Galectins in tumor angiogenesis
Introduction
The vascular bed forms a complex infrastructure allowing the transport of molecules and cells within the body. The pavement of this infrastructure is composed of endothelial cells (EC), i.e., the cells that line the luminal side of blood vessels. These cells act as a semi-permeable barrier and control the transfer of molecules and cells from the circulation to the underlying tissue and vice versa. In addition, the endothelium contributes to many different physiologic processes including coagulation, inflammation, and angiogenesis (1). In the latter process, i.e., the growth of new blood vessels out of pre-existing capillaries, EC are in fact the key players. To initiate blood vessel growth, the EC have to become activated since the turnover of these cells is low (2). Endothelial activation triggers a tightly regulated cascade of cellular activities, including extracellular matrix degradation, cell sprouting, proliferation, migration, and tube formation (Figure 1). Once new vessels have been formed the angiogenesis process ends with the deposition of new extracellular matrix, the attraction of pericytes for vessel stabilization, and a return to the quiescent EC phenotype (1,3).
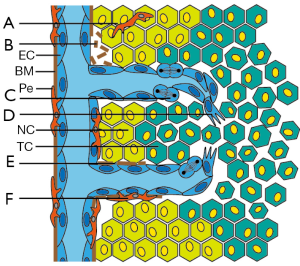
Already 40 years ago, it was recognized that most solid tumors rely on angiogenesis for continuous growth (4). Nowadays, tumor angiogenesis is considered a hallmark of cancer and an attractive target for cancer therapy (1,5,6). To induce tumor angiogenesis, tumor cells (TC) that are under metabolic/hypoxic stress undergo an angiogenic switch which results in the increased expression and release of angiostimulatory growth factors. Binding of these growth factors to their receptors on the EC surface results in activation of the angiogenesis cascade (1,6). In recent years, it has become evident that galectins can contribute to EC activation as well as to other steps of the angiogenesis cascade. This has identified galectins as potential target molecules for angiostatic cancer therapy (7,8). This review summarizes the recent advances in our knowledge on the role of galectins in tumor angiogenesis.
Galectin expression in the tumor endothelium
Thus far, 15 galectin family members have been identified and 11 of these are also expressed by mammalian cells. EC mainly express galectin-1, -3, -8, and -9 at the mRNA and protein level (9). These galectins can be found at the cell surface, in the cytoplasm and even in the nucleus of EC (9,10). The expression and localization depends on the EC activation status as well as on the environmental context in which the cells reside (8). Consequently, galectin expression in tumor vessels is often different as compared to vessels in normal tissues. For example, increased endothelial galectin-1 expression has been reported in tumor EC of e.g., prostate cancer (11,12), lung cancer (13), oral cancer (14), and colon cancer (9,15). In astrocytic brain tumors galectin-1 staining in the vessel wall was even found to significantly increase with increasing tumor grade (16). Aberrant endothelial galectin-3 expression has also been found in different tumors including breast (17), head and neck (13), lung (13), and colon (9). In primary central nervous system lymphomas multivariate analysis identified increased endothelial galectin-3 expression as an independent prognostic factor (18). The progression of certain brain tumors appears to be associated with loss of endothelial galectin-3 expression (19-21), albeit that this expression loss mainly occurs in the central tumor area and not in the tumor periphery (20). With respect to the expression of galectin-8 in tumor endothelium little is known. We observed only sporadic nuclear staining in colon tumor endothelium (9). Similar observations were made in breast and prostate cancer tissues although there, faint cytoplasmic expression was also reported (10). Neither study observed major differences in the expression between normal and cancerous tissue. In astrocytic brain tumors the expression of galectin-8 in the vessel wall was also reported. Subsequent comparison of the expression levels between patients that died within 1 year following diagnosis and those that survived for at least 2 years did not reveal any significant differences (22). These findings do not point towards a prognostic value of vascular galectin-8 staining in tumor tissue.
Similar as for galectin-8, only limited information is available on specific endothelial galectin-9 expression in cancer. In general, loss of galectin-9 expression is associated with poor survival. However, most studies that reported on this inverse correlation did not distinguish between the different cellular compartments (23-25). Recently, we described that endothelial galectin-9 expression was elevated in lung, liver, and kidney cancer tissues as compared to normal tissue (26). This appeared to be in contrast with our previous observation that galectin-9 expression was decreased in activated EC in vitro (9). However, this discrepancy only illustrates that the cellular context and locoregional differences in the tumor microenvironment influence galectin expression. For example, it is known that e.g., galectin-1 expression can be induced under hypoxic conditions (27-29) although not necessarily though HIF proteins (29). Interactions of EC with e.g., TC or inflammatory cells have also been shown to alter the expression and localization of endothelial galectins (30-33). Moreover, components of the extracellular matrix as well as factors secreted by TC or inflammatory cells can affect endothelial galectin expression. For example, interferon gamma can induce the expression of galectin-1 and galectin-9 (34-36) while interleukin-1b induces endothelial galectin-1 and galectin-3 expression (34,37). Interestingly, EC grown on fibronectin show increased galectin-3 expression but decreased interleukin-1b expression (38). All these data exemplify the complex regulation of endothelial galectin expression which is most likely further influenced by the expression and presence of specific glycan-ligands on galectin-binding proteins (32). Nevertheless, there is ample evidence that tumor-associated EC express multiple galectins and that the expression level and protein localization can be distinct from endothelium in normal tissues.
Galectins and EC function in vitro
As evidenced in the previous paragraphs, endothelial galectin expression is often altered in EC of cancerous tissues as compared to those in normal tissues. This is most likely related to the different environmental conditions in tumor tissues which are in general angiostimulatory. Thus, it can be anticipated that the altered galectin expression contributes to EC function during angiogenesis. As already described, EC adopt different roles during neovessel formation which requires different cellular activities (Figure 1). Galectins have been shown to be involved in proper execution of these activities. In general galectins appear to promote EC function in vitro, but the exact effect depends on multiple parameters, like galectin concentration, EC source, and environmental context.
EC activation
The first step in the angiogenesis cascade is the activation of quiescent EC. This is mediated through different growth factors like VEGF, EGF and bFGF, that bind to cell surface receptors thereby triggering intracellular downstream signaling. Galectins have been shown to facilitate signaling both extracellularly and intracellularly (39). On the cell surface, galectins can enhance or prolong signaling by mediating interactions between (co)receptors or by hampering the uptake of receptors (14,40-42). A recent study showed that galectin-1 stimulates VEGFR2 signaling even in the absence of ligand (43) confirming previous findings that galectin-1 can act by itself as a proangiogenic growth factor (44). Whether this is a common mechanism that also underlies the proangionic activity of other galectins still needs further investigation. Interestingly, the extracellular functions described above are mostly glycan-dependent while intracellulary, galectins can engage in direct protein-protein interactions with Ras proteins to facilitate further downstream signaling (45). For example, galectin-1 augments signaling via interactions with H-Ras while galectin-3 directs signaling downstream from K-Ras (46,47). The exact role of galectin-8 and galectin-9 in intracellular signaling has not been resolved.
EC proliferation
The activation of EC by growth factors results in increased cell proliferation. By inducing or maintaining this activation galectins directly contribute to regulation of EC proliferation. Indeed, we and other have shown galectin-1 can induce EC proliferation (44) and that interfering with galectin-1 expression or function results in lower proliferation rates (15,29). Galectin-3 has also been shown to induce EC growth (40). On the other hand, the effects on proliferation appear to be cell type and concentration dependent as not all EC respond equally to galectin-1 and galectin-3 treatment (40) while high concentration of exogenous galectins can even inhibit EC growth (44). Cell type and concentration dependent inhibition of EC proliferation was also observed following treatment with galectin-9 (26). However, the role of this galectin requires further study as multiple splice variants have been identified in EC which might exert different functions (26,48). The same holds true for galectin-8.
EC adhesion/migration
Once activated, the EC have to migrate towards the angiogenic stimulus. This requires continuous interaction of the cells with their environment. Galectins have been shown to be involved in these interactions thereby modulating EC migration. For example, we have shown that, similar to proliferation, exogenous galectin-1 can promote migration while blocking galectin-1 reduces the migratory capacity (15,44). This was also reported by Croci et al. (29) and Hsieh et al. (14). The latter group also showed that galectin-1 modulates the adhesion of EC to matrix components like laminin and fibronectin (14). Galectin-3 has also been associated with EC migration. Knockdown of endogenous galectin-3 expression reduces the migratory activity of EC (41). In addition, galectin-3 promotes migration by serving as an exogenous chemoattractant (41,49,50). The activity of galectin-3 appears to mainly involve stimulation of pro-migratory signaling via clustering of integrin alphavbeta3, stimulation of integrin-linked kinase activity, or maintaining VEGF receptor signaling (40,41,51).
Regarding the tandem repeat galectins, EC migration on a gelatin coating that contained galectin-8 was shown to be enhanced while knockdown of endogenous expression reduced EC migration (10). Exogenously applied galectin-9 can both induce and inhibit migration, depending on the concentration. On the other hand, increasing the endogenous galectin-9 expression does not seem to affect EC migration (26). However, the latter might depend on environmental conditions and further research is required to fully understand how the different splice variants of galectin-8 and galectin-9 modulate EC migration.
EC tube formation and sprouting
Cell proliferation and migration are important features during angiogenesis. However, more specific EC functions involve the capacity to form tube-like structures on 2D matrices and to sprout into 3D matrices. There is ample evidence that galectins are involved in regulating both processes. For example, EC isolated from galectin-1 knockout mice show hampered tube formation on matrigel (52). While this suggests that EC require galectin-1 for tube formation, Le Mercier et al. reported no difference in tube formation between normal HUVEC and HUVEC transfected with anti-gal-1 siRNA (53). Most likely, this discrepancy is related to the degree of expression knockdown. Apart from endogenous galectin-1, Croci et al. showed that galectin-1 secreted by Kaposi’s sarcoma cells could regulate tube formation by HUVEC (29). The galectin-1-mediated tube formation could be neutralized by lactose as well as by a galectin-1 blocking antibody (29). Similar observations were made by Laderach et al. using prostate cancer cells (12) and by D’haene et al. and Croci et al. using recombinant galectin-1 (40,43). Both latter studies linked the increased activity to elevated VEGFR2 signaling (40,43). Moreover, D’haene et al. also observed increased tube formation in the presence of galectin-3 (40). The latter confirmed previous findings by Markowska et al. (41,42) and Nangia-Makker et al. who also found increased tube-forming activity of EC in response to a processed form of galectin-3 (49,50,54). In addition, knockdown of endogenous galectin-3 expression resulted in reduced tube forming capacity (41) and hampered sprouting (42). Similar as for galectin-1, the stimulatory function of galectin-3 appears to be directly related to enhanced VEGF signaling (40-42). Whether this is also true for the other vascular galectins remains to be studied. Regarding this, exogenously applied galectin-8 was found to stimulate tube formation on matrigel while knockdown of endogenous galectin-8 expression reduced tube formation (10). While several galectin-8-binding proteins have been identified that might underlie this activity, e.g., CD166 and several members of the integrin family (55), an interaction with VEGF receptors has not yet been reported. Of note, Cueni et al. only observed a stimulatory effect of galectin-8 on tube formation by lymphatic EC and not by HUVEC (56). In addition, these effects depended on the context in which galectin-8 was presented to the cells, i.e., soluble or immobilized (56). This shows that still little is known regarding the exact function of galectin-8 in EC function during tube formation. The same is true for galectin-9 and additional studies are required to fully dissect the role of both tandem repeat galectins in EC tube formation and sprouting.
Galectins and tumor angiogenesis in vivo
As described above, galectins contribute to multiple steps of the angiogenesis cascade (Table 1). Consequently, vascular galectins have been found to contribute to tumor angiogenesis in vivo. Surprisingly, mouse knockout strains that lack one or more galectin genes are viable and do not present severe vascular defects (57-59). Only recently, we observed a small and transient growth delay in pups from galectin-1 null mice that might be due to impaired vascularization (60). Interestingly, under more pathological conditions, the absence of galectins does result in hampered angiogenic responses. For example, in the mouse corneal micropocket assay, bFGF and VEGF-induced neo-vascularization was impaired in galectin-3-/- mice (41). Likewise, using a corneal suture model, Markowska et al. observed reduced corneal neovascularization in galectin-3-/- mice as compared to wild-type animals (42). More compelling evidence for a role of galectin-3 in tumor angiogenesis was provided by Nangia-Makker et al. They performed matrigel plug assays with breast cancer cells with either absent or high galectin-3 expression. The latter cells significantly induced vascularization of the TC-containing matrigel plugs (61). In a subsequent study the authors showed that targeting galectin-3 with a modified citrus pectin reduced tumor growth and metastasis of breast and colon cancer cells. In treated mice, the number of blood vessels in the breast cell tumors was reduced to approximately 30% as compared untreated mice (49). In a more recent study, the angiostimulatory activity in in vivo breast cancer progression was linked to MMP processing of the N-terminal ‘tail’ of galectin-3 (50). Altogether, these findings suggest a role for galectin-3 in tumor angiogenesis during tumor progression in vivo. On the other hand, we did not see differences in tumor progression or tumor vascularization when comparing genetic mouse tumor models in galectin-3-/- or wildtype mice (62). Possibly, the relatively slow development of tumors in these models allowed the development of adaptive mechanisms. This might also explain the lack of an overt vascular phenotype in galectin-deficient mice. Regarding the adaptive mechanisms, it would be interesting to determine the contribution of galectin-1 in galectin-3-/- mice as this galectin has also been linked to tumor angiogenesis and cancer progression. For example, we have shown that tumor growth is hampered in galectin-1-/- mice due to impaired angiogenesis (15). Additional experiments showed that this inhibitory effect was tumor type independent although tumors could partially compensate the lack of endothelial galectin-1 by secreting the protein (44). This was later confirmed by Laderach et al. who observed that the induction of angiogenesis by tumor-secreted galectin-1 was reduced by anti-galectin-1 antibodies or by knockdown of galectin-1 expression in the TC (12). Furthermore, knockdown of galectin-1 in B16 melanoma cells and subsequent injection of these cells in either wildtype or galectin-1-/- mice prolonged the survival as compared to injection of wildtype melanoma cells. This was also suggested to be related to the angiostimulatory activity of tumor-secreted galectin-1 (52). Croci et al. also showed that TC derived galectin-1 induces angiogenesis in vivo. The authors performed matrigel plug assays with culture medium of Kaposi’s sarcoma cells that were either wildtype or galectin-1 knockdown. The latter showed considerable lower vascularization (29). In addition, when the knockdown cells were grafted into nude mice, tumor growth was inhibited compared to wildtype cells. This was accompanied by a decreased amount of EC in the galectin-1 knockdown tumors (29). More recently, the same group showed that tumor derived galectin-1 could even counteract VEGF-targeted angiostatic therapy by facilitating VEGF receptor signaling in the absence of VEGF. Blocking this escape mechanism by anti-galectin-1 antibodies resulted in vascular remodeling and inhibition of tumor growth (43). All these findings show that galectin-1 is involved in the induction and maintenance of tumor angiogenesis during tumor growth. Moreover, targeting galectin-1 could provide a therapeutic opportunity (15,43).
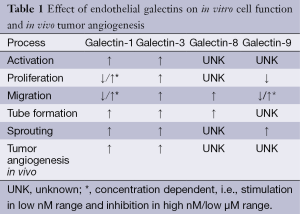
Full table
In line with the limited information on galectin-8 and galectin-9 in EC biology, the role of both galectins as regulators tumor angiogenesis in vivo is not well studied. Cardenas-Delgado observed increased ingrowth of vessels in matrigel plugs supplemented with galectin-8 that were subcutaneously injected in mice (10). This suggests that galectin-8 might stimulate tumor angiogenesis but experiments comparing in vivo growth of TC with or without galectin-8 expression as well as tumor models in galectin-8-/- mice are still lacking. Regarding galectin-9, we observed a small decrease in in vivo angiogenesis during treatment of the chicken chorioallantoic membrane (26). As already described, we frequently observed increased galectin-9 expression in EC from human tumor tissues. This does point towards a role in tumor progression but similar as for galectin-8 further studies in animal models are required to uncover this role.
Concluding remarks
Galectins have emerged as important regulators of tumor progression that influence different hallmarks of cancer, e.g., TC transformation, tumor immune escape, and tumor angiogenesis (7,8,63). Regarding the latter, four galectins are frequently reported to be expressed in the tumor endothelium, i.e., galectin-1, galectin-3, galectin-8 and galectin-9 (9). Ongoing research has shown that at least galectin-1 and galectin-3 and most likely also galectin-8 and galectin-9, contribute to proper EC function during tumor angiogenesis. These galectins are not only involved in initial EC activation but also contribute to the subsequent steps of the angiogenesis cascade. All this has identified galectins as potential targets for angiostatic cancer therapy (64). Consequently, several compounds have been developed that interfere with galectin function in either tumor angiogenesis or in other tumor-promoting activities including immunosuppression and metastasis (15,43,49,65-67). However, further development of effective galectin-targeted therapy relies on a better understanding of the exact role of endothelial galectins in tumor angiogenesis. An important aspect of endothelial galectin biology that needs to be resolved is the regulation of galectin expression. Multiple triggers have been found to influence endothelial expression, including hypoxia, cytokines, and cell-cell interactions [see reference (8)]. However, which intracellular signaling pathways and transcription factors control galectin expression in response to these triggers is still poorly understood. A better insight in the regulatory pathways will help to understand how and when endothelial galectin expression is modulated during tumor angiogenesis and how and when interfering with endothelial galectin expression could be beneficial.
Another important issue to address is the cross-talk between the different galectins during angiogenesis. As D’Haene already showed, combined application of galectins can potentiate their activity (40). On the other hand, we and others have shown that the activity of galectins is concentration dependent and can be both stimulatory as inhibitory (44). All this already suggests a complex fine-tuning mechanism by which galectins control EC function. This mechanism becomes even more complex when taking into account that several protein modifications have been identified including splicing, phosphorylation, and proteolytic processing, that further affect galectin function (26,50,68-70). In addition, still relatively little is known regarding the expression regulation of galectin-binding glycans in the (tumor) endothelium. Using an elegant set of experiments Croci et al. showed the importance of N-glycosylation in controlling the angiogenic activity of galectin-1 (29,43). Further unraveling these galectin-glycan axes is one of the major challenges for future research as it will provide leads for the development of novel galectin-targeting agents for the treatment of cancer.
Acknowledgements
Disclosure: The authors declare no conflict of interest.
References
- Griffioen AW, Molema G. Angiogenesis: potentials for pharmacologic intervention in the treatment of cancer, cardiovascular diseases, and chronic inflammation. Pharmacol Rev 2000;52:237-68. [PubMed]
- Hobson B, Denekamp J. Endothelial proliferation in tumours and normal tissues: continuous labelling studies. Br J Cancer 1984;49:405-13. [PubMed]
- Potente M, Gerhardt H, Carmeliet P. Basic and therapeutic aspects of angiogenesis. Cell 2011;146:873-87. [PubMed]
- Folkman J. Tumor angiogenesis: therapeutic implications. N Engl J Med 1971;285:1182-6. [PubMed]
- Hanahan D, Weinberg RA. Hallmarks of cancer: the next generation. Cell 2011;144:646-74. [PubMed]
- Carmeliet P, Jain RK. Molecular mechanisms and clinical applications of angiogenesis. Nature 2011;473:298-307. [PubMed]
- Thijssen VL, Poirier F, Baum LG, et al. Galectins in the tumor endothelium; opportunities for combined cancer therapy. Blood 2007;110:2819-27. [PubMed]
- Thijssen VL, Rabinovich GA, Griffioen AW. Vascular galectins: regulators of tumor progression and targets for cancer therapy. Cytokine Growth Factor Rev 2013;24:547-58. [PubMed]
- Thijssen VL, Hulsmans S, Griffioen AW. The galectin profile of the endothelium: altered expression and localization in activated and tumor endothelial cells. Am J Pathol 2008;172:545-53. [PubMed]
- Delgado VM, Nugnes LG, Colombo LL, et al. Modulation of endothelial cell migration and angiogenesis: a novel function for the “tandem-repeat” lectin galectin-8. Faseb J 2011;25:242-54. [PubMed]
- Clausse N, van den Brule F, Waltregny D, et al. Galectin-1 expression in prostate tumor-associated capillary endothelial cells is increased by prostate carcinoma cells and modulates heterotypic cell-cell adhesion. Angiogenesis 1999;3:317-25. [PubMed]
- Laderach DJ, Gentilini LD, Giribaldi L, et al. A Unique Galectin Signature in Human Prostate Cancer Progression Suggests Galectin-1 as a Key Target for Treatment of Advanced Disease. Cancer Res 2013;73:86-96. [PubMed]
- Lotan R, Belloni PN, Tressler RJ, et al. Expression of galectins on microvessel endothelial cells and their involvement in tumour cell adhesion. Glycoconj J 1994;11:462-8. [PubMed]
- Hsieh SH, Ying NW, Wu MH, et al. Galectin-1, a novel ligand of neuropilin-1, activates VEGFR-2 signaling and modulates the migration of vascular endothelial cells. Oncogene 2008;27:3746-53. [PubMed]
- Thijssen VL, Postel R, Brandwijk RJ, et al. Galectin-1 is essential in tumor angiogenesis and is a target for antiangiogenesis therapy. Proc Natl Acad Sci U S A 2006;103:15975-80. [PubMed]
- Rorive S, Belot N, Decaestecker C, et al. Galectin-1 is highly expressed in human gliomas with relevance for modulation of invasion of tumor astrocytes into the brain parenchyma. Glia 2001;33:241-55. [PubMed]
- Moisa A, Fritz P, Eck A, et al. Growth/adhesion-regulatory tissue lectin galectin-3: stromal presence but not cytoplasmic/nuclear expression in tumor cells as a negative prognostic factor in breast cancer. Anticancer Res 2007;27:2131-9. [PubMed]
- D’Haene N, Catteau X, Maris C, et al. Endothelial hyperplasia and endothelial galectin-3 expression are prognostic factors in primary central nervous system lymphomas. Br J Haematol 2008;140:402-10. [PubMed]
- Deininger MH, Trautmann K, Meyermann R, et al. Galectin-3 labeling correlates positively in tumor cells and negatively in endothelial cells with malignancy and poor prognosis in oligodendroglioma patients. Anticancer Res 2002;22:1585-92. [PubMed]
- Gordower L, Decaestecker C, Kacem Y, et al. Galectin-3 and galectin-3-binding site expression in human adult astrocytic tumours and related angiogenesis. Neuropathol Appl Neurobiol 1999;25:319-30. [PubMed]
- Strik HM, Deininger MH, Frank B, et al. Galectin-3: cellular distribution and correlation with WHO-grade in human gliomas. J Neurooncol 2001;53:13-20. [PubMed]
- Camby I, Belot N, Rorive S, et al. Galectins are differentially expressed in supratentorial pilocytic astrocytomas, astrocytomas, anaplastic astrocytomas and glioblastomas, and significantly modulate tumor astrocyte migration. Brain Pathol 2001;11:12-26. [PubMed]
- Kageshita T, Kashio Y, Yamauchi A, et al. Possible role of galectin-9 in cell aggregation and apoptosis of human melanoma cell lines and its clinical significance. Int J Cancer 2002;99:809-16. [PubMed]
- Jiang J, Jin MS, Kong F, et al. Decreased galectin-9 and increased tim-3 expression are related to poor prognosis in gastric cancer. PLoS One 2013;8:e81799. [PubMed]
- Zhang ZY, Dong JH, Chen YW, et al. Galectin-9 acts as a prognostic factor with antimetastatic potential in hepatocellular carcinoma. Asian Pac J Cancer Prev 2012;13:2503-9. [PubMed]
- Heusschen R, Schulkens IA, van Beijnum J, et al. Endothelial LGALS9 splice variant expression in endothelial cell biology and angiogenesis. Biochim Biophys Acta 2014;1842:284-92.
- Zhao XY, Chen TT, Xia L, et al. Hypoxia inducible factor-1 mediates expression of galectin-1: the potential role in migration/invasion of colorectal cancer cells. Carcinogenesis 2010;31:1367-75. [PubMed]
- Zhao XY, Zhao KW, Jiang Y, et al. Synergistic induction of galectin-1 by C/EBP{alpha} and HIF-1{alpha} and its role in differentiation of acute myeloid leukemic cells. J Biol Chem 2011;286:36808-19. [PubMed]
- Croci DO, Salatino M, Rubinstein N, et al. Disrupting galectin-1 interactions with N-glycans suppresses hypoxia-driven angiogenesis and tumorigenesis in Kaposi's sarcoma. J Exp Med 2012;209:1985-2000. [PubMed]
- Glinsky VV, Huflejt ME, Glinsky GV, et al. Effects of Thomsen-Friedenreich antigen-specific peptide P-30 on beta-galactoside-mediated homotypic aggregation and adhesion to the endothelium of MDA-MB-435 human breast carcinoma cells. Cancer Res 2000;60:2584-8. [PubMed]
- Glinsky VV, Glinsky GV, Glinskii OV, et al. Intravascular metastatic cancer cell homotypic aggregation at the sites of primary attachment to the endothelium. Cancer Res 2003;63:3805-11. [PubMed]
- Glinskii OV, Turk JR, Pienta KJ, et al. Evidence of porcine and human endothelium activation by cancer-associated carbohydrates expressed on glycoproteins and tumour cells. J Physiol 2004;554:89-99. [PubMed]
- Gil CD, La M, Perretti M, et al. Interaction of human neutrophils with endothelial cells regulates the expression of endogenous proteins annexin 1, galectin-1 and galectin-3. Cell Biol Int 2006;30:338-44. [PubMed]
- Baum LG, Seilhamer JJ, Pang M, et al. Synthesis of an endogeneous lectin, galectin-1, by human endothelial cells is up-regulated by endothelial cell activation. Glycoconj J 1995;12:63-8. [PubMed]
- Imaizumi T, Kumagai M, Nishi N, et al. 15-deoxy-delta(12,14)-prostaglandin J2 inhibits IFN-gamma-induced galectin-9 expression in cultured human umbilical vein endothelial cells. Int Arch Allergy Immunol 2003;131:57-61. [PubMed]
- Imaizumi T, Kumagai M, Sasaki N, et al. Interferon-{gamma} stimulates the expression of galectin-9 in cultured human endothelial cells. J Leukoc Biol 2002;72:486-91. [PubMed]
- Rao SP, Wang Z, Zuberi RI, et al. Galectin-3 functions as an adhesion molecule to support eosinophil rolling and adhesion under conditions of flow. J Immunol 2007;179:7800-7. [PubMed]
- Ahrens I, Domeij H, Topcic D, et al. Successful in vitro expansion and differentiation of cord blood derived CD34+ cells into early endothelial progenitor cells reveals highly differential gene expression. PLoS One 2011;6:e23210. [PubMed]
- Compagno D, Jaworski FM, Gentilini L, et al. Galectins: Major Signaling Modulators Inside and Outside the Cell. Curr Mol Med 2014;14:630-51. [PubMed]
- D’Haene N, Sauvage S, Maris C, et al. VEGFR1 and VEGFR2 Involvement in Extracellular Galectin-1- and Galectin-3-Induced Angiogenesis. PLoS One 2013;8:e67029. [PubMed]
- Markowska AI, Liu FT, Panjwani N. Galectin-3 is an important mediator of VEGF- and bFGF-mediated angiogenic response. J Exp Med 2010;207:1981-93. [PubMed]
- Markowska AI, Jefferies KC, Panjwani N. Galectin-3 protein modulates cell surface expression and activation of vascular endothelial growth factor receptor 2 in human endothelial cells. J Biol Chem 2011;286:29913-21. [PubMed]
- Croci DO, Cerliani JP, Dalotto-Moreno T, et al. Glycosylation-Dependent Lectin-Receptor Interactions Preserve Angiogenesis in Anti-VEGF Refractory Tumors. Cell 2014;156:744-58. [PubMed]
- Thijssen VL, Barkan B, Shoji H, et al. Tumor cells secrete galectin-1 to enhance endothelial cell activity. Cancer Res 2010;70:6216-24. [PubMed]
- Belanis L, Plowman SJ, Rotblat B, et al. Galectin-1 is a novel structural component and a major regulator of h-ras nanoclusters. Mol Biol Cell 2008;19:1404-14. [PubMed]
- Elad-Sfadia G, Haklai R, Ballan E, et al. Galectin-1 augments Ras activation and diverts Ras signals to Raf-1 at the expense of phosphoinositide 3-kinase. J Biol Chem 2002;277:37169-75. [PubMed]
- Elad-Sfadia G, Haklai R, Balan E, et al. Galectin-3 augments K-Ras activation and triggers a Ras signal that attenuates ERK but not phosphoinositide 3-kinase activity. J Biol Chem 2004;279:34922-30. [PubMed]
- Thijssen VL, Griffioen AW. Galectin-1 and -9 in angiogenesis; A sweet couple. Glycobiology 2014;24:915-20. [PubMed]
- Nangia-Makker P, Hogan V, Honjo Y, et al. Inhibition of human cancer cell growth and metastasis in nude mice by oral intake of modified citrus pectin. J Natl Cancer Inst 2002;94:1854-62. [PubMed]
- Nangia-Makker P, Wang Y, Raz T, et al. Cleavage of galectin-3 by matrix metalloproteases induces angiogenesis in breast cancer. Int J Cancer 2010;127:2530-41. [PubMed]
- Wesley UV, Vemuganti R, Ayvaci ER, et al. Galectin-3 enhances angiogenic and migratory potential of microglial cells via modulation of integrin linked kinase signaling. Brain Res 2013;1496:1-9. [PubMed]
- Mathieu V, de Lassalle EM, Toelen J, et al. Galectin-1 in melanoma biology and related neo-angiogenesis processes. J Invest Dermatol 2012;132:2245-54. [PubMed]
- Le Mercier M, Mathieu V, Haibe-Kains B, et al. Knocking down galectin 1 in human hs683 glioblastoma cells impairs both angiogenesis and endoplasmic reticulum stress responses. J Neuropathol Exp Neurol 2008;67:456-69. [PubMed]
- Nangia-Makker P, Balan V, Raz A. Regulation of tumor progression by extracellular galectin-3. Cancer Microenviron 2008;1:43-51. [PubMed]
- Troncoso MF, Ferragut F, Bacigalupo ML, et al. Galectin-8: a matricellular lectin involved in vascular and lymphatic angiogenesis. Glycobiology 2014;24:907-14. [PubMed]
- Cueni LN, Detmar M. Galectin-8 interacts with podoplanin and modulates lymphatic endothelial cell functions. Exp Cell Res 2009;315:1715-23. [PubMed]
- Colnot C, Fowlis D, Ripoche MA, et al. Embryonic implantation in galectin 1/galectin 3 double mutant mice. Dev Dyn 1998;211:306-13. [PubMed]
- Poirier F, Robertson EJ. Normal development of mice carrying a null mutation in the gene encoding the L14 S-type lectin. Development 1993;119:1229-36. [PubMed]
- Seki M, Oomizu S, Sakata KM, et al. Galectin-9 suppresses the generation of Th17, promotes the induction of regulatory T cells, and regulates experimental autoimmune arthritis. Clin Immunol 2008;127:78-88. [PubMed]
- Freitag N, Tirado-González I, Barrientos G, et al. Interfering with Gal-1-mediated angiogenesis contributes to the pathogenesis of preeclampsia. Proc Natl Acad Sci U S A 2013;110:11451-6. [PubMed]
- Nangia-Makker P, Honjo Y, Sarvis R, et al. Galectin-3 induces endothelial cell morphogenesis and angiogenesis. Am J Pathol 2000;156:899-909. [PubMed]
- Eude-Le Parco I, Gendronneau G, Dang T, et al. Genetic assessment of the importance of galectin-3 in cancer initiation, progression, and dissemination in mice. Glycobiology 2009;19:68-75. [PubMed]
- Liu FT, Rabinovich GA. Galectins as modulators of tumour progression. Nat Rev Cancer 2005;5:29-41. [PubMed]
- Schulkens IA, Griffioen AW, Thijssen VL. Angiostatic cancer therapy by targeting galectins in the tumor vasculature. In: Klyosov A, Traber PG. eds. ACS Symposium Series: Galectins and Disease Implications for Targeted Therapeutics. Washington DC: ACS Publications, 2012:233-47.
- Rabinovich GA, Cumashi A, Bianco GA, et al. Synthetic lactulose amines: novel class of anticancer agents that induce tumor-cell apoptosis and inhibit galectin-mediated homotypic cell aggregation and endothelial cell morphogenesis. Glycobiology 2006;16:210-20. [PubMed]
- Salomonsson E, Thijssen VL, Griffioen AW, et al. The anti-angiogenic peptide anginex greatly enhances galectin-1 binding affinity for glycoproteins. J Biol Chem 2011;286:13801-4. [PubMed]
- Mirandola L, Yu Y, Chui K, et al. Galectin-3C inhibits tumor growth and increases the anticancer activity of bortezomib in a murine model of human multiple myeloma. PLoS One 2011;6:e21811. [PubMed]
- Zick Y, Eisenstein M, Goren RA, et al. Role of galectin-8 as a modulator of cell adhesion and cell growth. Glycoconj J 2004;19:517-26. [PubMed]
- Nishi N, Itoh A, Shoji H, et al. Galectin-8 and galectin-9 are novel substrates for thrombin. Glycobiology 2006;16:15C-20C. [PubMed]
- Mazurek N, Sun YJ, Price JE, et al. Phosphorylation of galectin-3 contributes to malignant transformation of human epithelial cells via modulation of unique sets of genes. Cancer Res 2005;65:10767-75. [PubMed]