Bronchoscopic lung volume reduction: status quo
Introduction
Pulmonary emphysema, a form of parenchyma destruction, is a common feature of chronic obstructive pulmonary disease (COPD) (1). COPD is the third leading cause of death in the United States, following heart disease and cancer. It also is the fourth leading cause of disability, imposing an enormous burden on the US healthcare system (2). It has been shown that the presence of emphysema on a CT scan is a strong independent predictor of disease morbidity and mortality (3,4).
Dyspnea, exercise limitation, impaired cardiac function, prolonged respiratory failure and poor prognosis in emphysematous COPD patients have been linked to lung hyperinflation. The pathophysiology is related to the underlying abnormal lung and airway mechanics causing excessive gas trapping in the thorax following normal expiration (static hyperinflation), with additional hyperinflation developing during exercise (dynamic hyperinflation) (5). Thus, therapies targeting hyperinflation have been the focus of most of the research work in emphysema.
Both short and long-acting bronchodilators lead to reduced lung volumes and improvement of static and dynamic hyperinflation (6), however these therapies do not address the fundamental problem of architectural and mechanical disruption and tissue damage, and their effect becomes insufficient in patients with advanced disease. Lung volume reduction surgery (LVRS) reduces hyperinflation and improves lung function by removal of emphysematous lung tissue. Previous data from the National Emphysema Treatment Trial (NETT) showed that patients with heterogeneous disease with low exercise capacity and both FEV1 and DLCO of more than 20% predicted could benefit from LVRS, demonstrating improvements in symptoms and physiology and reduced mortality. However, LVRS is also associated with significant short-term morbidity and mortality (7).
Bronchoscopic lung volume reduction (BLVR), also targeting lung hyperinflation, has gained popularity over the last decade. This is primarily due to their noninvasive technique and the recent clinical success of endobronchial valve placement (EBV) (8,9). It was first reported in a sample of 8 patients who either had refused LVRS or had advanced emphysema precluding surgery. In addition to the 30% increase in FEV1 and DLCO, early signals towards pneumothorax and worsening bronchospasm were seen (10). Challenges continue to face these procedures and the need to refine patient selection, reduce morbidity and mortality, and improve procedural performance are areas of present and future research.
Implications of hyperinflation
One of the cardinal symptoms in patients with emphysema is dyspnea, which may cause significant exercise limitation and affect patients’ ability to cope with daily life. In addition, dyspnea is an indicator of disease severity and is considered to be an important prognostic index in patients with emphysema. The underlying mechanisms of dyspnea are complex, but lung hyperinflation is a major etiology. Lung hyperinflation is defined by an abnormal increase in the amount of gas in the lungs and airways at the end of tidal expiration, establishing functional residual capacity above the upper limits of normal predicted values. While static hyperinflation develops as a result of emphysema destruction of lung parenchyma and subsequent loss of elastic recoil, dynamic hyperinflation occurs during exercise due to impaired lung and chest wall mechanics. These include high tidal-volume and high-frequency breathing during exercise in addition to expiratory flow limitation (5). Lung hyperinflation has also been linked to exacerbations in COPD, skeletal muscle dysfunction and impaired cardiopulmonary function (11,12).
Severe emphysematous destruction of the pulmonary capillary bed as well as prolonged hypercapnia and hypoxemia will increase pulmonary vascular resistance contributing to pulmonary hypertension. Impairments in diastolic filling are seen with increased intrathoracic pressure and ventricular interdependence may further exacerbate poor left ventricular function. While small studies have raised concern for harm in resecting part of the pulmonary capillary bed, a substudy of NETT has shown that lung volume reduction results in improvements of pulmonary capillary wedge pressure (likely due to decreased intrathoracic pressure). Additionally, improvements in systemic oxygenation and functional capacity correlate with enhanced biventricular function and normalization of mean pulmonary artery pressure (13). The above explains why most of the treatment strategies of COPD targets primarily lung hyperinflation, whether pharmacologically (bronchodilators and anti-inflammatory agents) or non-pharmacologically (lung volume reduction techniques, see Table 1).
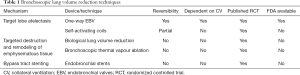
Full table
History of lung volume reduction techniques
LVRS
LVRS involves bilateral wedge resection of 20–35% of each hemithorax and can be performed either through median sternotomy or video-assisted thoracic surgery. Despite higher 90-day post procedure mortality (7.9% vs. 1.3%), mortality events rates were similar overall by the end of the 5-year follow up period (0.11 deaths per person-year in both groups) of the NETT. Subgroup analysis demonstrated that patients with biapical disease and low exercise capacity (defined as <40 W in men and <25 W in women) will have a much lower risk of death with LVRS (risk ratio 0.47) and will more likely have a clinically significant increase in exercise capacity by 10 W and 8 point decrease in Saint George’s Respiratory Questionnaire (SGRQ) at 24 months. Higher functioning, upper-lobe predominant emphysema patients still see improvements in exercise capacity and quality of life but do not achieve the mortality benefit (7). Patients with FEV1 ≤20% of predicted, a DLCO ≤20% of predicted, or homogeneous emphysema were deemed high risk and subsequently excluded from NETT due to increased perioperative mortality compared to standard of care (7). Long term follow-up of these high risk patients demonstrates improvement in dyspnea score and delayed improvement in quality of life after 4 years; however long term survival differences did not reach statistical significance and small numbers of patients may limit the robustness of this statistical analysis (14).
Bronchoscopic approaches to lung volume reduction
Currently, the most widely used devices for BLVR are: endobronchial valves, metallic coils, foam sealant, and thermal vapor ablation. As of this review publication, only endobronchial valves are FDA approved for this procedure. Pictures of each of the following techniques are shown in Figure 1.
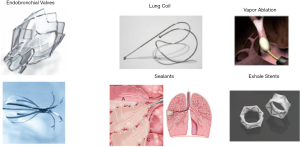
Endobronchial valves (EBV)
It took about two decades of research to define selection criteria and improve procedural technique to achieve consistently improved outcomes with EBV. EBVs include the Zephyr® Endobronchial Valve (Pulmonx, Inc., Neuchatel, Switzerland) and Spiration Valve System® (Olympus, Tokyo, Japan). They work by inducing lobar atelectasis depending on collateral ventilation (CV). They are one-way valves that allow air to escape the lung during expiration but prevent it from entering during inspiration. Tables 2 and 3 summarize the results of the valve trails for treatment of emphysema. Initial results from the VENT trial (Endobronchial Valve for Emphysema Palliation Trial) (15) in 2010 showed that treatment was substantially less effective and did not consistently reduce hyperinflation or improve lung function. That was likely due to the heterogeneity of the study and the existence of CV in the majority of patients. The study was followed by multiple trials that narrowed patients selection including STELVIO (16), BeLieVeR-HIFi (17), and TRANSFORM (18) to exclude CV+ candidates. It was not until the data of both the LIBERATE (8) and EMPROVE (9) studies that were conducted in the US, these devices became FDA approved and started to be widely used. Compared to LVRS, BLVR using valves carries less morbidity, substantially lower mortality, reversibility if needed, and lower cost and shorter hospital stays.
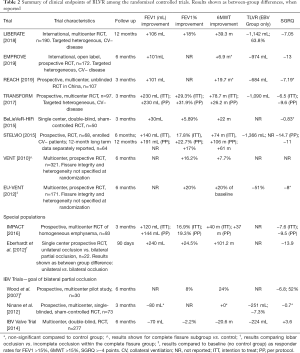
Full table
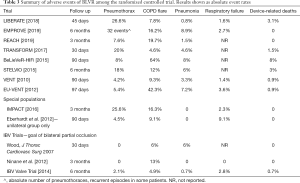
Full table
Early studies using the Intrabronchial Valve System® (IBV®) (previous version of the current Spiration Valve System®) were mostly negative or showed minimal clinical benefit but was likely related to the procedural strategy: valves were inserted in a biapical nonocclusive approach (excluding the lingula and 1 subsegment of the right upper lobe) with the goal of inducing incomplete lobar atelectasis (19-21). The study published by Eberhardt and colleagues was a direct comparison of the two approaches to EBV (unilateral occlusion vs. an incomplete bilateral approach), with unilateral occlusion providing clear superiority in achieving clinical endpoints and higher chances of developing partial or complete lobar atelectasis (22).
Self-activating coils
The PneumoRx® coil (Mountain View, California, USA) works compressing the lung parenchyma that leads to volume reduction and improvement of elastic recoil. In addition, coils reduce airflow in the targeted segments and consequently lead to redistribution of airflow towards less obstructive regions of the lung. Thus, BLVR using coils does not require absence of CV. Coils are implanted bronchoscopically, under fluoroscopy guidance, using a delivery catheter. Measurements are made to choose the appropriate coil size. Treatment is usually done sequentially with 8–14 coils implanted at a time, and each lung is treated separately. To date, there are three randomized control trials that evaluated coils as a treatment for severe emphysema. These include RESET (conducted in the UK) (23), REVOLENS (conducted in France) (24), and one multinational trial, RENEW (25). With differences in follow-up time, these trials favored coils over conventional medical therapy. The RENEW trial which randomized 315 patients (n=158 treatment group) found that patients treated with coils had a modest increase in their exercise capacity [10.3 meters increase in 6-minute walk test (6MWT)], increase in FEV1 (median change 7%) and improvement in quality of life (change in SGRQ −8.9 points), all of which were statistically significant. There were no differences in outcomes between heterogeneous and homogeneous emphysema. However, the rate of complications in the coil group was higher compared to conventional therapy. These included major complications (including hospitalization and other potentially life-threatening or fatal events, (34.8%), pneumonia (20%), and pneumothorax (9.7%). In addition, several retrospective studies evaluated the long-term efficacy of endobronchial coils and suggested a gradual waning of therapeutic benefit after one year (26,27). Retreatment was attempted in a small pilot study. Although this is safe and feasible, it did not lead to a significant change in any of the major clinical outcomes (28).
Of note, a post-hoc analysis of the RENEW trial found that patients who had the most benefit of the treatment were those with the highest emphysema scores, worse air trapping (RV ≥200% of predicted), and those with no significant airway disease on HRCT. These findings led to conducting ELEVATE Trial, which is taking into account the aforementioned results. The outcomes are still pending to date (NCT03360396) (29).
Biological lung volume reduction
AeriSeal (Aeris Therapeutics, Inc., Woburn, MA, USA) is a foam-like liquid medium used in bronchioles and alveoli to trigger an inflammatory reaction, remodeling and subsequent fibrosis to achieve volume reduction. The hydrogel product is installed bronchoscopically via a catheter into the targeted airway. In the initial report published in 2011 (30), which included 14 patients, therapy resulted in improvement in clinical outcomes including increase in FEV1 of +15.9% predicted, 6MWT of 28.7 m, and SGRQ score change of −9.9 points. However, treatment resulted in significant complications due to the induced inflammatory response. This resulted in reexamining the polymer type, dosage and techniques.
The ASPIRE study (AeriSeal System for Hyperinflation Reduction in Emphysema) published in 2015 (31), compared BLVR using foam sealant with medical therapy alone. The study was terminated for non-regulatory reasons after randomizing 95 patients. The trial resulted in high morbidity (43% requiring hospitalizations) and mortality (two deaths) in the treatment group. For these reasons, and due to insufficient data, this technique is currently not recommended.
Bronchoscopic thermal vapour ablation
Bronchoscopic Thermal Vapour Ablation (Uptake Medical Corp., Seattle) is accomplished through the installation of heated water vapor, inducing a local inflammatory reaction, destruction of emphysema and subsequent volume loss. A catheter with a balloon at its distal tip is advanced, the balloon is inflated, and the heated water vapor is delivered. The target and vapor dose depend on the density and volume of the targeted lung tissue as determined by dedicated software.
The first study was a multicenter prospective single-arm study that treated 44 patients with upper lobe-predominant emphysema. It resulted in 48% target lobar volume reduction and improvements in lung function (17% increase in FEV1), exercise tolerance (46.5±15.0 m in the 6MWT), and quality of life (14.0±2.4-point reduction in SGRQ) after 6-month follow-up. There was an increased risk of postprocedural complications related to local inflammatory response (32). In a post hoc analysis, the incidence and severity of serious adverse events correlated with the volume of the treated lobe (33). This conclusion led to designing and conducting the STEP-UP trial which used a sequential (stepwise) bilateral treatment to target individual segments based on their disease state (34). Compared to conventional treatment, vapor ablation resulted in a statistically significant increase in FEV1 (14.7%) at 6 months and a 9.7-point reduction in SGRQ. Importantly, interlobar fissure integrity did not affect outcomes in both trials. Patients in the treatment group experienced higher rates of pneumonia (18% vs. 8%) and COPD exacerbation (24% vs. 4%) compared with controls. Prophylactic antibiotics are recommended for 2 weeks after the procedure from expert opinion to avoid these complications (35).
Airway bypass stents
Exhale® Airway Bypass Procedure (Broncus Technologies, Mountain View, CA, USA) uses expandable silicone-coated, paclitaxel-eluting stents placed endobronchially into emphysematous lung tissue to enhance the emptying of trapped air and hence, achieving lung volume reduction. This is done using a needle tipped catheter creating extra-anatomic bronchial fenestrations that are maintained by the drug-eluting stents. The only randomized trial that examined this technique was the EASE trial (Exhalation Airway Stents for Emphysema) published in 2011 (36). It included patients with homogeneous emphysema, moderate to severe COPD (FEV1 ≤50% predicted) and with air-trapping RV >150%; 315 patients were randomized: 208 were treated and 107 underwent a sham procedure. Patients were followed up for 12 months. Data suggested only initial improvement (at 1-month) in lung function and dyspnea scores, but the effect was not sustained due to endurability of the stent. The loss of efficacy over time is thought to be due to factors involving mucus plugs and granuloma formation. Morbidity mostly included COPD exacerbations and infections (15.9% vs. 8.4%). For the above reasons, exhale stents are not a preferred treatment for lung volume reduction and are not widely used. Further research is needed to optimize this technique.
Patient selection
Following selection criteria of the major EBV trials, patients require severe hyperinflation from advanced emphysematous destruction as evidenced by a TLC >100% of predicted and RV >150% of predicted, along with target lobe destruction score >50% (as determined by the percentage of voxels <−910 Hounsfield units on HRCT). Preferably, heterogeneity between the emphysematous destruction of the target lobe compared to ipsilateral non-targeted lobe (INL) by 15% predicts more robust outcomes. Patients should be optimized medically through inhaler regimen, abstinence from smoking, and participation in pulmonary rehabilitation. Pre-procedure optimization cannot be stressed enough. Smoking contributes to accelerated lung function decline regardless of degree of nicotine use (37). Pharmacologic bronchodilator options are numerous and should be tailored based on Global Initiative for Chronic Obstructive Lung Disease guidelines; nebulized therapy should be considered in patients with poor inspiratory capacity (38). Furthermore nearly 17% of patients in the control arm of the LIBERATE trial still experienced a significant increase in FEV1 with optimal standard of care, benefits that were not as evident in the EMPROVE study during which pulmonary rehabilitation was not required during follow up (9). Exclusion criteria for EBV therapy include PaO2 <45 mmHg, PaCO2 >50 mmHg, FEV1 ≤15% of predicted, DLCO ≤20% of predicted, or uncontrolled pulmonary hypertension (including resting systolic pulmonary arterial pressure >45 mmHg); these patients are better served by transplant evaluation (8,9) as they are excluded from LVRS due to higher mortality post-operatively (7).
Early EBV trials, particularly VENT, were underwhelming in terms of improvements in lung function and exercise capacity but were pivotal in identifying the patient characteristics that would benefit from BLVR. Post hoc analysis identified the importance of “complete” fissure integrity [visually scored as ≥90% intact on high resolution CT (HRCT)] in achieving sustained outcomes (15). Fissure integrity is a marker of CV, which normally happens through the pores of Kohn, bronchioloalveolar communications of Lambert, and intrabronchiolar pathways of Martin (movement through these lower-resistance pathways can be enhanced in emphysema via airways obstruction), but more frank anatomic connections can be present if fissure development is incomplete (39). Therefore, bronchial occlusion will fail as a result of alternative pathways for target lobe ventilation. Because of the requirement for an intact fissure, left sided targets are more likely given the presence of only one fissure (8,9,40,41). CV assessment will be addressed further in ‘Techniques and Devices’ in discussion of use of the Chartis™ Pulmonary Assessment System (Pulmonx, Inc., Neuchatel, Switzerland).
Lobar perfusion is another important consideration when determining the optimal target. Retrospective analysis of NETT, while recapitulating the importance of upper lobe predominant emphysema in LVRS, demonstrated that the mortality benefit during follow up and the durable improvements in exercise capacity and dyspnea at 3 years were evident only in those patients with low perfusion in the upper lobes (42). Similar findings have been validated in BLVR patients, using 6MWT and SGRQ scores as clinical endpoints, with targets of <8% of total perfusion in the upper lobes and <13% of total perfusion in the lower lobe targets (43). These outcomes seem to occur independent of regional changes in target lobe perfusion after intervention, were not associated with changes in lung volumes or obstruction and necessitate lobar occlusion to achieve clinical benefits (43). Other studies of lobar perfusion have seen complementary findings such as a correlation of high INL baseline perfusion (>14%) with improvements in 6MWT (44) and correlation of FEV1 to changes in INL ventilation and perfusion post-intervention (45).
Techniques and devices
The Zephyr® EBV, formerly named the Emphasys® EBV, has a silicone membrane supported by a nickel-titanium frame and allows for collapse of the target lobe via a one-way, duckbill-shaped outlet. This design also allows mucus egress from the target lobe bronchus. It is deployed in the airways via a flexible bronchoscopy using a proprietary catheter with airway sizing markers for both airway diameter and length determination (10,15,46). Currently, the valve comes in 2 diameters, 4.0–7.0 mm and 5.5–8.5 mm. In addition, a J-shaped deployment catheter is available for the 4.0–7.0 mm valve to allow for improved articulation into the airway (Figure 2). The Spiration Valve System® is composed of a polyurethane polymer membrane supported by nitinol struts; it assumes an umbrella-shape with stabilizing anchors upon deployment in the airway. This umbrella shape allows distal airflow and mucus to be cleared along the edges during expiration (19). Airways are sized using a calibrated balloon (Figure 3) to determine which of the 4 available sizes (5, 6, 7, and 9 mm valves) should be deployed (9). Depending on the target lobe, the number of valves implanted can range from 2 to 9 valves, with most lobes on average requiring about 3–5 valves (8,15-18,40,41).
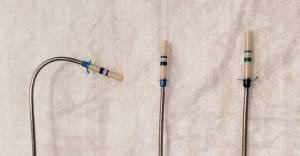
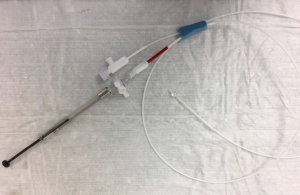
CV is assessed during the procedure using the ChartisTM Pulmonary Assessment System. This is a specialized flow catheter that measures flow and volume through the distal end; proximally, a balloon is inflated to simulate lobar occlusion of the target segment. Flow measurements will decrease and collateral resistance around the catheter increases to >10 cm H2O × mL/s if CV is negative. False positives for CV− may occur with rapid collapse of the bronchial walls around the catheter (referred to as a “low flow phenomenon”), obstruction distally on a secondary carina, or mucus plugging of the distal catheter tip; likewise false negatives for CV− can occur with incomplete sealing of the bronchus (this can be avoided by visualizing bronchial blanching around the balloon) and failure to obstruct all subsegments (a particular concern when sealing LB6) (47,48). Nonrandomized, prospective data cite a 71% positive predictive value and 83% negative predictive value for achieving lobar atelectasis or the minimally clinically important difference (MCID) in target lobe volume reduction (TLVR): this translated into 43%, 45%, and 68% of patients reaching MCID for the endpoints of FEV1, 6MWT, and SGRQ, respectively, when Chartis™ does not detect CV (48). HRCT and Chartis™ have also been validated using intraoperative fissure assessment, with 76% and 71% accuracy, respectively. HRCT showed much better sensitivity (93%) while Chartis™ was much more specific (83%) (39). Importantly, when used together, all cases of incomplete fissure integrity were detected (39), therefore the chance of inappropriately treating a patient who would derive little benefit from EBV is minimized. HRCT assessment of fissure integrity should ideally be assessed in 3 different views (axial, coronal, and sagittal).
Chartis™ assessment is not a perfect measure; however, while a small subgroup of CV+ patients still experienced clinically significant benefits (48) and readings may be inconclusive in 7–16% of patients (17,47,48). Koster and colleagues, through retrospective review, determined that fissure integrity by HRCT to have 88% PPV by visual scoring >95% and 93% NPV using a cutoff <80%; the Chartis™ system was 83% accurate in a small subset of patients. If one combines Chartis™ assessment in the indeterminate group (fissure score <95% and >80%), the accuracy approaches 90% (49). We do not treat patients at our center without definitive evidence of CV negativity and will refer these patients for LVRS evaluation.
The procedure is performed under general anesthesia to maximize device delivery and reduce the requirement for repeated procedures; nevertheless 14–35% of procedures performed in the clinical trials used conscious sedation with no signal towards poorer outcomes between the two anesthesia strategies (8,9,16). Patients are typically hospitalized after the procedure given the delayed risk of complications, namely pneumothorax. Shorter inpatient stays have been reported in several trials (16,19,40); however we mandate a 3 day inpatient stay based on the timing of pneumothorax development during the LIBERATE trial. These shorter inpatient protocols should take in the context that lower pneumothorax rates were seen in several of these trials due to a protocol that resulted in suboptimal TLVR (and therefore lower risk for pneumothorax development).
Clinical outcomes
VENT was a large multicenter, multinational randomized trial that enrolled patients in the United States and Europe. At 6 months, patients experienced statistically, but not clinically, significant improvements in FEV1 by 6.8%, a 20 m increase in 6MWT distance, and a −3.4 reduction in SGRQ scores. An indirect indication of suboptimal therapeutic response was a low pneumothorax rate seen in the VENT trial compared to subsequent studies, an event that suggests effective TLVR. While these results were disappointing, post-hoc analysis identified the importance of heterogeneity in emphysema pattern (defined as the difference in emphysema scores between the target and INL by >15%), complete lobar occlusion (defined as absence of air leakage between the bronchial wall and valve on HRCT), and “complete” fissure integrity as strong predictors of therapeutic response (15,46). The STELVIO (16,50), BeLieVeR-HIFi (17), TRANSFORM (18), and IMPACT study were subsequently designed to enroll CV− patients and showed efficacy in improving lung function and exercise capacity to varying degrees.
The pivotal trials of EBV that led to FDA-approval of EBV were the LIBERATE (8) and EMPROVE (9) trials utilizing the Zephyr® EBV and Spiration Valve System®, respectively. Both were multicenter, randomized clinical trials of patients with severe heterogenous emphysema and lack of CV. Slight differences in selection criteria should be noted. LIBERATE enrolled patients with a RV >175% of predicted, an emphysema destruction score ≥50% as assessed by voxel percentage <−910 Hounsfield units, and used the Chartis™ System to assess for CV at the time of bronchoscopy (8); EMPROVE used a less restrictive RV criteria (≥150% of predicted), an emphysema destruction score of only ≥40% with a more specific voxel cutoff <−920 Hounsfield units, and used only fissure integrity ≥90% by HRCT assessment as a marker of CV (9). MCIDs in endpoints were defined as FEV1 increases by >15%, 6MWT improvements by ≥25 m, and SGRQ reductions by >4 points; compared to the standard of care arms, responder rates were 47.7%, 41.8%, and 56.2%, respectively at 12 months (compared to 16.8%, 19.6%, and 30.2%) in LIBERATE and 36.8%, 32.4%, and 54.3%, respectively at 6 months (compared to 10%, 22.9%, and 18%) in EMPROVE. Secondary endpoints of reductions in supplemental oxygen usage and gas exchange were also reached (8,9). The REACH trial was a randomized trial of EBV conducted in China which showed improvements in FEV1 at 3- and 6-month by 104 and 91 mL, respectively, compared to marginal changes in the standard of care group. However, this study failed to show any improvement in breathlessness or exercise capacity, which may also be explained by lower TLVR on the order of 680–750 mL and baseline lower emphysema scores in the target lobe compared to LIBERATE and EMPROVE (41).
Aside from improvements in lung function, other patient factors and clinical outcomes should be mentioned. The European VENT study and the BeLieVeR-HIFi study also reported prolonged endurance times, improved workloads, as well as lower end expiratory lung volumes and higher inspiratory reserve volumes on cycle ergometry testing after BLVR (17,46). Similar FEV1 responder rates to BLVR are seen in upper and lower lobe targets (8,40,50), making it a feasible option compared to the more selective population for LVRS. Homogeneous emphysema does not necessarily preclude futility of BLVR (16,46). In fact, the IMPACT study specifically targeted patients with homogeneous emphysema and absent CV and found clinical benefits comparable to the LIBERATE and EMPROVE trials at 3 months (40). One-year follow up of participants of the STELVIO trial suggests that long term efficacy may be attenuated in patients with homogeneous emphysema, although this is based on small sample sizes (50).
Clinical outcomes reported in all of the major trials mentioned thus far are presented in Table 2. Many studies will use a MCID of ≥350 mL TLVR, as this equates to about 20% of the volume reduction in VENT and previous estimates of 300–400 mL TLVR resulting in significant FEV1 improvements (48). This cutoff was derived from the VENT trial, which had limitations in its selection criteria and subsequently lackluster results. More recent retrospective estimates of the TLVR needed to obtain clinically meaningful improvements in FEV1 and reductions in RV found that volumes of 900–1,000 mL (corresponding to percent reductions of 50–55%) are more appropriate. 6MWT distance improvements were seen at lower volumes of 600 mL but did not reach statistical significance on regression analysis; this points towards additional factors influencing cardiopulmonary function (51).
Nuclear scintigraphy and single positron emission CT (SPECT) assessments suggest that lobar shifts in ventilation and perfusion are balanced by equivalent changes in the nontreated lobes. Coxson et al. analyzed volume shifts in patients receiving the IBV® and demonstrated that 10.2% (335 mL) decrease in upper lobe volume was nearly matched by lower lobe volume increase by 11.6% (374 mL); it is noteworthy that the procedural strategy at that time was biapical atelectasis, with moderate to complete atelectasis only observed in 21% of patients (52). Pizarro et al. similarly observed ventilation and perfusion shifts that redistributed to the INL and contralateral non-concordant lobes; overall total lung volume was not significantly reduced but shifted evenly to the other lung zones (53). Lee and colleagues similarly found more prominent shifts in ventilation to the INL over the contralateral lung after 3 months using SPECT. Furthermore, they found improvements in INL gas trapping as well as improved homogeneity of ventilation/perfusion ratios, a finding that was more pronounced in the INL and in those patients with TLVR >50% of baseline (54).
Adverse events occur commonly in the early post-treatment period. Any respiratory complication can occur in 31–35% of patients (compared to 5–12% in placebo arms). However, outside of the initial post-procedure period, adverse events occur in similar proportions to patients on standard of care (21.4–33.6% vs. 10.6–30.6%). Numerically, acute exacerbations, pneumonias, and respiratory failure occur with less frequency in valve-treated patients. Of the adverse events listed, pneumothoraces occur with the highest frequency and often require tube thoracostomy for management; 26–31% of patients who develop pneumothorax may require removal of one or more EBVs. Pneumothoraces will most often occur in the INL due to rapid expansion after effective TLVR; 66–76% of pneumothoraces will occur within the first 3 days post treatment (median onset of day 1) and an additional 17–28% by 2 weeks (8,9). Exploratory analysis suggests that complex pneumothoraces will more often develop in patients in whom the most emphysematous lobe is not targeted and in those with higher emphysema destruction of the contralateral lung (8). These factors likely predict that patients who develop pneumothoraces and have a more compromised contralateral hemithorax will not be able to tolerate single lung ventilation. A summary of adverse events is provided in Table 3; differences in adverse event rates should be taken into context of individual study selection criteria.
Nonsustained lobar atelectasis should be assessed by post-procedural HRCT to arrange for revisions by repeat bronchoscopy. Adjustment procedures were required in 11 patients (5.8% of patients) in the LIBERATE trial (8) while others reported revisions necessary in about 17% of treated patients (18,41,50). The rate of expectoration is about 1% in most studies and is rarely reported to be more than 15% (8,15-18,20,41,46) so many adjustment procedures are required for ineffective TLVR and valve migration. In cases of ineffective TLVR, CT scanning may help identify malposition of valves and often be reconciled by replacing one proximal valve with two subsegmental valves.
Conclusions and future directions
BLVR is a rapidly evolving minimally-invasive procedure that can achieve the clinical benefits of LVRS without subjecting patients with advanced obstructive lung disease to substantial risks. An algorithm for current referral practice is presented in Figure 4; however ongoing and future work in BLVR should aim to improve patient outcomes by refining patient selection, procedural performance and reducing possible complications.
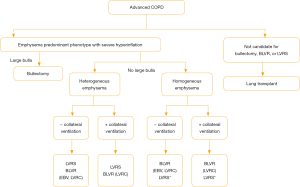
As mentioned earlier in this review, hyperinflation, measured by TLC ≥100%, was used as an inclusion criterion for patients before undergoing lung volume reduction in several trials (NETT, EMPROVE and LIBERATE). These criteria do not take into account dynamic hyperinflation, which plays a major role in activity limitation in patients with severe COPD (55). Future studies are needed to evaluate outcomes of BLVR in patients with dynamic hyperinflation.
Another important factor in patient selection is the magnitude of emphysema destruction of the target lobe on HRCT. This was assessed at different cutoffs of Hounsfield units (−950 in the NETT trial, −910 in LIBERATE and −920 in EMPROVE). In fact, one should take into account that there are variations in the optimal cut-off thresholds for individual lobes when selecting the target lobe for treatment (56). HRCT can help evaluate the pattern of emphysema destruction in the small airways, which is key in the pathology of COPD. New imaging techniques will allow functional mapping of the airways, and may help identify lung regions with the greatest loss of terminal bronchioles, luminal narrowing and obstruction in severe emphysema patients that can be potentially targeted in BLVR (57). As discussed earlier, perfusion changes have been assessed via several imaging studies, but none specifically evaluated perfusion in patient selection. This might help when two lobes are deemed to be potential targets for BLVR based on anatomic parameters and emphysema destruction. In such cases, targeting lobes with the lowest perfusion may provide better clinical outcomes by improving V/Q mismatch.
Fissure integrity and Chartis™ assessment are two other important parameters in patient selection for BLVR. While LIBERATE trial used Chartis™ assessment to enroll patients, EMPROVE trial used fissure integrity of ≥90% by HRCT. Although Chartis assessment could improve patient selection when fissure integrity between 75% and 90% (58), treatment outcomes can still be unpredictable despite using the two tests concordantly. It is important to understand fissure behavior in response to treatment to help select patients in the future.
Present and future studies should aim to address areas that help reducing morbidity mortality related to BLVR. Patients who are at high-risk, frail or those with comorbidities need to be defined, and the role of periprocedural rehabilitation may be examined. Assuring optimal periprocedural management by establishing a comprehensive multidisciplinary care and ensuring patients are on the appropriate pharmacologic and nonpharmacologic therapy can be essential in reducing morbidity and mortality. Similarly, it might be important to examine the roles of prophylactic corticosteroids and antibiotics in preventing potential complications (namely acute exacerbations and pneumonia). While it is still not quite understood why pneumothorax may occur, we hypothesize possible contributing factors. Those may include: the rate of targeted lobar collapse, tissue integrity of the ipsilateral non-treated lobe, presence of pleural adhesions, and local gas concentrations of nitrogen and oxygen and their impact on atelectasis development (59). The above are all potential areas to study that may help in predicting and preventing potential life-threatening complications of BLVR.
Acknowledgments
Funding: None.
Footnote
Provenance and Peer Review: This article was commissioned by the Guest Editor (Bassam Redwan) for the series “Lung Emphysema” published in Annals of Translational Medicine. The article was sent for external peer review organized by the Guest Editor and the editorial office.
Conflicts of Interest: All authors have completed the ICMJE uniform disclosure form (available at http://dx.doi.org/10.21037/atm-20-1551). The series “Lung Emphysema” was commissioned by the editorial office without any funding or sponsorship. The authors have no other conflicts of interest to declare.
Ethical Statement: The authors are accountable for all aspects of the work in ensuring that questions related to the accuracy or integrity of any part of the work are appropriately investigated and resolved.
Open Access Statement: This is an Open Access article distributed in accordance with the Creative Commons Attribution-NonCommercial-NoDerivs 4.0 International License (CC BY-NC-ND 4.0), which permits the non-commercial replication and distribution of the article with the strict proviso that no changes or edits are made and the original work is properly cited (including links to both the formal publication through the relevant DOI and the license). See: https://creativecommons.org/licenses/by-nc-nd/4.0/.
References
- Marsh SE, Travers J, Weatherall M, et al. Proportional classifications of COPD phenotypes. Thorax 2008;63:761-7. [Crossref] [PubMed]
- National Heart, Lung, and Blood Institute. COPD National Action Plan. Available online: https://www.nhlbi.nih.gov/health-topics/education-and-awareness/COPD-national-action-plan. 2018. Accessed February 9th, 2020.
- Haruna A, Muro S, Nakano Y, et al. CT scan findings of emphysema predict mortality in COPD. Chest 2010;138:635-40. [Crossref] [PubMed]
- Zulueta JJ, Wisnivesky JP, Henschke CI, et al. Emphysema scores predict death from COPD and lung cancer. Chest 2012;141:1216-23. [Crossref] [PubMed]
- Rossi A, Aisanov Z, Avdeev S, et al. Mechanisms, assessment and therapeutic implications of lung hyperinflation in COPD. Respir Med 2015;109:785-802. [Crossref] [PubMed]
- O'Donnell DE, Flüge T, Gerken F, et al. Effects of tiotropium on lung hyperinflation, dyspnoea and exercise tolerance in COPD. Eur Respir J 2004;23:832-40. [Crossref] [PubMed]
- Fishman A, Martinez F, Naunheim K, et al. A randomized trial comparing lung-volume-reduction surgery with medical therapy for severe emphysema. N Engl J Med 2003;348:2059-73. [Crossref] [PubMed]
- Criner GJ, Sue R, Wright S, et al. A Multicenter Randomized Controlled Trial of Zephyr Endobronchial Valve Treatment in Heterogeneous Emphysema (LIBERATE). Am J Respir Crit Care Med 2018;198:1151-64. [Crossref] [PubMed]
- Criner GJ, Delage A, Voelker K, et al. Improving Lung Function in Severe Heterogenous Emphysema With the Spiration Valve System (EMPROVE). A Multicenter, Open-Label Randomized Controlled Clinical Trial. Am J Respir Crit Care Med 2019;200:1354-62. [Crossref] [PubMed]
- Toma TP, Hopkinson NS, Hillier J, et al. Bronchoscopic volume reduction with valve implants in patients with severe emphysema. Lancet 2003;361:931-3. [Crossref] [PubMed]
- Seemungal TA, Donaldson GC, Bhowmik A, et al. Time course and recovery of exacerbations in patients with chronic obstructive pulmonary disease. Am J Respir Crit Care Med 2000;161:1608-13. [Crossref] [PubMed]
- Donaldson AV, Maddocks M, Martolini D, et al. Muscle function in COPD: a complex interplay. Int J Chron Obstruct Pulmon Dis 2012;7:523-35. [PubMed]
- Criner GJ, Scharf SM, Falk JA, et al. Effect of lung volume reduction surgery on resting pulmonary hemodynamics in severe emphysema. Am J Respir Crit Care Med 2007;176:253-60. [Crossref] [PubMed]
- Kaplan RM, Sun Q, Naunheim KS, et al. Long-term follow-up of high-risk patients in the National Emphysema Treatment Trial. Ann Thorac Surg 2014;98:1782-9. [Crossref] [PubMed]
- Sciurba FC, Ernst A, Herth FJ, et al. A randomized study of endobronchial valves for advanced emphysema. N Engl J Med 2010;363:1233-44. [Crossref] [PubMed]
- Klooster K, ten Hacken NH, Hartman JE, et al. Endobronchial Valves for Emphysema without Interlobar Collateral Ventilation. N Engl J Med 2015;373:2325-35. [Crossref] [PubMed]
- Davey C, Zoumot Z, Jordan S, et al. Bronchoscopic lung volume reduction with endobronchial valves for patients with heterogeneous emphysema and intact interlobar fissures (the BeLieVeR-HIFi study): a randomised controlled trial. Lancet 2015;386:1066-73. [Crossref] [PubMed]
- Kemp SV, Slebos DJ, Kirk A, et al. A Multicenter Randomized Controlled Trial of Zephyr Endobronchial Valve Treatment in Heterogeneous Emphysema (TRANSFORM). Am J Respir Crit Care Med 2017;196:1535-43. [Crossref] [PubMed]
- Wood DE, McKenna RJ, Yusen RD, et al. A multicenter trial of an intrabronchial valve for treatment of severe emphysema. J Thorac Cardiovasc Surg 2007;133:65-73. [Crossref] [PubMed]
- Ninane V, Geltner C, Bezzi M, et al. Multicentre European study for the treatment of advanced emphysema with bronchial valves. Eur Respir J 2012;39:1319-25. [Crossref] [PubMed]
- Wood DE, Nader DA, Springmeyer SC, et al. The IBV Valve trial: a multicenter, randomized, double-blind trial of endobronchial therapy for severe emphysema. J Bronchology Interv Pulmonol 2014;21:288-97. [Crossref] [PubMed]
- Eberhardt R, Gompelmann D, Schuhmann M, et al. Complete unilateral vs partial bilateral endoscopic lung volume reduction in patients with bilateral lung emphysema. Chest 2012;142:900-8. [Crossref] [PubMed]
- Shah PL, Zoumot Z, Singh S, et al. Endobronchial coils for the treatment of severe emphysema with hyperinflation (RESET): a randomised controlled trial. Lancet Respir Med 2013;1:233-40. [Crossref] [PubMed]
- Deslée G, Mal H, Dutau H, et al. Lung Volume Reduction Coil Treatment vs Usual Care in Patients With Severe Emphysema: The REVOLENS Randomized Clinical Trial. JAMA 2016;315:175-84. [Crossref] [PubMed]
- Sciurba FC, Criner GJ, Strange C, et al. Effect of Endobronchial Coils vs Usual Care on Exercise Tolerance in Patients With Severe Emphysema: The RENEW Randomized Clinical Trial. JAMA 2016;315:2178-89. [Crossref] [PubMed]
- Kontogianni K, Gerovasili V, Gompelmann D, et al. Coil therapy for patients with severe emphysema and bilateral incomplete fissures - effectiveness and complications after 1-year follow-up: a single-center experience. Int J Chron Obstruct Pulmon Dis 2017;12:383-94. [Crossref] [PubMed]
- Hartman JE, Klooster K, Gortzak K, et al. Long-term follow-up after bronchoscopic lung volume reduction treatment with coils in patients with severe emphysema. Respirology 2015;20:319-26. [Crossref] [PubMed]
- Hartman JE, Klooster K, Ten Hacken NHT, et al. The Safety and Feasibility of Re-treating Patients with Severe Emphysema with Endobronchial Coils: A Pilot Study. COPD 2017;14:339-43. [Crossref] [PubMed]
- Herth FJF, Slebos DJ, Shah PL, et al. Protocol of a Randomized Controlled Study of the PneumRx Endobronchial Coil System versus Standard-of-Care Medical Management in the Treatment of Subjects with Severe Emphysema (ELEVATE). Respiration 2019;98:512-20. [Crossref] [PubMed]
- Herth FJ, Eberhardt R, Ingenito EP, et al. Assessment of a novel lung sealant for performing endoscopic volume reduction therapy in patients with advanced emphysema. Expert Rev Med Devices 2011;8:307-12. [Crossref] [PubMed]
- Come CE, Kramer MR, Dransfield MT, et al. A randomised trial of lung sealant versus medical therapy for advanced emphysema. Eur Respir J 2015;46:651-62. [Crossref] [PubMed]
- Snell G, Herth FJ, Hopkins P, et al. Bronchoscopic thermal vapour ablation therapy in the management of heterogeneous emphysema. Eur Respir J 2012;39:1326-33. [Crossref] [PubMed]
- Gompelmann D, Eberhardt R, Ernst A, et al. The localized inflammatory response to bronchoscopic thermal vapor ablation. Respiration 2013;86:324-31. [Crossref] [PubMed]
- Herth FJ, Valipour A, Shah PL, et al. Segmental volume reduction using thermal vapour ablation in patients with severe emphysema: 6-month results of the multicentre, parallel-group, open-label, randomised controlled STEP-UP trial. Lancet Respir Med 2016;4:185-93. [Crossref] [PubMed]
- Gompelmann D, Shah PL, Valipour A, et al. Bronchoscopic Thermal Vapor Ablation: Best Practice Recommendations from an Expert Panel on Endoscopic Lung Volume Reduction. Respiration 2018;95:392-400. [Crossref] [PubMed]
- Shah PL, Slebos DJ, Cardoso PF, et al. Bronchoscopic lung-volume reduction with Exhale airway stents for emphysema (EASE trial): randomised, sham-controlled, multicentre trial. Lancet 2011;378:997-1005. [Crossref] [PubMed]
- Oelsner EC, Balte PP, Bhatt SP, et al. Lung function decline in former smokers and low-intensity current smokers: a secondary data analysis of the NHLBI Pooled Cohorts Study. Lancet Respir Med 2020;8:34-44. [Crossref] [PubMed]
- Harrison EM, Kim V. Long-acting maintenance pharmacotherapy in chronic obstructive pulmonary disease. Respir Med 2019;1:100009.
- Diso D, Anile M, Carillo C, et al. Correlation between collateral ventilation and interlobar lung fissures. Respiration 2014;88:315-9. [Crossref] [PubMed]
- Valipour A, Slebos DJ, Herth F, et al. Endobronchial Valve Therapy in Patients with Homogeneous Emphysema. Results from the IMPACT Study. Am J Respir Crit Care Med 2016;194:1073-82. [Crossref] [PubMed]
- Li S, Wang G, Wang C, et al. The REACH Trial: A Randomized Controlled Trial Assessing the Safety and Effectiveness of the Spiration® Valve System in the Treatment of Severe Emphysema. Respiration 2019;97:416-27. [Crossref] [PubMed]
- Chandra D, Lipson DA, Hoffman EA, et al. Perfusion scintigraphy and patient selection for lung volume reduction surgery. Am J Respir Crit Care Med 2010;182:937-46. [Crossref] [PubMed]
- Argula RG, Strange C, Ramakrishnan V, et al. Baseline regional perfusion impacts exercise response to endobronchial valve therapy in advanced pulmonary emphysema. Chest 2013;144:1578-86. [Crossref] [PubMed]
- Thomsen C, Theilig D, Herzog D, et al. Lung perfusion and emphysema distribution affect the outcome of endobronchial valve therapy. Int J Chron Obstruct Pulmon Dis 2016;11:1245-59. [PubMed]
- Kristiansen JF, Perch M, Iversen M, et al. Lobar Quantification by Ventilation/Perfusion SPECT/CT in Patients with Severe Emphysema Undergoing Lung Volume Reduction with Endobronchial Valves. Respiration 2019;98:230-8. [Crossref] [PubMed]
- Herth FJ, Noppen M, Valipour A, et al. Efficacy predictors of lung volume reduction with Zephyr valves in a European cohort. Eur Respir J 2012;39:1334-42. [Crossref] [PubMed]
- Gompelmann D, Eberhardt R, Michaud G, et al. Predicting atelectasis by assessment of collateral ventilation prior to endobronchial lung volume reduction: a feasibility study. Respiration 2010;80:419-25. [Crossref] [PubMed]
- Herth FJ, Eberhardt R, Gompelmann D, et al. Radiological and clinical outcomes of using Chartis™ to plan endobronchial valve treatment. Eur Respir J 2013;41:302-8. [Crossref] [PubMed]
- Koster TD, van Rikxoort EM, Huebner RH, et al. Predicting Lung Volume Reduction after Endobronchial Valve Therapy Is Maximized Using a Combination of Diagnostic Tools. Respiration 2016;92:150-7. [Crossref] [PubMed]
- Klooster K, Hartman JE, Ten Hacken NH, et al. One-Year Follow-Up after Endobronchial Valve Treatment in Patients with Emphysema without Collateral Ventilation Treated in the STELVIO Trial. Respiration 2017;93:112-21. [Crossref] [PubMed]
- Gompelmann D, Kontogianni K, Schuhmann M, et al. The minimal important difference for target lobe volume reduction after endoscopic valve therapy. Int J Chron Obstruct Pulmon Dis 2018;13:465-72. [Crossref] [PubMed]
- Coxson HO, Nasute Fauerbach PV, Storness-Bliss C, et al. Computed tomography assessment of lung volume changes after bronchial valve treatment. Eur Respir J 2008;32:1443-50. [Crossref] [PubMed]
- Pizarro C, Ahmadzadehfar H, Essler M, et al. Volumetric and scintigraphic changes following endoscopic lung volume reduction. Eur Respir J 2015;45:262-5. [Crossref] [PubMed]
- Lee SW, Lee SM, Shin SY, et al. Improvement in Ventilation-Perfusion Mismatch after Bronchoscopic Lung Volume Reduction: Quantitative Image Analysis. Radiology 2017;285:250-60. [Crossref] [PubMed]
- O'Donnell DE, Webb KA. The major limitation to exercise performance in COPD is dynamic hyperinflation. J Appl Physiol (1985) 2008;105:753-5; discussion 755-7. [Crossref] [PubMed]
- Wang Z, Gu S, Leader JK, et al. Optimal threshold in CT quantification of emphysema. Eur Radiol 2013;23:975-84. [Crossref] [PubMed]
- Vasilescu DM, Martinez FJ, Marchetti N, et al. Noninvasive Imaging Biomarker Identifies Small Airway Damage in Severe Chronic Obstructive Pulmonary Disease. Am J Respir Crit Care Med 2019;200:575-81. [Crossref] [PubMed]
- Fiorelli A, Santini M, Shah P. When can computed tomography-fissure analysis replace Chartis collateral ventilation assessment in the prediction of patients with emphysema who might benefit from endobronchial valve therapy? Interact Cardiovasc Thorac Surg 2018;26:313-8. [Crossref] [PubMed]
- Joyce CJ, Williams AB. Kinetics of absorption atelectasis during anesthesia: a mathematical model. J Appl Physiol (1985) 1999;86:1116-25. [Crossref] [PubMed]