respectively, with these differences being statistically significant (P<0.05). However, on day 28 of infarction, the expression was decreased to a level approaching that of the control group (Figure 3).
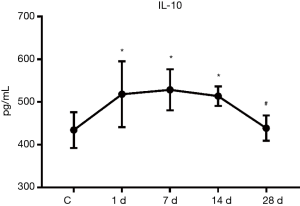
IL-10 expression in myocardial tissues
The results of western blotting revealed that the expression of IL-10 protein in myocardial tissues was increased to a certain degree when compared to the control group on day 1 of MI, and was significantly increased on day 7 of MI, and was 1.43 times that of the control group (P<0.05). Moreover, IL-10 expression peaked on day 14 of MI, and was 1.7 times that of the control group. However, on day 28 of MI, the expression of IL-10 protein was decreased significantly when compared to that on day 14, and was 74.48% of that found on day 14 (P<0.05, Figure 4).
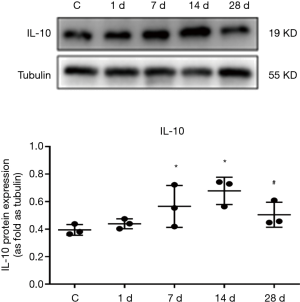
Correlation analysis of serum IL-10 and IL-10 expression in myocardial tissues showed that r was 0.543 and P was 0.005, demonstrating that the 2 were positively correlated.
Changes in the expressions of IL-10 messenger ribonucleic acid (mRNA) in mice with MI
Moreover, IL-10 mRNA expressions were detected via real time polymerase chain reaction (PCR) in mice with MI. The results of real time PCR revealed that the expression of IL-10 mRNA in myocardial tissues was increased to a certain degree when compared to the control group on day 1 of MI, and was significantly increased on day 7 of MI, Moreover, IL-10 expression peaked on day 14 of MI, However, on day 28 of MI, the expression of IL-10 protein was decreased significantly when compared to that on day 14, (P<0.05, Figure 5).The trend of IL-10 mRNA expressions were the same as the protein of the IL-10.
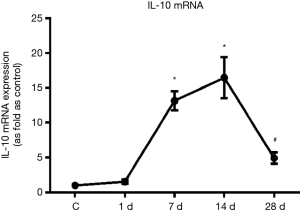
Distribution and changes of M2 macrophages in the infarcted areas of mice with MI
Immunohistochemical staining results showed that there were no cells with a significantly positive expression of arginase (i.e., M2 macrophages) distributed in the myocardial tissues in the control group. There were some cells with positive expression of arginase occasionally visible in the infarcted areas on day 1 of MI, there were some cells with positive expression of arginase on day 7, there were more cells with positive expression of arginase on day 14 when compared to day 7, and there were still many cells with positive expression of arginase on day 28 of MI (Figure 2).
Changes in the expressions of MCP-1, iNOS, and arginase in mice with MI
The results of western blotting revealed that there was an increasing trend first and then a decreasing expression of MCP-1 and iNOS proteins in the myocardial tissues. Compared to the control group, the expressions of MCP-1 protein were 1.08 times, 2.73 times, 2.29 times, and 1.39 times that of the control group on days 1, 7, 14, and 28 of MI, respectively, while the expressions of iNOS protein were 1.5 times, 4.48 times, 3.28 times, and 1.43 times higher, respectively. However, there was a gradual increasing trend in the arginase protein expression. Compared to the control group, the expression of arginase protein was 1.54 times, 2.42 times, 3.78 times, and 4.12 times that of the control group on days 1, 7, 14, and 28 of MI, respectively (Figure 6).with these differences being statistically significant (P<0.05).
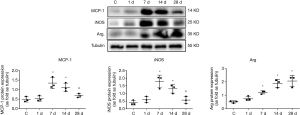
Cardiomyocyte apoptosis of mice with MI
The results of TUNEL staining showed that individual apoptotic cells were occasionally visible in the myocardial tissues in the control group. On day 1 of MI, the number of apoptotic cells in the infarcted areas was increased to some extent, and the number of apoptotic cells was increased gradually with the prolonged time of infarction (Figure 7).
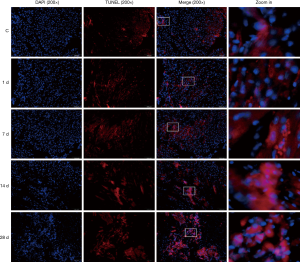
Changes in the expressions of Bax and Bcl-2 proteins in the myocardial tissues of mice with MI
Western blotting revealed the expressions of Bcl-2 and Bax proteins, along with the ratio of Bcl-2/Bax proteins, were gradually increased with prolonged time of infarction. Compared with the control group, the expressions of Bax protein on days 1, 7, 14, and 28 of MI were 1.19 times, 1.50 times, 1.70 times, and 2.23 times those of the control group, while the expressions of Bcl-2 protein were 1.44 times, 3.08 times, 4.54 times, and 6.04 times those of the control group (Figure 8). The ratios of Bcl-2/Bax were 1.22 times, 2.03 times, 2.67 times, and 2.74 times those of the control group (Figure 9) with these differences being statistically significant (P<0.05). Tubulin is an internal control protein. As fold as Tubulin means the ratio of the one protein to Tubulin
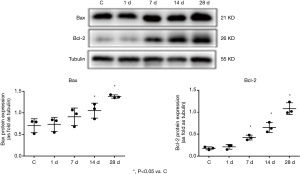
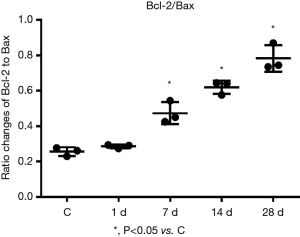
Correlation analysis of various proteins in myocardial tissues
Correlation analysis suggested a positive correlation among IL-10, Bcl-2, Bax, and arginase protein expressions (Table 1).
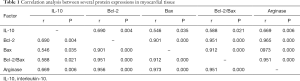
Full table
Discussion
The inflammatory responses after MI act as an important pathological process in MI. A large number of inflammatory factors with different functions are significantly involved in the inflammatory responses (2,10). IL-10 is an important anti-inflammatory factor, and plays a strong anti-inflammatory role. Studies have demonstrated that IL-10 is closely related to the occurrence and prognosis of cardiovascular diseases, and has an important regulatory effect on the remodeling of tissue functions and structures after ischemic injury (8,9,11). Recently, it has been reported that the target cells in which IL-10 plays anti-inflammatory effects are mainly macrophages. Mononuclear macrophages secrete IL-10 after activation by various endogenous and exogenous mediators, causing IL-10 genetic transcription via lipopolysaccharide activation pathway and protein kinase A-dependent activation pathway, among others (12,13). Additionally, mononuclear macrophages also secrete IL-10 during the process of clearing apoptotic cells, and this process depends on the activation of p38 mitogen-activated protein kinase (MAPK) (14). It has been reported that the expression of IL-10 is increased during ischemia, and acts as a protective response to ischemia (15,16). Some researchers have found that serum Il-10 is significantly increased on the day of acute MI. Moreover, IL-10 remains at a higher level on day 1 after percutaneous coronary intervention (PCI) therapy, but it decreases to some extent on day 7 after therapy, suggesting that IL-10 is associated with the time and severity of myocardial ischemia to some extent (17). Our study results showed that serum IL-10 expression was increased significantly on day 1 of MI in mice, and remained at a higher level on days 7 and 14 of MI, but was decreased after that, continuing to decline to a level comparable to that of the control group on day 28. There was a small number of cells with positive IL-10 expression in the infarcted areas on day 1 of MI. Moreover, the majority of the cells with positive IL-10 expression were visible on days 7 and 14 of MI, while the cells with positive IL-10 expression were decreased on day 28. However, the expression of myocardial IL-10 protein was significantly elevated on day 7 of MI, and peaked on day 14, while it decreased significantly on day 28. Correlation analysis showed that the expression of serum IL-10 was positively correlated with the expression of IL-10 protein in myocardial tissues. These results suggested an increasing trend initially and then a decrease in IL-10 expression (regardless of in serum or myocardial tissues) with the prolonged time of infarction in the mice models with MI. On day 1, MI was considered as the initial stage of acute inflammation, thus a greater necrosis of cardiomyocytes, accompanied by exudation and infiltrates of inflammatory cells, was observed. However, day 7 of MI was the critical stage of acute inflammation, in which a large amount of exudation and infiltrates of inflammatory cells, accompanied by the release of a large number of proinflammatory factors were observed. In response to these, the body releases anti-inflammatory factors (such as IL-10) to maintain a balance in the biological functions (11,18). Therefore, at around days 1 and 7 of MI, IL-10 was highly expressed in serum and/or myocardial tissues, and this continued until week 2. After that, tissue injury entered the repair stage, with inflammation also degrading gradually, and there was more extracellular matrix (including collagen) to replace the infarcted myocardial tissues gradually (3,19). At that time, synthesis and secretion of IL-10 gradually decreased, suggesting that the dynamic changes of IL-10 in serum and myocardial tissues during the process of MI were closely associated with the occurrence and development of inflammation, as well as the outcomes of MI. These might be caused by the defense and anti-inflammatory effects of the body to inflammatory responses during MI.
In inflammatory responses, macrophages are activated and phenotypic transformation occurs with the progression of inflammation. In the initial and early stages of inflammation, macrophages act as “proinflammatory” macrophages (M1 phenotype, secreting specific marker proteins such as TNF-α, iNOS, and IL-1β, etc.), which in turn promote the progression of inflammation. However, in the middle and advanced stages, macrophages act as “repairing” macrophages (M2 phenotype, secreting specific marker proteins such as arginase and CD206,), effecting anti-inflammation and tissue repair (19,20). In addition, in vitro experiment showed that fibroblasts can be activated and strengthened proliferation and migratory abilities after IL-10 intervention, and there were more collagens that were closely related to M2 phenotypic transformation of macrophages (20). In the present study, degeneration and necrosis of cardiomyocytes were observed on day 1 of MI in mice, the expression of inflammatory factor monocyte chemoattractant protein-1 (MCP-1) began to increase, and there was inflammatory cell infiltrates in the infarcted areas. Results of immunohistochemical staining showed the existence of only a small number of M2 macrophages in these inflammatory cells. Meanwhile, iNOS, which represents the marker protein of MI macrophages, was increased to some extent. On day 7 of MI, inflammatory cell infiltration in the infarcted areas was more significant. Among them, the expression of MCP-1 protein peaked, and iNOS expression achieved the highest value. However, the number of cells (M2 macrophages) with positive arginase expression and the expression of arginase protein were both increased significantly. On day 14 of MI, the iNOS expression showed declination with decreasing expression of MCP-1 protein. Until day 28, the expressions of MCP-1 and iNOS proteins declined back to levels comparable to those in the control group, while the number of cells with positive arginase expression still remained high, and the expression of arginase protein was still elevated. This suggested that the macrophages were activated and there was a phenotypic transformation from M1 macrophages in the initial stage of inflammation to M2 macrophages in the middle and advanced stages of inflammation during the process of MI. Correlation analysis showed that IL-10 expression in myocardial tissues was positively correlated with the expression of Arginase protein during the occurrence and development of MI. This indicated that the dynamic changes of IL-10 after MI might affect or regulate macrophage activation and promote the transformation of proinflammatory macrophages to repair-type macrophages.
Cardiomyocyte apoptosis also occurs with the degeneration and necrosis of cells during the process of MI. Bax and Bcl-2 are important cell apoptosis-related genes and cell apoptosis regulatory proteins. Bax plays a positive regulatory effect during cell apoptosis, promoting the occurrence of cell apoptosis. Bcl-2 plays a negative regulatory role, blocking the apoptotic pathway and inhibiting the occurrence of apoptosis (5,21). However, the ratio of Bcl-2/Bax is closely related to cell apoptosis and survival (6,22). In this study, the results showed that the number of apoptotic cells in myocardial tissues was increased gradually with prolonged time of infarction, and the expression of Bax protein was also increased gradually, indicating that cardiomyoctyte apoptosis was gradually aggravated with prolonged time of infarction. With prolonged time of infarction, the expression of Bcl-2 protein was elevated gradually, and the ratio of Bcl-2/Bax was gradually increased in particular. Correlation analysis showed that the expression of IL-10 protein in myocardial tissues was positively correlated in a significant way with the expressions of Bax and Bcl-2 proteins, especially the ratio of Bcl-2/Bax proteins. These dynamic changes suggest that IL-10 expression can affect the occurrence of cardiomyocyte apoptosis during the emergence and development of MI. Due to the gradual increase in the ratio of Bcl-2 to Bax, the effects of IL-10 might regulate cardiomyocyte apoptosis by controlling the balance between the anti-apoptotic gene (Bcl-2) and the apoptotic gene (Bax), thereby affecting remodeling of functions and structures of myocardial tissues.
In addition, further correlation analysis showed a positive correlation between IL-10 and arginase in myocardial tissues, and between the expressions of Bcl-2 and Bax proteins. This suggests that IL-10, arginase. and apoptosis-regulatory proteins show close associations and play an important role in the remodeling of tissue structures and damage repair processes after MI.
Acknowledgments
The authors would like to thank the collaborators at the School of Public Health, North China University of Science and Technology. A special thanks goes to Miss Sun Ying, head of the laboratory of Yang fang and his team who conducts IL-10 analyses.
Funding: None.
Footnote
Reporting Checklist: The authors have completed the ARRIVE reporting checklist. Available at http://dx.doi.org/10.21037/apm-20-3349
Data Sharing Statement: Available at http://dx.doi.org/10.21037/atm-20-3349
Conflicts of Interest: All authors have completed the ICMJE uniform disclosure form (available at http://dx.doi.org/10.21037/atm-20-3349). The authors have no conflicts of interest to declare.
Ethical Statement: The authors are accountable for all aspects of the work in ensuring that questions related to the accuracy or integrity of any part of the work are appropriately investigated and resolved. The study was approved by North China University of Science and Technology (2019052).
Open Access Statement: This is an Open Access article distributed in accordance with the Creative Commons Attribution-NonCommercial-NoDerivs 4.0 International License (CC BY-NC-ND 4.0), which permits the non-commercial replication and distribution of the article with the strict proviso that no changes or edits are made and the original work is properly cited (including links to both the formal publication through the relevant DOI and the license). See: https://creativecommons.org/licenses/by-nc-nd/4.0/.
References
- Yuepeng J, Zhao X, Zhao Y, et al. Gene polymorphism associated with TNF-α (G308A) IL-6 (C174G) and susceptibility to coronary atherosclerotic heart disease: A meta-analysis. Medicine (Baltimore) 2019;98:e13813. [Crossref] [PubMed]
- Kirolos I, Yakoub D, Pendola F, et al. Cardiac physiology in post myocardial infarction patients: the effect of cardiac rehabilitation programs—a systematic review and update meta-analysis. Ann Transl Med 2019;7:416. [Crossref] [PubMed]
- Prabhu SD, Frangogiannis NG. The Biological Basis for Cardiac Repair After Myocardial Infarction: From Inflammation to Fibrosis. Circ Res 2016;119:91-112. [Crossref] [PubMed]
- Zhao L, Yang XR, Han X. MicroRNA-146b induces the PI3K/Akt/NF-κB signaling pathway to reduce vascular inflammation and apoptosis in myocardial infarction by targeting PTEN. Exp Ther Med 2019;17:1171-81. [PubMed]
- Lv FH, Yin HL, He YQ, et al. Effects of curcumin on the apoptosis of cardiomyocytes and the expression of NF-κB, PPAR-γ and Bcl-2 in rats with myocardial infarction injury. Exp Ther Med 2016;12:3877-84. [Crossref] [PubMed]
- Naseri MH, Mahdavi M, Davoodi J, et al. Up regulation of Bax and down regulation of Bcl2 during 3-NC mediated apoptosis in human cancer cells. Cancer Cell Int 2015;15:55. [Crossref] [PubMed]
- Kamaly N, Fredman G, Fojas JJ, et al. Targeted interleukin-10 nanotherapeutics developed with a microfluidic chip enhance resolution of inflammation in advanced atherosclerosis. ACS Nano 2016;10:5280-92. [Crossref] [PubMed]
- Shi J, Li J, Guan H, et al. Anti-fibrotic actions of interleukin-10 against hypertrophic scarring by activation of PI3K/AKT and STAT3 signaling pathways in scar-forming fibroblasts. PLoS One 2014;9:e98228. [Crossref] [PubMed]
- Jung M, Ma YG, Iyer RP, R, et al. IL-10 improves cardiac remodeling after myocardial infarction by stimulating M2 macrophage polarization and fibroblast activation. Basic Res Cardiol 2017;112:33. [Crossref] [PubMed]
- Frangogiannis NG. The inflammatory response in myocardial injury, repair, and remodelling. Nat Rev Cardiol 2014;11:255-65. [Crossref] [PubMed]
- de Waal Malefyt R, Abrams J, Bennett B, et al. Interleukin 10(IL-10) inhibits cytokine synthesis by human monocytes: an autoregulatory role of IL-10 produced by monocytes. J Exp Med 1991;174:1209-20. [Crossref] [PubMed]
- Ernst O, Glucksam-Galnoy Y, Athamna M, et al. The cAMP pathway amplifies early myD88-dependent and type I interferon-independent LPS-induced interleukin-10 expression in mouse macrophages. Mediators Inflamm 2019;2019:3451461. [Crossref] [PubMed]
- MacKenzie KF, Clark K, Naqvi S, et al. PGE (2) induces macrophage IL-10 production and a regulatory-like phenotype via a prtein kinase A-SIK-CRTC3 pathway. J Immunol 2013;190:565-77. [Crossref] [PubMed]
- Raza A, Crothers JW, McGill MM, et al. Anti-inflammatory roles of p38α MAPK in macrophages are context dependent and require IL-10. J Leukoc Biol 2017;102:1219-27. [Crossref] [PubMed]
- Shibata M, Endo S, Inada K, et al. Elevated plasma levels of interleukin-1 receptor antagonist and interleukin-10 in patients with acute myocardial infarction. J Interferon Cytokine Res 1997;17:145-50. [Crossref] [PubMed]
- Bakhta O, Blanchard S, Guihot AL, et al. Cardioprotective role of colchicine against inflammatory injury in a rat model of acute myocardial infarction. J Cardiovasc Pharmacol Ther 2018;23:446-55. [Crossref] [PubMed]
- Wang HJ, Zhao XH. Changes and its significance of serum interleukin-6 and interleukin-10 in AMI patients after percutaneous coronary intervention. Chin J Cardiovasc Rehabil Med 2012;21:372-4.
- Peng Y, Cai X, Zhang G, et al. Molecular characterization and expression of interleukin-10 and interleukin-22 in golden pompano (Trachinotus ovatus) in response to Streptococcus agalactiae stimulus. Fish Shellfish Immunol 2017;65:244-55. [Crossref] [PubMed]
- Mills CD. M1 and M2 macrophages: oracles of health and disease. Crit Rev Immunol 2012;32:463-88. [Crossref] [PubMed]
- Gombozhapova A, Rogovskaya Y, Shurupov V, et al. Macrophage activation and polarization in post-infarction cardiac remodeling. J Biomed Sci 2017;24:13. [Crossref] [PubMed]
- Uzu M, Sato H, Shimizu A, et al. Connexin 43 enhances Bax activation via JNK activation in sunitinib-induced apoptosis in mesothelioma cells. J Pharmacol Sci 2017;134:101-7. [Crossref] [PubMed]
- Vucicevic K, Jakovljevic V, Colovic N, et al. Association of Bax expression and Bcl2/Bax ratio with clinical and molecular prognostic markers in chronic lymphocytic leukemia. J Med Biochem 2016;35:150-7. [Crossref] [PubMed]