Ex-vivo treatment of allografts using adipose-derived stem cells induced prolonged rejection-free survival in an allogenic hind-limb transplantation model
Introduction
Vascularized composite tissue allotransplantation (VCA) has been increasingly adopted during recent times for the reconstruction of tissues following severe injury (1). However, the side effects of the post-operative use of immunosuppressants may outweigh the benefits of VCA (2). The development of solutions that minimize the adverse effects of these immunosuppressants has been a research focus during recent years. Localized allograft immunomodulation through the ex-vivo (the period after allograft acquirement but before transplantation) treatment of allografts may provide an alternative to traditional systematic recipient immunosuppression. Ex-vivo organ perfusion has been commonly used in transplantation since the 1960s (3). However, the ex-vivo pretreatment of allografts for the induction of immunotolerance is a largely under-explored field (4). The increasing number of allografts available from non-heart beating donors has led to increased demand for improved ex-vivo organ preservation and pretreatment methods, which provides an opportunity for the development of ex-vivo allograft modification techniques.
Previous studies have shown that the application of bone marrow-derived mesenchymal stem cell (BMSC) infusion to the allografts increased the survival time of transplanted hind-limbs in a rat model of hind-limb transplantation (5), and hints at the local infusion of mesenchymal stem cell (MSCs) before transplantation, in place of the systemic delivery of MSCs, in order to reduce the potential side-effects of the systemic infusion of MSCs and the cumulative dose of immunosuppressants required.
However, the harvesting of BMSCs from the iliac crest is limited by pain and the risk of infection during the process of obtaining bone marrow (6). In contrast, MSCs can be isolated from adipose tissue through minimally invasive techniques or during liposuction to obtain adipose-derived stem cells (ADSCs). ADSCs are an immune-privileged cell type, which lacks the expression of major histocompatibility complex (MHC) class II molecules, and have been proven to inhibit mixed lymphocyte reactions in vitro through the secretion of the immunomodulatory cytokine, PGE-2 (7). Evidence of induced tolerance using ADSCs with high proliferation and immunomodulatory potential has been reported in the field of VCA (8), while there is a lack of evidence of the local perfusion of ADSCs in VCA. In our study, we tested the use of ADSCs for the ex-vivo treatment of allografts and explored their application in VCA.
The main issues addressed in this paper are the possibility of the use of ADSCs for the ex-vivo treatment of allografts, verification of its immunomodulatory effects in preventing rejection in a model of VCA and demonstration of its possible mechanisms of action. We examined the rejection-free survival of allografts through general observations, histopathological changes, as well as analysis of peripheral T cells and serum cytokines. Furthermore, we detected the expression of Tregs in allotissue, in order to explore the underlying mechanism. The results of these experiments were thereafter used to focus on immunotolerance induction in VCA and its clinical applications. We present the following article in accordance with the ARRIVE reporting checklist (available at http://dx.doi.org/10.21037/atm-19-4730).
Methods
Animals and immunosuppressive agents
Six to eight week old male Brown Norway (BN) and Lewis rats, weighing between 200 to 250 g, were purchased from Shanghai Sippr-BK Laboratory Animal Ltd. (Shanghai, People’s Republic of China) and were used as donors and recipients, respectively. All animals were housed under pathogen free conditions in standard temperature (24±2 °C) with 12 h light/dark cycle at the animal center of Shanghai Ninth People’s Hospital. Cyclosporine (Sigma Aldrich, USA) was administered postoperatively at a dose of 16 mg/kg within 5 days and then withdrawn entirely. The allografts were monitored daily for clinical signs of rejection, which were histologically confirmed. In total, sixteen animals (4 for the media control group and 6 each for the two treatment groups) were used.
ADSC isolation and expansion
Rat adipose derived mesenchymal stem cells (ADSCs) were cultured, as previously described (9). In brief, inguinal adipose tissue was obtained from 4- to 6-week-old Lewis rats (recipients). These tissues were digested using 0.01% collagenase IV (Roche Diagnostic, Mannheim, Germany) for an hour, and passed through a 40 µm filter (Falcon, Corning, USA). After centrifugation, the cell pellets were seeded into 10 cm culture dishes. The cells were cultured in complete Dulbecco’s Modified Eagle Medium with low levels of glucose and were supplemented with 10% fetal bovine serum (FBS; Sciencell, USA), 100 U/mL penicillin and 100 mg/mL streptomycin (Gibco, Invitrogen, USA) at 37 °C, under a 5% CO2 atmosphere. Cells of passages 2 to 4 were mixed and were used for further study. The cultured ADSCs were identified via the expression of surface markers CD44, CD90 and CD45 (BioLegend Inc., San Diego, USA), using a FACS Calibur flow cytometer (BD Biosciences, San Jose, CA, USA). Adipogenesis, osteogenesis and chondrogenesis were induced using a differentiation medium (Cyagen Bioscience, Inc., Guangzhou, China), and the differentiated cells were identified by staining using oil red, alizarin red and alcian blue, respectively. Differential oxygen tension was achieved by culturing the ADSCs in normoxic (21% O2) and hypoxic (5% O2) incubators (BioSpherix, Lacona, NY, USA).
Procedure of hindlimb transplantation, ex-vivo allograft engineering and general observations
All experiments were approved by the Shanghai Ninth People’s Hospital, Shanghai Jiao Tong University School of Medicine Institutional Animal Care and Use Committee {No. HKDL[2018]214}. Orthotopic hindlimb transplantation was performed from a BN donor to a Lewis receiver, under anesthesia using isoflurane. An osteomyocutaneous flap from donor BN rats were dissected and harvested, as previously described (10).
After procurement, the ex-vivo allograft vasculature was flushed through the artery using 60 units/mL of heparinized saline (Sigma Aldrich, St. Louis, MO, USA). The vasculature was then perfused using 1 million r-ADSCs (hypoxic or normoxic) in cold saline or cell-free saline alone within an hour, as previously described (5).
An oblique incision was made at the level of the inguinal ligament in the Lewis rats, the femoral vessels were dissected from the inguinal ligament for bifurcation, and the limbs were amputated at the mid-femoral level. The femoral vessels were anastomosed under an operating microscope using 12-0 nylon. Muscle and skin were stitched with 3-0 silk sutures. Lewis recipients were monitored daily for signs of rejection. Signs of epidermolysis, desquamation, exudation, eschar formation, and necrosis were considered as indications of VCA rejection.
Flow cytometry analysis of peripheral T cells
Peripheral blood from the tail vein of the recipients was collected in heparinized Eppendorf tubes. Fifty microliters of peripheral blood was stained at room temperature for 30 minutes in the dark with the following antibodies for multilineage analysis: APC-CD3, PE/Cy7-CD4, and PE-CD8a and PerCP-Cy5.5-CD45 (BD Pharmingen, San Jose, CA, USA). The blood samples were incubated with a red blood cell lysate (Beyotime, Shanghai, China) for 15 minutes and washed twice with phosphate buffered saline and analyzed using a FACS Calibur flow cytometer (BD Biosciences, San Jose, CA, USA).
Histopathology of transplanted allografts
Skin biopsy specimens were obtained from the interior aspect of the transplanted hind limb on day 7 and 14 after surgery. Additional biopsy specimens were also taken, if clinical signs of rejection were observed, in order to confirm the degree of rejection. Biopsy specimens were fixed in 4% paraformaldehyde for 24 hours, and then embedded in paraffin, cross-sectioned and stained with hematoxylin and eosin using standard procedures.
For the forkhead box p3 (Foxp3) labeling, paraffin sections were processed for heat-induced antigen retrieval in a 10 mM citrate buffer (pH 7.3), incubated with a anti rat Foxp3 (cat no. 320002; 1:200 dilution; Biolegend, San Diego, CA, USA) and visualized using a chromogenic substrate solution (Beyotime Institute of Biotechnology, Haimen, China). Finally, the slides were counter stained using hematoxylin. The percentage of Foxp3-stained cells was determined using a Nikon microscope (Ni-U, Nikon Corporation, Tokyo, Japan).
All slides were examined by 2 observers based on previously published criteria and Banff 2007 classification rejection grades (11,12), which are as follows: grade 0, no or rare inflammatory infiltrates; grade I, mild perivascular infiltration, no involvement of the overlying epidermis; grade II, moderate to severe perivascular inflammation with or without mild epidermal and or adnexal involvement (limited to spongiosis and exocytosis), no epidermal dyskeratosis or apoptosis; grade III, epidermal involvement with epithelial apoptosis, dyskeratosis, and/or keratinolysis; and grade IV, necrotizing acute rejection, frank necrosis of epidermis or other skin structures.
Measurement of cytokine levels in peripheral blood
Serum samples obtained from the peripheral blood of recipients was stored at −80 °C, until the assays were performed. Serum transforming growth factor-β (TGF-β), interleukin-10 (IL-10) and tumor necrosis factor (TNF)-a concentrations were measured using enzyme-linked immunosorbent assay kits, by following the manufacturer’s instructions (R&D Systems, Minneapolis, MN, USA).
Statistical analysis
Data are presented as mean ± SD, unless otherwise indicated. Data analysis was performed using GraphPad Prism software (La Jolla, CA). One-way ANOVA was used to evaluate differences between groups and post hoc analysis was performed using Tukey’s Multiple Comparison test. The survival rates were analyzed using the log-rank test. Further, a P value of <0.05 was considered to be statistically significant.
Results
Survival rate: ex-vivo infusion of ADSCs prolongs the survival of allografts
ADSCs were isolated from Lewis inguinal adipose tissue and expanded using previously established protocols (13). The cells were characterized through the detection of mesenchymal stem cell surface markers, CD90 and CD44, and the negative marker, CD45. The multipotency of the ADSCs was determined using adipogenic and osteogenic differentiation assays (Figure S1).
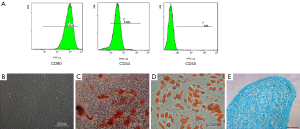
After surgery, the recipient Lewis rats received treatment, with or without the ex-vivo perfusion of ADSCs. Our results demonstrated that ex-vivo perfusion of ADSCs, either under hypoxic or normoxic conditions, could prolong the survival rate of the allografts (Figure 1). The allografts in the medium control group presented with obvious signs of grade III rejection (14) within 16 days. In the ADSC-treatment group, the mean survival time of the allografts was 26 days. Likewise, the mean survival time of the hypoxic-ADSC treated group was 32 days, indicating the temporary immunomodulatory effect of ex-vivo treatment using ADSCs. Animal models that suffered from surgical failure were excluded from the statistical analysis, which included one case of venous thrombosis in the saline control group and two cases in the ADSC perfused group.
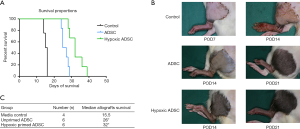
ADSCs alleviated the rejection response of allotransplants at an early stage
The animal models were monitored daily for signs of acute rejection and histological changes were assessed via hematoxylin and eosin-staining at day 7 and 14 post-transplantation. As shown in Figure 2, no significant differences were found 7 days post-transplantation, between the control and ADSC treated groups, while signs of grade III rejection, such as histological desquamation, dense inflammation, epidermolysis and gross exudation and necrosis were obvious 14 days post-transplantation in control group, but scarcely observed in the ADSC-treated groups that 2 specimens showed grade I rejection in ADSC treated group and the rest showed grade 0 rejection at 14 days post-transplantation.
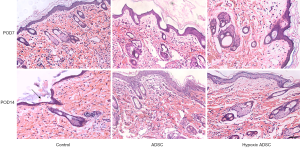
ADSCs increase the expression of anti-inflammatory cytokines and inhibit systemic immunological T cells
In our study, we measured the dynamic expression of T cell subsets in the control and ADSC-treated groups on postoperative day 10 and 14. The results showed that the percentage of CD3+ and CD4+ T cells decreased in both ADSC and hypoxia primed ADSC treated groups on postoperative day 10 and 14, compared with the control group (Figure 3). As shown in previous studies, CD3+ T cell activation and infiltration played a vital role in allograft rejection, which increased along with the severity of rejection in VCA (14). In accordance with the histopathological changes in these groups, the results of this study indicated that ex-vivo treatment using ADSCs significantly inhibited systemic immune rejection during the early stages.
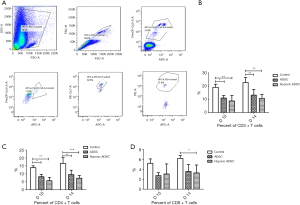
The expression of the pro-inflammatory cytokine, TNF-α, and the anti-inflammatory cytokines, IL-10 and TGF-β, were measured in the serum of recipients on postoperative day 10 and 14 using ELISA. Significantly higher levels of IL-10 in the ADSC and hypoxic ADSC groups were detected on postoperative day 7 and 14 (Figure 4). Further, an increase in TGF-β levels was detected on postoperative day 7 in the ADSC group. Intriguingly, slightly higher TNF-α levels were detected in the ADSC treated group on postoperative day 7, compared with the medium control.
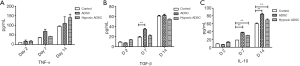
Ex-vivo treatment using ADSCs increased Treg infiltration levels in allografts
Immunohistochemical (IHC) staining of allograft skin tissue revealed a significant increase in Foxp3 expression in the subcutaneous layers of the ADSC and hypoxic ADSC treated groups, compared with the media control, at postoperative day 14 (P<0.05) (Figure 5). The CD4+ CD25+ CD127− FoxP3+ regulatory T (Treg) cells are a unique subset of helper T-cells, which regulate the immune response and establish self-tolerance through the secretion of immunoregulatory cytokines, such as TGF-β and IL-10, and Tregs can arise spontaneously or can be induced in situ. Treg inhibition of acute rejection and immune response modulation during transplantation has been confirmed in a previous study (15). Correspondingly, our study found increased IL-10 levels in the ADSC treated groups, compared with the media control. These results indicate that ex-vivo treatment using ADSCs combined with short-term immunosuppressants could increase the level of Tregs infiltration and provide allografts the ability to develop temporary immunotolerance.
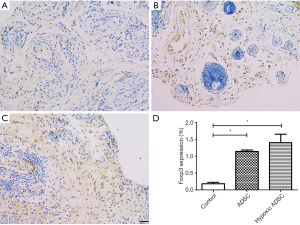
Discussion
VCA technology has improved over the past 50 years, since the first hand transplantation was performed (16). However, the side effects of immunosuppressants still challenge the application of VCA (1). Induction as well as maintenance of recipient immunotolerance towards allografts has become the ultimate objective in the field of transplantation, especially for non-lifesaving VCA. In our study, we attempted ex-vivo allograft engineering via delivery of ADSCs into allografts to modify allografts and induce temporary immunotolerance in the allografts, which may be a promising alternative for the use of pharmacological immunosuppressants alone and confers the possibility of decreasing the dose of systemic immunosuppressants required.
Previous studies have demonstrated the immunomodulatory effects of mesenchymal stem cells and its application in VCA. Kuo et al. (17) found that the systemic delivery of ADSCs to recipients prolonged allograft survival and modulated Treg expression in allografts in a swine model. Soares et al. (5) were the first to attempt ex-vivo perfusion using BMSCs in allografts, and to some extent, prolonged the survival of the allografts. Our study used an improved source of mesenchymal stem cells and mode of administration. However, Soares et al. (5) found that unprimed BMSCs were not able to significantly prolong allograft survival in a hind limb allotransplantation model of rats. In our study, unprimed ADSCs prolonged the survival of allografts, indicating that differences in the source of mesenchymal stem cells may result in diverse immunomodulatory effects on allografts.
In our study, the ex-vivo treatment of allografts using ADSCs was found to prolong the survival time of allografts, while the treatment also inhibited the proliferation and tissue infiltration of lymphocytes, which induced the expression of immunomodulatory cytokines, IL-10 and TGF-β, and to some extent, increased Treg expression levels in allografts. These results proved that the combination of ex-vivo treatment and mesenchymal stem cell therapy may exert an immunomodulatory effect, which is helpful for the induction of immune tolerance in VCA and is an adjuvant therapy for traditional immunosuppressant treatments. In addition, these results are consistent with that of previous studies carried out on swine and rat hind-limb transplantation models of systemic delivery of ADSCs to recipients (17,18), indicating that the ex-vivo treatment of allografts using ADSCs has a similar immunomodulatory effect as that induced by the systemic infusion of ADSCs, without the usual side effects associated with systemic infusion, such as unpredictable cell-induced embolism and trapping of MSCs in the capillaries of the lung and liver (19).
Extraction of the culture medium (CM) of hypoxia-preconditioned ADSCs can enhance these benefits, such as promotion of the survival of grafts, neovascularization, improvement of wound healing and attenuation of inflammation, which have been induced by the CM of ADSCs, as given in previous publications (20,21). The underlying mechanism may be related to hypoxia induced secretion of vascular endothelial growth factor A (VEGF-A) and immunomodulatory cytokines. In our study, hypoxia primed ADSCs showed slightly prolonged survival time of allografts, compared with that of the normoxic ADSC treated group, and induced an increase in Foxp3 expression in the allografts. These results indicate that pretreatment with MSCs may further modify the immunomodulatory results produced by ADSCs. Although the results did not reveal that the beneficial effect is due to the contact-dependent mechanism of the ADSCs or its paracrine effect, pretreatment of ADSCs via hypoxic conditions proved to be an efficient way to improve the immunomodulatory effect of ADSCs in VCA.
At present, there are many issues related with the ex-vivo pretreatment of allografts with MSCs, such as homing of MSCs in allografts, the influence of the recipient’s immune system on this process and the interaction with immunosuppressants, remain unresolved and warrant further studies. Hoogduijn et al. (22) demonstrated that a high dosage of immunosuppressants could induce the apoptosis of MSCs in vitro. Further, the viability and proliferation of ADSCs were found to be more sensitive towards treatment with tacrolimus, compared with BMSCs (23). Thus, the choice of coordinate immunosuppressants and its dosage needs to be further explored by inducing long-term tolerance via the ex-vivo pretreatment using MSCs. In addition, previous study has suggested that ex-vivo infused mesenchymal stem cells engraft within allografts, implying that infused MSCs may form long-term niches (24). However, the length of time and the amount of ADSCs that remain active in allograft tissue need to be determined in further studies.
Conclusions
Ex-vivo treatment using allografts with ADSCs prolonged the survival of allografts, compared with the medium control, suppressed the proliferation and infiltration of T lymphocytes and improved the secretion of immunomodulatory cytokines, such as IL-10, through the potential induction of Treg expression in the allografts. Thus, ex-vivo pretreatment of allografts using ADSCs may be an important adjunctive regimen for the induction of immunotolerance in VCA.
Acknowledgments
We would like to thank the Laboratory of tissue engineering at Shanghai 9th People’s Hospital, Shanghai Jiao Tong University, for providing the equipment and technical guidance necessary to complete the study.
Funding: This study was supported by the National Natural Science Foundation of China (grant number 81401613 to FL, 81871576 to JY).
Footnote
Reporting Checklist: The authors have completed the ARRIVE reporting checklist. Available at http://dx.doi.org/10.21037/atm-19-4730
Peer Review File: Available at http://dx.doi.org/10.21037/atm-19-4730
Data Sharing Statement: Available at http://dx.doi.org/10.21037/atm-19-4730
Conflicts of Interest: All authors have completed the ICMJE uniform disclosure form (available at http://dx.doi.org/10.21037/atm-19-4730). The authors have no conflicts of interest to declare.
Ethical Statement: The authors are accountable for all aspects of the work in ensuring that questions related to the accuracy or integrity of any part of the work are appropriately investigated and resolved. All experiments were approved by the Shanghai Ninth People’s Hospital, Shanghai Jiao Tong University School of Medicine Institutional Animal Care and Use Committee {No. HKDL[2018]214}. Authors are accountable for all aspects of the study.
Open Access Statement: This is an Open Access article distributed in accordance with the Creative Commons Attribution-NonCommercial-NoDerivs 4.0 International License (CC BY-NC-ND 4.0), which permits the non-commercial replication and distribution of the article with the strict proviso that no changes or edits are made and the original work is properly cited (including links to both the formal publication through the relevant DOI and the license). See: https://creativecommons.org/licenses/by-nc-nd/4.0/.
References
- Khalifian S, Brazio PS, Mohan R, et al. Facial transplantation: the first 9 years. Lancet 2014;384:2153-63. [Crossref] [PubMed]
- Bamoulid J, Staeck O, Halleck F, et al. The need for minimization strategies: current problems of immunosuppression. Transpl Int 2015;28:891-900. [Crossref] [PubMed]
- Balfoussia D, Yerrakalva D, Hamaoui K, et al. Advances in machine perfusion graft viability assessment in kidney, liver, pancreas, lung, and heart transplant. Exp Clin Transplant 2012;10:87-100. [Crossref] [PubMed]
- Van Raemdonck D, Neyrinck A, Rega F, et al. Machine perfusion in organ transplantation: a tool for ex-vivo graft conditioning with mesenchymal stem cells? Curr Opin Organ Transplant 2013;18:24-33. [Crossref] [PubMed]
- Soares MA, Massie JP, Rifkin WJ, et al. Ex vivo allotransplantation engineering: Delivery of mesenchymal stem cells prolongs rejection-free allograft survival. Am J Transplant 2018;18:1657-67. [Crossref] [PubMed]
- Hattori H, Sato M, Masuoka K, et al. Osteogenic potential of human adipose tissue-derived stromal cells as an alternative stem cell source. Cells Tissues Organs 2004;178:2-12. [Crossref] [PubMed]
- Cui L, Yin S, Liu W, et al. Expanded adipose-derived stem cells suppress mixed lymphocyte reaction by secretion of prostaglandin E2. Tissue Eng 2007;13:1185-95. [Crossref] [PubMed]
- Cheng HY, Ghetu N, Huang WC, et al. Syngeneic adipose-derived stem cells with short-term immunosuppression induce vascularized composite allotransplantation tolerance in rats. Cytotherapy 2014;16:369-80. [Crossref] [PubMed]
- Wang Y, Wang X, Zhou X, et al. Suppressive effect mediated by human adipose-derived stem cells on T cells involves the activation of JNK. Int J Mol Med 2019;43:177-84. [PubMed]
- Oda H, Ikeguchi R, Aoyama T, et al. Relative antigenicity of components in vascularized composite allotransplants: An experimental study of microRNAs expression in rat hind limb transplantation model. Microsurgery 2019;39:340-8. [Crossref] [PubMed]
- Gu C, Liu F, Luo X, et al. Triptolide Reduces the Required Dose of Tacrolimus by Attenuating Inflammation, Enhancing Immunosuppression, and Increasing Donor Chimerism in a Heterotopic Hindlimb Transplantation Model. Plast Reconstr Surg 2016;138:1243-53. [Crossref] [PubMed]
- Cendales LC, Kanitakis J, Schneeberger S, et al. The Banff 2007 working classification of skin-containing composite tissue allograft pathology. Am J Transplant 2008;8:1396-400. [Crossref] [PubMed]
- Bunnell BA, Flaat M, Gagliardi C, et al. Adipose-derived stem cells: isolation, expansion and differentiation. Methods 2008;45:115-20. [Crossref] [PubMed]
- Hautz T, Zelger B, Grahammer J, et al. Molecular markers and targeted therapy of skin rejection in composite tissue allotransplantation. Am J Transplant 2010;10:1200-9. [Crossref] [PubMed]
- Rothstein DM, Camirand G. New insights into the mechanisms of Treg function. Curr Opin Organ Transplant 2015;20:376-84. [Crossref] [PubMed]
- Zuo KJ, Olson JL. The evolution of functional hand replacement: From iron prostheses to hand transplantation. Plast Surg (Oakv) 2014;22:44-51. [Crossref] [PubMed]
- Kuo YR, Chen CC, Chen YC, et al. Recipient Adipose-Derived Stem Cells Enhance Recipient Cell Engraftment and Prolong Allotransplant Survival in a Miniature Swine Hind-Limb Model. Cell Transplant 2017;26:1418-27. [Crossref] [PubMed]
- Plock JA, Schnider JT, Zhang W, et al. Adipose- and Bone Marrow-Derived Mesenchymal Stem Cells Prolong Graft Survival in Vascularized Composite Allotransplantation. Transplantation 2015;99:1765-73. [Crossref] [PubMed]
- Boltze J, Arnold A, Walczak P, et al. The Dark Side of the Force - Constraints and Complications of Cell Therapies for Stroke. Front Neurol 2015;6:155. [Crossref] [PubMed]
- Han YD, Bai Y, Yan XL, et al. Co-transplantation of exosomes derived from hypoxia-preconditioned adipose mesenchymal stem cells promotes neovascularization and graft survival in fat grafting. Biochem Biophys Res Commun 2018;497:305-12. [Crossref] [PubMed]
- Ong HT, Redmond SL, Marano RJ, et al. Paracrine Activity from Adipose-Derived Stem Cells on In Vitro Wound Healing in Human Tympanic Membrane Keratinocytes. Stem Cells Dev 2017;26:405-18. [Crossref] [PubMed]
- Hoogduijn MJ, Crop MJ, Korevaar SS, et al. Susceptibility of Human Mesenchymal Stem Cells to Tacrolimus, Mycophenolic Acid, and Rapamycin. Transplantation 2008;86:1283-91. [Crossref] [PubMed]
- Tsuji W, Schnider JT, McLaughlin MM, et al. Effects of immunosuppressive drugs on viability and susceptibility of adipose- and bone marrow-derived mesenchymal stem cells. Front Immunol 2015;6:131. [Crossref] [PubMed]
- Chang EI, Bonillas RG, El-ftesi S, et al. Tissue engineering using autologous microcirculatory beds as vascularized bioscaffolds. FASEB J 2009;23:906-15. [Crossref] [PubMed]