Evaluation of radial artery pulse pressure effects on detection of stroke volume changes after volume loading maneuvers in cardiac surgical patients
Introduction
Appropriate fluid resuscitation is the most important hemodynamic intervention in the early postoperative period in patients after cardiac surgery (1,2). However, the risk of fluid overload has been clearly established, especially in patients with a limited cardiac reserve. For this reason, it is important to know whether the patient will respond to volume expansion (VE) before fluid administration (3,4). Several indicators and tests are currently available to identify fluid responsiveness to avoid deleterious fluid overload (2-7). The percentage change in cardiac output (CO) or stroke volume (SV) following fluid challenge (FC) has been used to discriminate responders from non-responders (8-10). However, direct CO or SV measurements are not available in most critically ill patients in routine clinical practice (11,12). Consequently, alternative hemodynamic variables such as changes in arterial pressure are still frequently used to assess FC response in clinical practice (12). Because pulse pressure (PP) is physiologically related to SV, some authors have reported using the changes in PP as a surrogate parameter of the changes in SV after a FC (12-17). However, these studies were mainly conducted in septic shock patients. Whether PP can effectively be used to discriminate fluid responsiveness compared to SV has not been well demonstrated in patients after cardiac surgery. The aim of the present study was to document the relationship between SV and radial artery pulse pressure (rPP) after passive leg raising (PLR) and FC in mechanically ventilated patients after cardiac surgery.
We present the following article in accordance with the STROBE reporting checklist (available at http://dx.doi.org/10.21037/atm-20-847).
Methods
Patients
This prospective single-center observational study was conducted in a 39-bed cardiac surgery intensive care unit (CSICU) of Zhongshan Hospital, Fudan University (Shanghai, China). Patients were enrolled in a consecutive nonrandomized manner between April 1 and July 30, 2019. Approval was obtained from the Ethics Committee of Zhongshan Hospital (No. B2016-077) and informed consent was obtained from all study patients.
All patients were routinely undergoing transesophageal echocardiography to detect or quantify cardiac disorders as well as to confirm the effect of surgery immediately after the procedure. After admittance to the CSICU and before study enrollment, patients were also routinely undergoing transthoracic echocardiography to identify different causes of hypotension, such as hypovolemia, heart failure, cardiac tamponade, and tension pneumothorax. The decision to perform VE was based on the clinical judgment of the intensivists. Indeed, the decision to FC was made individually on the basis of hemodynamic instability or signs of inadequate tissue perfusion. Hemodynamic instability was principally based on hemodynamic parameters and laboratory examinations, including at least one of the following criteria: systolic arterial pressure (SAP) <90 mmHg (or a decrease of >50 mmHg in patients previously known as hypertensive), mean arterial pressure (MAP) <65 mmHg, or the need for vasopressor infusion, urinary output of ≤0.5 mL/kg/h for at least 2 h, blood lactate of >2 mmol/L, or presence of skin mottling. Patients with active bleeding, arrhythmia, right heart dysfunction (tricuspid annular plane systolic excursion <16 mm), intracardiac shunt, and pulmonary hypertension were excluded in this study.
Management
All patients had a central venous catheter and a radial arterial catheter, which were routinely placed in the operating room. FloTrac/Vigileo system (Edwards Lifesciences, Irvine, CA, USA) was then connected to the radial arterial catheter. SV, CO, and stroke volume variation (SVV) were acquired from the FloTrac/Vigileo system. Pressure transducers were consistently adjusted to the level of the patient’s right atrium. Standard postoperative cardiac patient monitoring characteristics included continuous electrocardiography, heart rate (HR), pulse oximetry (SpO2), central venous pressure (CVP), and invasive radial arterial blood pressure. SAP, diastolic arterial pressure (DAP), and MAP were recorded from the bedside monitor connected to the FloTrac/Vigileo pressure transducer. All patients received remifentanil and propofol to achieve deep sedation and were mechanically ventilated without spontaneous breathing. The ventilator settings were adjusted according to routine practice. These criteria included mechanical ventilation mode: intermittent positive pressure ventilation, tidal volume: 6 and 8 mL/kg of ideal body weight, respiratory rate: 15 breaths per min, FiO2: 50%, plateau pressure <30 cmH2O, PaCO2 ≤45 mmHg, and SpO2 >95% (5,6). Since higher PEEP may have adverse effects, such as lung over inflation and hemodynamic deterioration, a PEEP of 5 cmH2O was set initially after cardiac surgery.
Study design
Three set of measurements, including HR, SAP, DAP, MAP, rPP, CVP, SVV, CO, and SV, were recorded at baseline, at the end of PLR, and after a FC (250 mL of Gelofusine for 10 min). The patient was placed in a semi-recumbent position (45°) at baseline. Thereafter, a standardized PLR test was performed as previously described (18,19). Briefly, the patient was moved from the semi-recumbent position to a position in which the legs were elevated to 45° and the trunk remained horizontal. This was achieved by moving the bed without touching the patient. The body posture was then returned to the baseline position. A FC was carried out after SV was stable. Infusion rates of vasopressors, inotropic agents, and sedative drugs were kept consistent during the study periods.
Changes (in %) in rPP induced by PLR (ΔrPP-PLR) and FC (ΔrPP-FC) were expressed as relative changes [(rPP after PLR minus rPP at baseline)/rPP at baseline; (rPP after FC minus rPP at baseline)/rPP at baseline]. Changes (in %) in SV induced by PLR and FC were expressed as relative changes [(SV after PLR minus SV at baseline)/SV at baseline, (SV after FC minus SV at baseline)/SV at baseline].
Statistical analysis
Results were expressed as means ± standard deviation (SD) for quantitative variables and frequencies with percentages for qualitative variables according to variable distributions. Data comparison between fluid responders and non-responders was performed using a two-sample Student’s t-test. Comparisons within groups were assessed using Student’s paired t-test. Analysis of categorical data utilized the χ2 or Fisher’s exact methods. Patients were divided into two groups according to the SV increase of >15% after FC (20). The receiver operating characteristic (ROC) curves were used to evaluate the discriminative power of ΔrPP-PLR (%), ΔrPP-FC (%), ΔSAP-PLR (%), ΔSAP-FC (%), ΔDAP-PLR (%), ΔDAP-FC (%), ΔMAP-PLR (%), ΔMAP-FC (%), and SVVbaseline (%) as indicators to assess fluid responsiveness. The comparison of areas under the ROC curves was performed as previously described by DeLong et al. (21). The best cut-off value for each indicator was calculated by maximizing the Youden index (sensitivity + specificity ‒ 1). Pearson’s correlation coefficient was calculated to evaluate the correlation between different hemodynamic parameters. Statistical significance was defined as P<0.05. Statistical analysis was performed with SPSS software (version 19.0; SPSS, Inc., Chicago, IL, USA).
A grey zone approach was used to determine a range of values for which formal conclusive information could not be obtained. Grey zones were calculated according to the Cannesson method (22).
Results
Study population
A total of 102 patients (75 males) after cardiac surgery were included during the study period. Their basal and clinical data are summarized in Table 1. All patients were sedated without spontaneous breathing or cardiac arrhythmia. No patient exhibited right heart failure at echocardiography. A total of 52% patients were fluid responders defined by an increase in the SV of ≥15% after a FC. There was no statistical difference in baseline characteristics between the two groups.
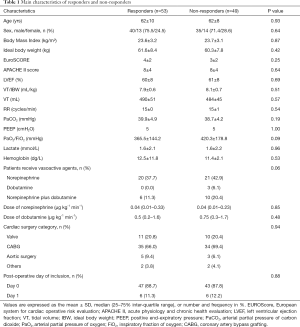
Full table
Hemodynamic changes after PLR and FC
Hemodynamic data in responders and non-responders at all study times [baseline (T0), PLR (T1), and FC (T2)] are reported in Table 2. Basal HR was not different between the responders and non-responders (73±20 vs. 69±18 beats/min, P>0.05). A significant increase was present after PLR or FC for SAP (T1 122±20 vs. 100±16 mmHg, P<0.05; T2 125±21 vs. 100±16 mmHg, P<0.05), DAP (T1 63±12 vs. 55±12 mmHg, P<0.05; T2 62±11 vs. 55±12 mmHg, P<0.05), and MAP (T1 81±15 vs. 67±15 mmHg, P<0.05; T2 82±14 vs. 67±15 mmHg, P<0.05) in responders, whereas an increase was less apparent in non-responders. The CVP was increased in both groups from baseline to PLR or FC. In responders, PLR or FC significantly increased the rPP (T1 55±15 vs. 46±12 mmHg, P<0.05; T2 63±17 vs. 46±12 mmHg, P<0.05), CO (T1 3.5±1.6 vs. 2.9±1.2 L/min, P<0.05; T2 4.0±1.8 vs. 2.9±1.2 L/min, P<0.05), and SV (T1 48.8±15.2 vs. 40.4±11.1 mL, P<0.05; T2 55.0±16.9 vs. 40.4±11.1 mL, P<0.05) and decreased the SVV (T1 8±5 vs. 15±4, P<0.05; T2 8±4 vs. 15±4, P<0.05). The changes were less notable in non-responders.
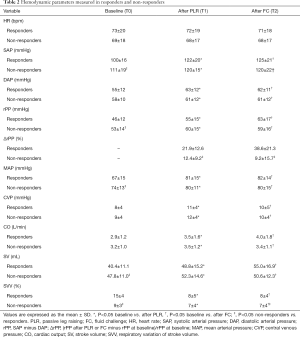
Full table
Evaluation of changes in rPP and detection of changes in SV
No statistically significant correlations were present between changes (in %) in rPP and changes (in %) in SV after PLR (ΔSV-PLR, Figure 1A, r=0.14, P=0.15). A weak relationship between changes (in %) in rPP after PLR and changes (in %) in SV after FC was also found (r=0.30, P<0.01, Figure 1B). The area under the receiver operating characteristic curve (AUROC) for the changes (in %) in rPP after PLR to predict fluid responsiveness was 0.734 (95% CI: 0.637–0.816, P<0.01). However, changes (in %) in rPP after FC had a significant positive correlation with changes (in %) in SV (r=0.62, P<0.001, Figure 1C). The AUROC for the changes (in %) in rPP after FC to detect a fluid-induced increase in SV of ≥15% was 0.881 (95% CI: 0.802–0.937, P<0.001, Figure 2).
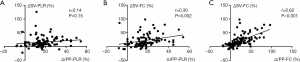
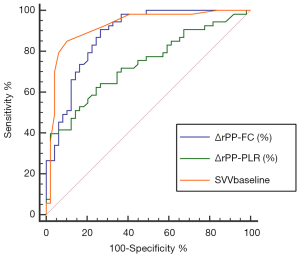
The ability of radial arterial pressure changes to detect a fluid-induced increase in SV of >15% is described in Table 3. The AUROCs for the changes (in %) in SAP, DAP, and MAP after FC to detect a fluid-induced increase in SV of ≥15% were 0.778 (95% CI: 0.685–0.854, P<0.001), 0.684 (95% CI: 0.585–0.773, P<0.001), and 0.734 (95% CI: 0.638–0.817, P<0.001). The AUROCs for the changes (in %) in SAP, DAP, and MAP after PLR to predict fluid responsiveness were 0.754 (95% CI: 0.659–0.834, P<0.001), 0.652 (95% CI: 0.551–0.744, P<0.001), and 0.704 (95% CI: 0.605–0.790, P<0.001). The best cut-off values when detecting fluid responsiveness were >17% for rPP-PLR (sensitivity of 62.26% and specificity of 75.51%) and >16% for rPP-FC (sensitivity of 90.57% and specificity of 73.47%).
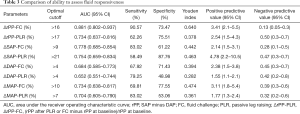
Full table
Grey zone limits
Inconclusive zone limits for SVV, ΔrPP-PLR, and ΔrPP-FC are represented in Figure 3. A large grey zone was found in ΔrPP-PLR and 43.9% of the patients were within this inconclusive zone. Conversely, small grey zones were observed in ΔrPP-FC and SVV, which included 30.39% and 4.9% of the patients, respectively (Figure 3).
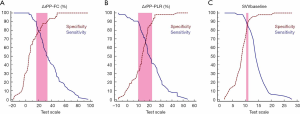
Discussion
The objective of this study was to evaluate whether the changes in rPP can track the changes in SV after PLR or FC in mechanically ventilated patients after cardiac surgery. The present study showed that the changes in rPP induced by a FC could be used to detect the changes in SV with an acceptable grey zone. In contrast, the changes in rPP induced by PLR were unreliable in predicting fluid responsiveness.
Hemodynamic lability commonly occurs in the early period post cardiac surgery (23). Evaluation of volume status is one of the most difficult issues in hemodynamically unstable patients and it is crucial to detect whether the patient is responsive to fluid. Various indicators and tests have been introduced in patients under mechanical ventilation to predict fluid responsiveness (5-7,24,25). However, the majority of these methods requires direct measurement of SV by invasive or mini-invasive monitors (11). This raises an important issue since not all patients have continuous SV monitoring in the clinical scenarios (12).
PP is an indicator that is often ignored, despite its ability to provide important information. It is well-known that the SV-to-aortic PP ratio (SV/PP) has been proposed as an estimate of total arterial compliance at rest. In theory, if the arterial compliance remains stable, changes in PP should be parallel to the changes in SV. Although PP can be affected by vascular tone, this rarely has an impact over a short period of time. PP might thus be a potential candidate for bedside monitoring of the SV changes (26).
Changes in PP in the present study are different from the respiratory pulse pressure variation (PPV) previously described in mechanically ventilated patients (27). PPV can be calculated as the difference between the maximal value of pulse pressure (PPmax) and the minimal value of pulse pressure (PPmin) over a single respiratory cycle divided by their averaged value and expressed as a percentage: PPV (%) =100 × (PPmax − PPmin)/[(PPmax + PPmin)/2]. Studies have demonstrated that PPV can reliably predict fluid responsiveness in patients under mechanical ventilation (28-30). However, this method has several limitations and can only be used in strict conditions.
At present, it is still controversial whether the changes in PP can assess fluid responsiveness in patients. Several studies have investigated the ability of PP changes to evaluate CO changes after a FC in critically ill patients (Table 4) (12,14-17,31). Garcia et al. found that overall systemic vascular resistance did not change after a FC. Therefore, there was a strong correlation between changes in PP and changes in SV after a fluid challenge (r2=0.79; P<0.0001) (32). Similar to the present findings, Monnet et al. demonstrated that the changes in PP acquired from a femoral artery and in CO after a FC were significantly correlated (r=0.56). A fluid-induced increase in PP of >17% allowed to detect a fluid-induced increase in CO of >15%, with a sensitivity of 65% and a specificity of 85%. Changes in PP did not reflect the changes in CO in patients treated with norepinephrine (r=0.21) (15). Furthermore, Dufour et al. found that there was a positive relationship between volume expansion-induced changes in SV and in PP at the femoral site (r=0.60). Increases in femoral PP of >9% indicated SV increases of >15%, with a sensitivity of 82% and a specificity of 95% (14).
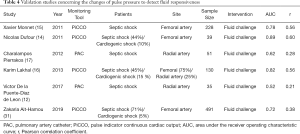
Full table
In contrast to the above findings, De la Puente-Diaz de Leon et al. reported that rPP changes cannot serve as a surrogate to assess relative CO changes after a FC in mechanically ventilated septic patients (r=0.21). The AUROC curve for the relative changes in rPP to predict fluid responsiveness was 0.52 (95% CI: 0.31–0.72, P=0.8) (12). Pierrakos et al. also showed the lack of correlation between changes in rPP and SV after a fluid challenge in patients with septic shock (r2=0.08, P=0.04). Similarly, rPP changes cannot differentiate the cardiac index in responders and non-responders (AUROC =0.618, 95% CI: 0.474–0.761, P=0.113) (17). Moreover, Ait-Hamou et al. found that fluid-induced changes in PP only roughly detected the fluid-induced changes in CO (r=0.38). The AUROC for changes in PP to detect a positive fluid response was 0.72 with a best diagnostic threshold of 10% (31).
Results of the present study are different with those reported by Pierrakos et al. and De la Puente-Diaz de Leon et al. (12,17). In these two studies, all subjects in the group were septic shock patients, and all of them were monitored by radial artery pressure. However, patients were monitored by femoral artery pressure in other studies(14-16,31,32). Patients after cardiac surgery present with a different cardiovascular physiology compared to septic patients. Their cardiac function is often impaired in different degree after surgery, while the main causes of hypotension in septic shock are diffuse vasodilation, redistribution of intravascular fluid due to increased endothelial permeability and reduced arterial tension. Recent studies revealed that vascular function in patients with septic shock may present with some abnormalities, such as peripheral vascular decoupling and decreased vascular tone (33). Vasoactive drug (norepinephrine) dosage was significantly higher in the latter study compared to the present study (0.43 vs. 0.04 µg/kg/min) (12). It may cause a dissociation between the changes in radial and femoral arteries due to the large dose of vasoactive drugs. Therefore, rPP may not track SV in septic shock patients in these studies. This can partially explain why the results of the present study do not agree with studies conducted in septic patients.
The present study data showed that a significant increase in rPP correlated with an increase in SV (responders) only after a FC in patients after cardiac surgery. It was also found that changes in ΔrPP-PLR could not predict fluid responsiveness. PLR might induce sympathetic or cardiac reflex stimulation. In addition, it might be due to the change in arterial compliance caused by mechanical stretch during PLR, which affected the relationship between SV and rPP (34). Therefore, ΔrPP-PLR may not track SV changes.
Some limitations were present in the study. First, all patients were undergoing mechanical ventilation without spontaneous respiration. It is important to note that both arrhythmias and spontaneous breathing activity will lead to misreading of the pulse pressure. Second, although the FloTrac/Vigileo system can be directly connected to the radial artery catheter, the reliability of tracking SV changes is still under debate (35). Third, only the radial artery was used to measure arterial pressure in the present study. Whether study results can be extrapolated to other monitoring sites needs to be studied further.
Conclusions
In summary, the present study demonstrated that changes in rPP can help to detect the changes in SV after a fluid challenge with a smaller grey zone in mechanically ventilated patients after cardiac surgery. If rPP increase is >16% after the fluid challenge, an SV increase of ≥15% can be expected. By contrast, the changes in rPP should not be used for monitoring the effects of PLR on SV.
Acknowledgments
Funding: This study was supported by grants from the Research Fund of Shanghai Municipal Health Commission (2019ZB0105), the Research Funds of Xiamen Branch, Zhongshan Hospital (2019ZSXMYS04), and the Research Funds of Zhongshan Hospital (2019ZSYXQN34, 2019ZSQN13, and 2018ZSQN53).
Footnote
Provenance and Peer Review: This article was commissioned by the Guest Editors (Glenn Hernández and Guo-wei Tu) for the series “Hemodynamic monitoring in critically ill patients” published in Annals of Translational Medicine. The article was sent for external peer review organized by the Guest Editors and the editorial office.
Reporting Checklist: The authors have completed the STROBE reporting checklist. Available at http://dx.doi.org/10.21037/atm-20-847
Data Sharing Statement: Available at http://dx.doi.org/10.21037/atm-20-847
Conflicts of Interest: All authors have completed the ICMJE uniform disclosure form (available at http://dx.doi.org/10.21037/atm-20-847). The series “Hemodynamic monitoring in critically ill patients” was commissioned by the editorial office without any funding or sponsorship. GWT served as the unpaid Guest Editor of the series and serves as an unpaid Section Editor of Annals of Translational Medicine from Oct 2019 to Sep 2020. The other authors have no other conflicts of interest to declare.
Ethical Statement: The authors are accountable for all aspects of the work in ensuring that questions related to the accuracy or integrity of any part of the work are appropriately investigated and resolved. The study was conducted in accordance with the Declaration of Helsinki (as revised in 2013). Approval was obtained from the Ethics Committee of Zhongshan Hospital (No. B2016-077) and informed consent was obtained from all study patients.
Open Access Statement: This is an Open Access article distributed in accordance with the Creative Commons Attribution-NonCommercial-NoDerivs 4.0 International License (CC BY-NC-ND 4.0), which permits the non-commercial replication and distribution of the article with the strict proviso that no changes or edits are made and the original work is properly cited (including links to both the formal publication through the relevant DOI and the license). See: https://creativecommons.org/licenses/by-nc-nd/4.0/.
References
- Malbrain MLNG, Van Regenmortel N, Saugel B, et al. Principles of fluid management and stewardship in septic shock: it is time to consider the four D's and the four phases of fluid therapy. Ann Intensive Care 2018;8:66. [Crossref] [PubMed]
- Su L, Pan P, Li D, et al. Central Venous Pressure (CVP) Reduction Associated With Higher Cardiac Output (CO) Favors Good Prognosis of Circulatory Shock: A Single-Center, Retrospective Cohort Study. Front Med (Lausanne) 2019;6:216. [Crossref] [PubMed]
- Zhang H, Zhang Q, Chen X, et al. Respiratory variations of inferior vena cava fail to predict fluid responsiveness in mechanically ventilated patients with isolated left ventricular dysfunction. Ann Intensive Care 2019;9:113. [Crossref] [PubMed]
- Jozwiak M, Monnet X, Teboul JL. Prediction of fluid responsiveness in ventilated patients. Ann Transl Med 2018;6:352. [Crossref] [PubMed]
- Ma GG, Hao GW, Yang XM, et al. Internal jugular vein variability predicts fluid responsiveness in cardiac surgical patients with mechanical ventilation. Ann Intensive Care 2018;8:6. [Crossref] [PubMed]
- Ma GG, Tu GW, Zheng JL, et al. Changes in Stroke Volume Variation Induced by Passive Leg Raising to Predict Fluid Responsiveness in Cardiac Surgical Patients With Protective Ventilation. J Cardiothorac Vasc Anesth 2020;34:1526-33. [Crossref] [PubMed]
- Xu LY, Tu GW, Cang J, et al. End-expiratory occlusion test predicts fluid responsiveness in cardiac surgical patients in the operating theatre. Ann Transl Med 2019;7:315. [Crossref] [PubMed]
- Carsetti A, Cecconi M, Rhodes A. Fluid bolus therapy: monitoring and predicting fluid responsiveness. Curr Opin Crit Care 2015;21:388-94. [Crossref] [PubMed]
- Teboul JL, Monnet X, Chemla D, et al. Arterial Pulse Pressure Variation with Mechanical Ventilation. Am J Respir Crit Care Med 2019;199:22-31. [Crossref] [PubMed]
- Monnet X, Teboul JL. My patient has received fluid. How to assess its efficacy and side effects? Ann Intensive Care 2018;8:54. [Crossref] [PubMed]
- Pour-Ghaz I, Manolukas T, Foray N, et al. Accuracy of non-invasive and minimally invasive hemodynamic monitoring: where do we stand? Ann Transl Med 2019;7:421. [Crossref] [PubMed]
- De la Puente-Diaz de Leon V, de Jesus Jaramillo-Rocha V, Teboul JL, et al. Changes in Radial Artery Pulse Pressure During a Fluid Challenge Cannot Assess Fluid Responsiveness in Patients With Septic Shock. J Intensive Care Med 2020;35:149-53. [Crossref] [PubMed]
- Chemla D, Hebert JL, Coirault C, et al. Total arterial compliance estimated by stroke volume-to-aortic pulse pressure ratio in humans. Am J Physiol 1998;274:H500-5. [PubMed]
- Dufour N, Chemla D, Teboul JL, et al. Changes in pulse pressure following fluid loading: a comparison between aortic root (non-invasive tonometry) and femoral artery (invasive recordings). Intensive Care Med 2011;37:942-9. [Crossref] [PubMed]
- Monnet X, Letierce A, Hamzaoui O, et al. Arterial pressure allows monitoring the changes in cardiac output induced by volume expansion but not by norepinephrine. Crit Care Med 2011;39:1394-9. [Crossref] [PubMed]
- Lakhal K, Ehrmann S, Perrotin D, et al. Fluid challenge: tracking changes in cardiac output with blood pressure monitoring (invasive or non-invasive). Intensive Care Med 2013;39:1953-62. [Crossref] [PubMed]
- Pierrakos C, Velissaris D, Scolletta S, et al. Can changes in arterial pressure be used to detect changes in cardiac index during fluid challenge in patients with septic shock? Intensive Care Med 2012;38:422-8. [Crossref] [PubMed]
- Routsi C, Stanopoulos I, Kokkoris S, et al. Weaning failure of cardiovascular origin: how to suspect, detect and treat-a review of the literature. Ann Intensive Care 2019;9:6. [Crossref] [PubMed]
- Monnet X, Teboul JL. Passive leg raising: five rules, not a drop of fluid! Crit Care 2015;19:18. [Crossref] [PubMed]
- Chaves RCF, Correa TD, Neto AS, et al. Assessment of fluid responsiveness in spontaneously breathing patients: a systematic review of literature. Ann Intensive Care 2018;8:21. [Crossref] [PubMed]
- DeLong ER, DeLong DM, Clarke-Pearson DL. Comparing the areas under two or more correlated receiver operating characteristic curves: a nonparametric approach. Biometrics 1988;44:837-45. [Crossref] [PubMed]
- Cannesson M, Le Manach Y, Hofer CK, et al. Assessing the diagnostic accuracy of pulse pressure variations for the prediction of fluid responsiveness: a "gray zone" approach. Anesthesiology 2011;115:231-41. [Crossref] [PubMed]
- St André AC, DelRossi A. Hemodynamic management of patients in the first 24 hours after cardiac surgery. Crit Care Med 2005;33:2082-93. [Crossref] [PubMed]
- Yonis H, Bitker L, Aublanc M, et al. Change in cardiac output during Trendelenburg maneuver is a reliable predictor of fluid responsiveness in patients with acute respiratory distress syndrome in the prone position under protective ventilation. Crit Care 2017;21:295. [Crossref] [PubMed]
- de Courson H, Ferrer L, Cane G, et al. Evaluation of least significant changes of pulse contour analysis-derived parameters. Ann Intensive Care 2019;9:116. [Crossref] [PubMed]
- Cecconi M, Rhodes A. Pulse pressure: more than 100 years of changes in stroke volume. Intensive Care Med 2011;37:898-900. [Crossref] [PubMed]
- de Courson H, Boyer P, Grobost R, et al. Changes in dynamic arterial elastance induced by volume expansion and vasopressor in the operating room: a prospective bicentre study. Ann Intensive Care 2019;9:117. [Crossref] [PubMed]
- Michard F, Boussat S, Chemla D, et al. Relation between respiratory changes in arterial pulse pressure and fluid responsiveness in septic patients with acute circulatory failure. Am J Respir Crit Care Med 2000;162:134-8. [Crossref] [PubMed]
- Teboul JL, Monnet X. Pulse pressure variation and ARDS. Minerva Anestesiol 2013;79:398-407. [PubMed]
- Lakhal K, Ehrmann S, Benzekri-Lefevre D, et al. Respiratory pulse pressure variation fails to predict fluid responsiveness in acute respiratory distress syndrome. Crit Care 2011;15:R85. [Crossref] [PubMed]
- Ait-Hamou Z, Teboul JL, Anguel N, et al. How to detect a positive response to a fluid bolus when cardiac output is not measured? Ann Intensive Care 2019;9:138. [Crossref] [PubMed]
- Monge García MI, Gil Cano A, Gracia Romero M. Dynamic arterial elastance to predict arterial pressure response to volume loading in preload-dependent patients. Crit Care 2011;15:R15. [Crossref] [PubMed]
- Hamzaoui O, Scheeren TWL, Teboul JL. Norepinephrine in septic shock: when and how much? Curr Opin Crit Care 2017;23:342-7. [Crossref] [PubMed]
- Lakhal K, Ehrmann S, Runge I, et al. Central venous pressure measurements improve the accuracy of leg raising-induced change in pulse pressure to predict fluid responsiveness. Intensive Care Med 2010;36:940-8. [Crossref] [PubMed]
- Suehiro K, Tanaka K, Matsuura T, et al. The Vigileo-FloTrac system: arterial waveform analysis for measuring cardiac output and predicting fluid responsiveness: a clinical review. J Cardiothorac Vasc Anesth 2014;28:1361-74. [Crossref] [PubMed]