Cigarette smoking and lung function decline beyond quitting
The tobacco epidemic
From a public health perspective, the global epidemic of tobacco smoking is one of the most prominent features of the 20th Century. In the U.S., at the time of the 1964 Surgeon General’s report on smoking and health, the rise of population cigarette consumption peaked at more than 4,000 cigarettes per year per capita (1). The subsequent decline can be attributed to a combination of multiple tobacco control measures that were sustained over time—from advertising and sponsorship bans, focused federal taxes, clean indoor air laws, nicotine replacement therapies and other anti-smoking campaigns targeting the smoking general public (1). Smoking rates for U.S. adults fell 3-fold over this 50-year period—from 42.4% in 1965 to 14.0% in 2017 (2)—such that former or ex-smokers by then now outnumbered smokers in the general communities of most high-income countries.
Despite this decline in tobacco’s popularity, smoking-related lung diseases such as chronic obstructive pulmonary disease (COPD) and lung cancer continued to appear due to a latent phase of decades (3). A recent analysis of the Global Burden of Disease Study (GBD 1992–2017) data interestingly showed the relative risk of COPD mortality continued to increase in the U.S., and although this has been attributed to the smoking peak (4), increasing urbanisation with its pollution risks almost certainly played a role. China now has the largest number of active smokers in the world with a prevalence peaking around 34.1% in 1996, though that represented 63% of men and only 3.8% of women (5), which in 2014–2015, was still 58% and 4.0% respectively (6). Nonetheless, the relative risk of COPD mortality has been reported as decreasing in China over this period (4), with this paradox attributed to public health measures that have achieved lower indoor and outdoor air pollution targets through cleaner energy sources in spite of industrialization and increased motor vehicle traffic (7). Even so, as for any country, reducing tobacco consumption would be the most effective means of preventing COPD and other smoking-related diseases. Through the direction of the World Health Organization, tobacco control measures have been at least partially introduced into many developing countries, covering about 5 billion (65% of people) worldwide (8).
The 1977 landmark study of smokers
It is now over 40 years since Fletcher and Peto described their view of the natural history of COPD (9) which formed the foundation of how health and public health professionals approach COPD prevention through promoting smoking abstinence and quitting. It describes the decline in lung function in terms of the forced expiratory volume in one second (FEV1) which has been traditionally used to follow the progression of COPD, although this is only one measure of lung function. By observing the smoking habits and FEV1 of 792 English men followed at 6-monthly intervals for 8 years, but at differing ages, the concept of the susceptible smoker and adverse FEV1 trajectories was founded. In other words, they proposed that only a subset of smokers is at risk of the lung function damage that typically progresses to severe COPD (see Figure 1). A key message was that the trajectory/slope of FEV1 decline for these susceptible smokers could “revert back to normal” if they quit the habit (9). Thus, susceptible individuals who quit smoking by mid-adult life could delay the onset of disabling breathlessness by several years, while quitting later, even around the retirement age, could still result in a modest survival benefit. A second realisation was that young adult smokers often cut down or ended their tobacco consumption due to respiratory symptoms, such that these most severely affected individuals would be then classified among the lighter or ex-smokers respectively. Despite the importance of this work (9), it has encouraged health care professionals, guideline and funding bodies to overlook the potential for ongoing lung function loss in this susceptible subgroup, who took up the additive habit when peer group pressures and the media dominated over the weak public health messages of the era.
The rationale for the present study
Oelsner and colleagues have extended this work by comprehensively undertaking a secondary analysis of the lung function data of the National Heart Lung and Blood Institute (NHLBI) Pooled Cohorts Study to more definitively establish the relationship between cigarette smoking and lung function decline in the modern era (11).
The study rationale was based on perceived inconsistencies in the literature. Firstly, in the only randomized controlled trial of smoking cessation, Anthonisen and colleagues documented an improvement in the lung function measure, FEV1, for two years after sustained quitting which then declined at a lesser rate than that of continuing smokers (12). This finding was unexpected and suggested a sizeable proportion had the so-called asthma-COPD overlap (13). Among survivors, the FEV1 then declined at 28 mL/year for sustained quitters, 48 mL/year for intermittent quitters, and 62 mL/year for continuing smokers (14), but due to the exclusion of never-smokers, these rates of decline were not compared with “normal” ageing.
Secondly, pathological evidence suggests that airway cellular and remodelling changes of COPD persist beyond smoking cessation, as distinct from any lung tissue destruction known as emphysema. Of many studies, the work by Hogg and colleagues (15) described increasing mucus and inflammatory cells across the spectrum of COPD severity, including B-cell containing lymphoid follicles that suggest microbial colonization and/or infection as a driver for those susceptible. Smoking itself is associated with increased cytotoxic CD8+ cells in the small airways which is the main site of obstruction in COPD (15,16), but combined with reduced total cell counts, reduced neutrophil and increased macrophage numbers in larger conducting airways, this may represent smoking-related inflammatory and immunosuppressive processes, as well as airway remodelling in smokers with COPD (16). However, interpreting histopathological studies is limited by their cross-sectional nature, which almost invariably cannot investigate the link between lung function changes and airway wall inflammation over time.
Thirdly, and most obviously, the work by Oelsner and colleagues seemed to have been prompted by the 2010 meta-analysis by Lee and colleagues that did not find differences in the rate of FEV1 decline between never, past smokers and recent quitters (17). But this meta-analysis was limited by including studies of cross-sectional design and those measuring spirometry prior to internationally accepted technical standards. But in identifying a knowledge gap, Lee and colleagues described an “ideal” study which would include a number of large studies that recorded: smoking habits, FEV1 levels and confounding variables at regular intervals so as to assess the lung function decline trajectory between at least two timepoints, and separately for continuing smokers and quitters using prospective and recently recorded smoking data (17).
The present study
Oelsner and colleagues have responded by performing a secondary analysis of the NHLBI Pooled Cohorts Study to document trajectories of cigarette smoking-related FEV1 decline over a median 7-year period (IQR: 3, 20) (11). This consortium comprises multiple general population-based U.S. cohorts with harmonized respiratory data that included pre-bronchodilator (but not post-bronchodilator) spirometry measured in accordance with the 2005 American Thoracic Society/European Respiratory Society standards (18). Six of these cohorts were included in the present analysis. The 25,352 eligible participants who had lung function measured at ≥2 timepoints (39% of 65,251 in total) were adults of all ages but with a median birth year in the 1930s, corresponding with the expansion phase of the smoking epidemic. Approximately two-thirds (60–68%) had a pre-bronchodilator FEV1 decline calculated from two measurements with trajectories (≥3 timepoints) developed for the remainder. Smoking categories were based on self-reported smoking status and daily intensity, namely: consistent never smokers, consistent ex-smokers, consistent current smokers and variable smokers that included those who had quit. Linear mixed models with cohort specific unstructured covariance matrices, adjusted for confounders, accommodated the repeated FEV1 measures and age variation. An additional sensitivity analysis excluded “prevalent lung disease” defined by pre-BD lung function less than lower limits of normal, self-reported doctor-diagnosed respiratory conditions and self-reported inhaler use.
While associations between spectrum of cigarette smoking exposures and FEV1 decline were reported, the paper focused on the associations for recency of smoking (decade since quit date), low smoking intensity (<5 cigarettes/day) and low cumulative consumption (1–10 pack-years). In this paragraph, the excess in lung function loss compared with never smokers has been reported, for whom the unadjusted mean FEV1 decline was 31 mL/year. A most striking feature was a “gradient of harm” for more recent decades since quitting smoking, but also a novel absolute excess of FEV1 decline even for those who had quit even over 30 years ago. Within the category of current smoking, the rate of excess FEV1 decline for those smoking ≤5 cigarettes/day was almost nine times higher than the modest estimate for former and/or current smokers with a 1–10 pack-year smoking history [7.65 mL/year (95% CI: 6.21–9.09) versus 0.87 (0.17–1.57)], implying that current smoking per se was more important. The dose-response relationship for smoking intensity was much less pronounced. The predicted FEV1 curves nicely illustrated these dose-response relationships ((figure 2, panels B and D respectively)), but interestingly, the trajectories for increasing pack-year history was almost parallel with never-smokers (Panel C), with a possible contribution from a transient improvement in recent ex-smokers (12), especially as only pre-bronchodilator spirometry was measured. When restricting the analysis to participants without co-existent lung disease, the magnitude of estimates reduced only slightly. The tables in the main document did not feature the 10.3% of participants who had quit during the period of interest, but importantly, the adjusted difference in excess FEV1 decline of 3.00 mL/year (95% CI: 2.29-3.71) fell nicely between the estimates for longer-term quitters and current smokers (panel A, table S4).
The many strengths of this study have been outlined in Table 1, while some limitations are worth mentioning. Firstly, the possibility of residual confounding by socioeconomic status (SES) beyond adjustment for educational level is not excluded. Lower SES is associated with increased smoking rates (20), some jobs with hazardous occupational exposures, and lower levels of outdoor air pollution from residing in less urbanised areas (21). While all these factors need not be adjusted for separately, ideally the models would have included another SES variable such as the zip/postcode or state of testing.
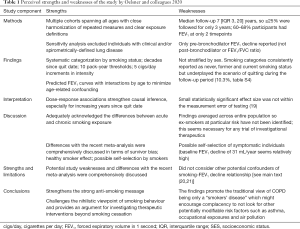
Full table
Secondly, the estimate sizes were quite small in some instances and the definition of clinical significance provided by the authors seemed rather liberal. Thus, a cut-off of 1.55 mL/year is small given the uncertainty around the measurement error of the test and the minimal clinically important difference (MCID) of ≥100 mL (19). But the dose-response nature of the findings does strengthen the biological plausibility of these causal associations.
Thirdly, a sensitivity analysis restricted to around 30–40% of eligible participants who had trajectories derived from ≥3 FEV1 measurements would have strengthened the study findings. Also, the ratio between FEV1 and forced vital capacity is more specific for airway obstruction.
The study’s importance in the field
Despite some limitations, this paper (11) provides the best evidence that “former and low-intensity current smokers have excess lung function decline compared with never smokers”. It describes an overall excess decline in a general population that spanned younger to older-aged adults, but regardless of time period or age group. It is timely because ex-smokers now commonly outnumber current smokers in many high-income countries following years of effective public health policy. The large participant numbers in part explain the narrow 95% confidence intervals, but perhaps the relatively short follow-up period for many has obscured the substantial variation in FEV1 decline expected for those with and without susceptibility to the harmful lung function effects of smoking.
Arguably, the most impressive finding was the inverse dose-response smoking-FEV1 relationship for decades “since quit date”. There was a strong signal for current cigarette smoking per se and the dose-response association for increasing smoking intensity was less pronounced with only minimal decline seen for those with a remote smoking history. These observations are consistent with the Copenhagen City Health Study (CCHS) that found a strong relationship between continued smoking and development of COPD [OR 6.3 (95% CI: 4.2–9.5)], and also a decreasing relationship with longer time periods since quitting smoking (22). Thus, a modified version of the original Fletcher and Peto diagram is presented [see Figure 1 (10)].
From longitudinal studies such as the CCHS, at least 25% of current cigarette smokers are at risk of developing the lung function feature of COPD (22). For such long-term cohorts, this estimate may be higher due to the phenomena of survivor bias and the healthy smoker effect (9). Given the median birth age for the NHLBI Pooled Cohorts Study was in the 1930s before the widespread influence of population-based public health strategies, individuals who developed symptoms may have quit smoking prior to major airway changes (9). Thus, the group quitting more than 30 years ago may represent a group of predominately susceptible smokers who stopped early due to respiratory symptomatology.
The authors make the distinction between normalization and ongoing subtle deterioration of FEV1 decline, the latter suggesting the possibility of ongoing active lung damage following smoking cessation that might be amenable to additional preventive strategies. While cross-sectional histopathological findings support the widely held view that airway inflammation and remodelling persists after smoking cessation (15,16), the present study does not identify these susceptible individuals (23). Nor does the study address other causes of impaired lung function such as the interaction of smoking with asthma resulting in asthma-COPD overlap (13,24), nor effects of air pollution and occupational hazards. It is also difficult to interpret the FEV1 values for an individual given that actual lung function varies widely with age, sex, height and ethnicity. To place the presented FEV1 values in some context, 3.8–4.1 litres (around 4,000 mL) approximates the predicted FEV1 of a 40–45 year old Caucasian male measuring 172–180 cm in height, whereas the study reports an average lung function loss for a current smoker to be 40 mL/year with an excess of 8–10 mL/year beyond ‘normal’ ageing—susceptible smokers may lose up to 60–90 mL/yr. However, for susceptible ex-smokers with a shorter and/or more remote smoking history, the excess in FEV1 decline is much less likely to manifest as clinical COPD in the absence of other major risk factors across the life-course.
Finally, the authors conclude that the lung function findings reinforce the recommendation that ‘no level of tobacco smoke exposure is safe’ and smoking cessation is key to maintaining maximal lung function. The dose-response associations indirectly confirm the dogma that ‘the time to stop smoking is now’ which is fundamental to COPD prevention in the general population. Although the decline in smoking from population-based strategies in many high-income countries has been a public health triumph, more evidence is needed to support a more targeted approach directed towards susceptible individuals in terms of active case finding and personalised interventions using modern technologies. However, for a country like China which has high and sustained smoking rates for men, an escalation of effective public health policy across the general population is more urgently needed to reduce tobacco consumption and cardiorespiratory morbidity and mortality in the future (25).
Acknowledgments
Funding: None.
Footnote
Provenance and Peer Review: This article was commissioned by the editorial office, Annals of Translational Medicine. The article did not undergo external peer review.
Conflicts of Interest: Both authors have completed the ICMJE uniform disclosure form (available at http://dx.doi.org/10.21037/atm-20-3667). JLP has received a travel grant from Boehringer-Ingelheim. The other author has no conflicts of interest to declare.
Ethical Statement: The authors are accountable for all aspects of the work in ensuring that questions related to the accuracy or integrity of any part of the work are appropriately investigated and resolved.
Open Access Statement: This is an Open Access article distributed in accordance with the Creative Commons Attribution-NonCommercial-NoDerivs 4.0 International License (CC BY-NC-ND 4.0), which permits the non-commercial replication and distribution of the article with the strict proviso that no changes or edits are made and the original work is properly cited (including links to both the formal publication through the relevant DOI and the license). See: https://creativecommons.org/licenses/by-nc-nd/4.0/.
References
- U.S. Department of Health and Human Services. The health consequences of smoking - 50 years of progress. Atlanta, GA: Department of Health and Human Services; 2014.
- Wang TW, Asman K, Gentzke AS, et al. Tobacco Product Use Among Adults - United States, 2017. MMWR Morb Mortal Wkly Rep 2018;67:1225-32. [Crossref] [PubMed]
- Poulos LM, Cooper SJ, Ampon R, et al. Mortality from asthma and COPD in Australia. Australian Institute of Health and Welfare (AIHW). Cat. no. ACM 30. Canberra: AIHW. Available online: Accessed 04-02-20; 2014.https://www.aihw.gov.au/reports/chronic-respiratory-conditions/copd/contents/deaths
- Wen H, Xie C, Wang L, et al. Difference in Long-Term Trends in COPD Mortality between China and the U.S., 1992(-)2017: An Age(-)Period(-)Cohort Analysis. Int J Environ Res Public Health 2019;16:1529. [Crossref]
- Yang G, Fan L, Tan J, et al. Smoking in China: findings of the 1996 National Prevalence Survey. JAMA 1999;282:1247-53. [Crossref] [PubMed]
- Fang L, Gao P, Bao H, et al. Chronic obstructive pulmonary disease in China: a nationwide prevalence study. Lancet Respir Med 2018;6:421-30. [Crossref] [PubMed]
- Dong GH. Ambient air pollution in China. Respirology 2019;24:626-7. [Crossref] [PubMed]
- WHO Report on the Global Tobacco Epidemic, 2019. Geneva: World Health Organization; 2019. Licence: CC BY-NC-SA 3.0 IGO.
- Fletcher C, Peto R. The natural history of chronic airflow obstruction. Br Med J 1977;1:1645-8. [Crossref] [PubMed]
- Boston University School of Public Health. Available online: Accessed 22-04-20.http://sphweb.bumc.bu.edu/otlt/MPH-Modules/PH/RespiratoryHealth/RespiratoryHealth5.html
- Oelsner EC, Balte PP, Bhatt SP, et al. Lung function decline in former smokers and low-intensity current smokers: a secondary data analysis of the NHLBI Pooled Cohorts Study. Lancet Respir Med 2020;8:34-44. [Crossref] [PubMed]
- Anthonisen NR, Connett JE, Kiley JP, et al. Effects of smoking intervention and the use of an inhaled anticholinergic bronchodilator on the rate of decline of FEV1. The Lung Health Study. JAMA 1994;272:1497-505. [Crossref] [PubMed]
- Global Initiative for Asthma. Global Initiative for Chronic Obstructive Lung Disease. Diagnosis of Diseases of Chronic Airflow Limitation. Asthma COPD and Asthma-COPD Overlap Syndrome (ACOS). 2014.
- Anthonisen NR, Connett JE, Murray RP. Smoking and lung function of Lung Health Study participants after 11 years. Am J Respir Crit Care Med 2002;166:675-9. [Crossref] [PubMed]
- Hogg JC, Chu F, Utokaparch S, et al. The nature of small-airway obstruction in chronic obstructive pulmonary disease. N Engl J Med 2004;350:2645-53. [Crossref] [PubMed]
- Eapen MS, McAlinden K, Tan D, et al. Profiling cellular and inflammatory changes in the airway wall of mild to moderate COPD. Respirology 2017;22:1125-32. [Crossref] [PubMed]
- Lee PN, Fry JS. Systematic review of the evidence relating FEV1 decline to giving up smoking. BMC Med 2010;8:84. [Crossref] [PubMed]
- Oelsner EC, Balte PP, Cassano PA, et al. Harmonization of Respiratory Data From 9 US Population-Based Cohorts: The NHLBI Pooled Cohorts Study. Am J Epidemiol 2018;187:2265-78. [Crossref] [PubMed]
- Donohue JF. Minimal clinically important differences in COPD lung function. COPD 2005;2:111-24. [Crossref] [PubMed]
- Chen Y, Chen H, Li Z. Effect of characteristics and life in cities in China on residents' smoking behaviour. J Int Med Res 2018;46:4226-34. [Crossref] [PubMed]
- Zhang S, Wei Z, Liu W, et al. Indicators for Environment Health Risk Assessment in the Jiangsu Province of China. Int J Environ Res Public Health 2015;12:11012-24. [Crossref] [PubMed]
- Lokke A, Lange P, Scharling H, et al. Developing COPD: a 25 year follow up study of the general population. Thorax 2006;61:935-9. [Crossref] [PubMed]
- Colak Y, Lange P. The long-lasting dark shadow of past and present smoking. Lancet Respir Med 2020;8:3-5. [Crossref] [PubMed]
- Perret JL, Dharmage SC, Matheson MC, et al. The Interplay between the Effects of Lifetime Asthma, Smoking, and Atopy on Fixed Airflow Obstruction in Middle Age. Am J Respir Crit Care Med 2013;187:42-8. [Crossref] [PubMed]
- Sun Y, Sin DD. Crisis of COPD in China: the future is now. Lancet Respir Med 2018;6:404-5. [Crossref] [PubMed]