Characterization of antibody response against 16kD and 38kD of M. tuberculosis in the assisted diagnosis of active pulmonary tuberculosis
Introduction
Tuberculosis is a chronic infectious disease caused by the Mycobacterium tuberculosis complex (1,2), and has a higher infection rate in children and the elderly (3,4). In recent years, due to global population movements, lax awareness of tuberculosis prevention, the emergence of multidrug-resistant tuberculosis, and the spread of HIV (5-8), tuberculosis has returned to prominence, with its infection rate increasing year by year and seriously threatening human health and public health safety (9-11). However, there is still a lack of simple, rapid, and effective means of tuberculosis diagnosis in clinical practice. Therefore, early, rapid, and accurate diagnosis of tuberculosis is of great significance for the control of a tuberculosis epidemic (12,13).
As a global infectious disease, the chemotherapy of tuberculosis has been facing severe challenges. The chemotherapy of tuberculosis has gradually developed from the single drug in the early stage to the most basic four drug combination treatment in the 1970s. Since the advent of standard short-term chemotherapy, the basic principles of early, combined, appropriate, regular and whole course anti tuberculosis chemotherapy have been running through. Initially-treated sensitive tuberculosis is often cured by standardized anti-tuberculosis treatment. If diagnosed as drug-resistant tuberculosis, especially multidrug-resistant tuberculosis, the difficulty of treatment will increase. With the introduction of some new drugs, the cure rate of drug-resistant tuberculosis has gradually increased. Studies have shown that the success rate of multi-drug-resistant tuberculosis treatment programs containing bedaquiline can reach up to 88% and the cure rate can reach 76%. Nevertheless, the early diagnosis and early treatment of tuberculosis are still the key to the control of the tuberculosis epidemic.
Although etiological detection is the gold standard for tuberculosis diagnosis, it still has many limitations (14), including a low detection rate of acid-fast staining in sputum smears (15,16), long time-consuming isolation and culture of tuberculosis bacteria, expensive nucleic acid detection, high laboratory technical requirements, many atypical manifestations on imaging examination, and difficulty in finding small lesions (17,18). These factors make early diagnosis of tuberculosis a great challenge. The immunological diagnosis of tuberculosis has been studied more in recent years, with the interferon-γ release test (19) demonstrating high sensitivity and specificity, and being widely used in the diagnosis of tuberculosis; however, it is expensive and cannot discern between latent infections, active tuberculosis, and inactive tuberculosis well (20,21).
Diagnostic methods for detecting specific antibodies in patients’ serum have the advantages of low cost, simplicity, and speed, and have been applied in the diagnosis of various diseases (22). At present, the commonly used serological diagnostic method is enzyme-linked immunosorbent assay (ELISA), which can detect specific antigens in serum. The ELISA method is simple and fast, and is widely used in the serological diagnosis of tuberculosis (23-25). Thus far, however, the sensitivity and specificity of the antigens used have been poor and need to be further improved (26-30). The 16kD protein (heat shock protein HspX, Rv2031c), as a small molecule heat shock protein, has strong species specificity, and, as a membrane protein, is anchored on the cell membrane and has strong immunogenicity (31,32). Meanwhile, the 38kD protein (periplasmic phosphate-binding lipoprotein PstS1, RV0934), which is a tuberculosis secretory protein (33), is generally believed to be secreted during infection and is expected to be used to assist in judging the infection status of tuberculosis (29,34).
Therefore, in this study, the secreted protein 38kD and the relatively conserved membrane protein 16kD of M. tuberculosis were used as detection antigens, and the superior antibody detection effect of the novel evolved immunoglobulin-binding molecule (NEIBM)-ELISA method was used (35,36) to detect the serum antibody level of active tuberculosis patients. Through this, we hope to explore the significance of these two antigens in the serological detection of M. tuberculosis, further modify the detection antigen through the follow-up, and ultimately obtain a better detection method of M. tuberculosis serological detection.
The target population selected in this study was patients with active pulmonary tuberculosis, which would be closely related to clinical practice and based on etiological diagnosis. Unlike previous studies that only distinguish active TB patients from healthy people, this study subdivided active tuberculosis patients into two groups of confirmed cases and clinically diagnosed cases. This grouping is closer to the clinical reality, and at the same time is conducive to more accurate analysis of the two antigen and antibody responses of Mycobacterium tuberculosis in different populations. Through this method, our study may provide a solid foundation for the serological diagnosis of M. tuberculosis.
We present the following article in accordance with the MDAR reporting checklist (available at http://dx.doi.org/10.21037/atm-20-5476).
Methods
Ethical statement
All procedures performed in this study involving human participants were in accordance with the Declaration of Helsinki (as revised in 2013). The study was approved by the Ethics Committee of Shanghai Pulmonary Hospital and informed consent was taken from all the patients.
Serum samples
Serum samples from 384 patients with confirmed pulmonary tuberculosis (tuberculosis pathogen test-positive and mycobacterium-positive cases) and 384 patients with clinically diagnosed pulmonary tuberculosis (pathogen test-negative and mycobacterium-negative cases) were randomly collected. All serum samples were collected from inpatients in Shanghai Pulmonary Hospital. All participants in the study had signed written informed consent. The serum information of these patients was shown in Table 1.
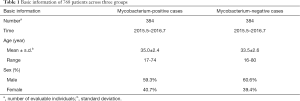
Full table
Experimental proteins and reagents
The ELISA sample diluent was prepared and stored in our laboratory. The prokaryotic expression plasmid pET-32a and the E. coli host strain BL21 (DE3) were purchased from Novagen (Darmstadt, Germany). The detection antibody used in the experiment was horseradish peroxidase (HRP)-labeled LD5 (HRP-LD5) NEIBM, which was independently developed by the Department of Pathogenic Biology, Naval Military Medical University (37). The antibody was constructed by randomly arranging the D domain of Staphylococcal protein A (SpA) and the B3 domain of Peptostreptococcus magnus protein L (PpL). Compared with other commercial antibodies, this antibody has a strong binding ability to human immunoglobulin (Ig)M, IgG, and IgA (35).
Construction of recombine vectors of 16kD and 38kD protein
The amino acid sequences of 16kD and 38kD were obtained from GenBank (GenBank accession number: 6137792266, 14115207). The DNA sequences of 16kD and 38kD were synthesized using sequential OE-PCR as previously described (38). Then the PCR products of 16kD and 38kD were digested with different enzymes, purified and inserted into the cloning sites of the prokaryotic expression vector pET-32a vector under the T7 promoter and a His-tag was added at the N-terminus of the target to form recombinant plasmids. The recombinant expression plasmids were individually verified by sequencing analysis.
Expression and purification of 16kD and 38kD fusion proteins from M. tuberculosis
To begin, 50 L of frozen pET-32a-16kD/BL21 (DE3) and pET-32a-38kD/BL21 (DE3) were transfected into 1 mL of 2-fold YT medium containing 100 g/mL Amp + 100 g/mL Kana antibiotics for overnight culture and screening. Then, 500 L of activated glycerol bacteria were transferred to 50 mL of LB medium for expansion culture. After that, the 50 mL LB culture medium that had been cultured was all transferred into 450 mL of fresh culture medium. Finally, IPTG inducer with a final concentration of 1 mM/L was added into the culture medium, and the bacteria were collected by low-temperature centrifugation; the above bacteria were resuspended with 30 mL of phosphate-buffered saline (PBS) (pH 7.2); the precipitates were lysed overnight with 8 M of urea 4 °C by ultrasonic lysis and centrifuged. The lysed supernatant was further purified by nickel column affinity chromatography Ni-NTA resin (Qiagen, Hilden, Germany), and the purified protein was identified by SDS-PAGE and then subpacked for freezing storage at −80 °C.
Detection of antibody response levels of M. tuberculosis 16kD and 38kD antigens in different populations by NEIBM-ELISA
The prepared fusion proteins of M. tuberculosis 16kD and 38kD were diluted to a final concentration of 1 mg/mL with carbonate buffer. It was then added to a 96-well ELISA plate, coated overnight, and then sealed. The serum samples were diluted 20 times, 100 L was then added to each hole on the corresponding ELISA plate, and incubated in 37 °C incubator for 45 min. The plates were washed three times with ELISA and then dried; sample diluent and HRP-LD5 were mixed at a volume ratio of 1:2,000, and then 100 L of each well was added to the ELISA plate, which was incubated in 37 °C incubator for 45 min; 50 L of color developing solution was added after washing the plate three times, which was left at room temperature for 3 min, color-developed for 7 minutes, and then 50 L of stop solution was added to each well of the ELISA plate to terminate the reaction. These were then put into the ELISA (Thermo Scientific Multiskan Fc, Vantaa, Finland) to determine the OD450 value (36).
After the OD value was read by ELISA, the serum OD value of the normal control group was sorted from large to small, and the average value of 50% was calculated. The OD value of each hole in the control group was divided by this average value to obtain a ratio (S/N), which was regarded as the corrected response value, and analyzed. S/N values greater than 2.5 were considered positive. At the same time, the positive reaction patients were divided into low reactions [2.5–4], moderate reactions [5–10], and high reactions (>10) according to the numerical value.
Statistical analysis
Statistical software SPSS18.0 and SAS 9.0 were used to analyze the differences of serum anti-16kD and anti-38kD antibody reactivity between 384 confirmed cases of M. tuberculosis and 384 clinically diagnosed cases. Chi-square test and independent samples t-test were used for statistical methods, and the difference was considered to be statistically significant when P<0.05.
Results
Analysis of antibody reactivity of M. tuberculosis antigens 16kD and 38kD in bacteria-positive patients
The NEIBM-ELISA method was used to detect the antibody response of 16kD and 38kD antigens of M. tuberculosis in 384 positive patients with M. tuberculosis, as shown in Figure 1. In 384 M. tuberculosis-positive patients, the levels of anti-16kD and anti-38kD antibodies, along with the reaction patterns, were similar. After statistical processing, it was found that there was no significant difference in the level of antibody response detected by the two antigens (Figure 1A). In addition, there was no statistical difference in the positive rate of antibody reaction between 16kD and 38kD antigens of M. tuberculosis alone, but the positive rate of 16kD and 38kD antigens detected together was 61.5%, which was higher than the positive rate of the two antigens detected alone. This suggests that the effect of the combined detection of the two antigens was better than that detected by the 16kD and 38kD antigens alone (Figure 1B).
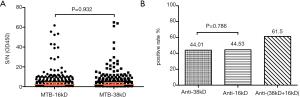
Among the 384 M. tuberculosis-positive patients, the positive rate of M. tuberculosis 16kD antigen detection was 44.5%, including 9.4% in the high-reaction group, 16.4% in the moderate-reaction group, and 18.75% in the low-reaction group (see Table 2). However, the positive rate of M. tuberculosis 38kD antigen detection was 44.01%, including 8.6% in the high-reaction group, 17.4% in the moderate-reaction group, and 18.0% in the low-reaction group (see Table 3). Chi-square test found that there was no significant difference in the detection results of 16kD and 38kD antigens, suggesting that the sensitivity and specificity of using these two antigens to detect serum antibodies in M. tuberculosis-positive patients were similar.
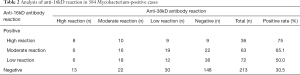
Full table
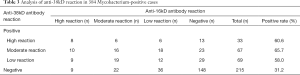
Full table
The results showed that the proportion of serum samples positive for the 16kD antigen test and negative for 38kD antigen test was 17.4%, with the most samples being found in low-reaction group, followed by the moderate- and high-reaction groups (see Tables 2 and 4). The proportion of serum surface specimens positive for 38kD antigen test and negative for 16kD antigen test was 16.9%; with the most samples being found in the low-reaction group, followed by the moderate- and high- reaction groups (see Tables 3 and 4). The relationship between the grouping and reaction levels suggests that the detection results of 16kD and 38kD antigens were similar.

Full table
The proportion of serum specimens positive for both 16kD and 38kD antigens of M. tuberculosis was 27.1%; the group with the best consistency of reaction degree was the low-reaction group, followed by the moderate- and high-reaction groups (see Tables 2-4).
Analysis of antibody reactivity of M. tuberculosis antigens 16kD and 38kD in bacteria-negative patients
The NEIBM-ELISA method was used to detect the antibody response of 16kD and 38kD antigens of M. tuberculosis in 384 Mycobacterium tuberculosis-negative patients, as shown in Figure 2. Among the 384 M. tuberculosis-negative patients, the antibody response levels of anti-16kD and anti-38kD were similar, and the proportion of antibody high-response samples detected by the two antigens was higher than that of the bacterium-positive patients, with no significant difference in the antibody response levels detected by the two antigens (Figure 2A). In addition, there was no statistical difference in the positive rate of antibody reaction between 16kD and 38kD antigens of M. tuberculosis alone, while the positive rate of co-detection of the two antigens was 47.4%, which was higher than that of the two antigens alone, but lower than the 61.5% of the bacterium-positive patients. This suggests that the combined detection of two antigens was better than that of 16kD and 38kD antigens alone, but the detection rate was not high (Figure 2B).
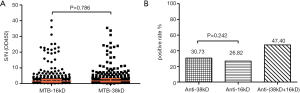
Among the 384 M. tuberculosis-negative patients, the positive rate of M. tuberculosis 16kD antigen detection was 26.8%, including 5.2% in the high-reaction group, 9.6% in the moderate-reaction group, and 12.0% in the low-reaction group (see Table 5). Among the 384 M. tuberculosis-negative patients, the positive rate of M. tuberculosis 38kD antigen detection was 30.7%, including 5.2% in the high-reaction group, 13.0% in the moderate-reaction group, and 12.5% in the low-reaction group (see Table 6). Chi-square test showed that there was no significant difference between the two antigens, which indicates that there was no significant difference between the two antigens in the detection of serum antibodies M. tuberculosis-negative patients.
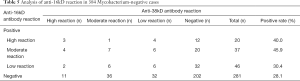
Full table
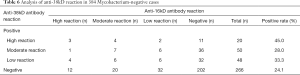
Full table
The proportion of serum samples positive for 16kD antigen test and negative for 38kD antigen test was 16.7%, with the most samples being found in low-reaction group for 16kD antigen, followed by the moderate- and high-reaction groups (see Tables 5 and 7). The proportion of serum samples positive for 38kD antigen test and negative for 16kD antigen test was 20.6%, with the most samples being found in moderate-reaction group, followed by the low- and high-reaction groups (see Tables 6 and 7).

Full table
The results showed that the proportion of serum samples positive for both 16kD and 38kD antigens of M. tuberculosis was 10.1%. The consistency of the degree of response was similar between the low-reaction group and the moderate-reaction group, and slightly worse in the high-reaction group (see Table 7). In addition, the complementarity of 16kD and 38kD antigens in the results of bacterial-negative patients was superior to that of bacterial-positive patients, which further suggests that the combination of 16kD and 38kD could be applied to the serological detection of M. tuberculosis infection.
Discussion
Tuberculosis caused by a complex dominated by M. tuberculosis is a chronic infectious disease. Traditional etiological methods, including sputum smear microscopy, along with M. tuberculosis isolation and culture, cannot be used for rapid and effective diagnosis. Although the sensitivity and specificity of the interferon-gamma release test in immunodiagnosis are high, this test is expensive and cannot distinguish between latent infections, active tuberculosis, and inactive tuberculosis well. Therefore, finding a rapid and simple diagnostic method that can be applied in clinic is an important for current diagnostic research of M. tuberculosis (12).
The ELISA method is widely used in serological diagnosis of tuberculosis due to its speed and simplicity in operation. Thus far, however, the sensitivity and specificity of the antigens used have been poor, and require improvement. In this study, the secreted protein 38kD and the relatively conserved membrane protein 16kD of M. tuberculosis were used as detection antigens, and the NEIBM-ELISA method, with its superior antibody detection effect, was used to detect the serum antibody level of active tuberculosis patients. The aim of the research was to explore the significance of these two antigens in the serological detection of M. tuberculosis, and to further modify the detection antigen through the follow-up, so as to create a better serological detection method for M. tuberculosis.
The target population selected in this study was patients with active pulmonary tuberculosis, which would be closely related to clinical practice and based on etiological diagnosis. Unlike previous studies that only distinguish tuberculosis patients from healthy people, this study subdivided active tuberculosis patients into two groups of confirmed cases and clinically diagnosed cases for analysis. This grouping more closely approximates the clinical reality, while being more conducive to a more accurate analysis of the two antigens and the antibody reaction of M. tuberculosis in different populations. Through this method, our study may provide a solid foundation for the serological diagnosis of M. tuberculosis.
The 16kD and 38kD antigens of M. tuberculosis were used to detect the corresponding antibody levels in the serum of confirmed cases of tuberculosis, and it was found that the positive detection rates of these two antigens in confirmed cases of tuberculosis were higher than those in the clinically diagnosed cases (about 44%). This indicates that the tuberculosis of confirmed cases might have been precipitated by M. tuberculosis infection. Meanwhile, there was no significant difference in the positive detection rate between the two antigens, suggesting that the detection effect of these two antigens in confirmed cases of tuberculosis is similar and needs to be further improved. We combined the detection of the 16kD 38kD antigens and found that the positive detection rate could be increased to 61.5%, which was significantly better than the detection effect of the two antigens alone. We can therefore speculate that the combined detection of multiple antigens for serological diagnosis of infection can be effectively used in clinical practice.
The positive detection rate of 16kD and 38kD antigens was 26–30% in the clinical diagnosis cases of tuberculosis, which was lower than those in the confirmed cases of tuberculosis. There was no significant difference in the positive detection rates of the two antigens, suggesting that the detection effects of 16kD and 38kD antigens were not significantly different in tuberculosis diagnosis cases. When the two antigens are combined, the detection effect is better than that of 16kD and 38kD antigens alone, but the detection rate is still lower than that of confirmed cases of tuberculosis, suggesting that the level of anti-tuberculosis immune response in confirmed cases may be higher than that in clinically diagnosed cases. On the one hand, a strong immune response can reflect more serious disease, especially if the proportion of pulmonary cavity cases in confirmed cases is larger than that in clinically diagnosed cases. This may indicate that the immune damage of pulmonary cavity patients is stronger, as the body is more likely to discharge necrotic tissues and tuberculosis bacteria after the formation of pulmonary cavity, and tuberculosis bacteria are more easily detected. Furthermore, because there is no gold standard for bacteriological diagnosis in clinical diagnosis cases, there may be some cases that are not tuberculosis infection. Both imaging and disease characteristics are very similar to mycobacterium tuberculosis infection, which makes it easy to diagnose non-tuberculosis patients as tuberculosis patients clinically, and this part of cases cannot be verified. However, these cases could not be verified, but the lower antibody levels in clinically diagnosed cases than in confirmed cases further suggests this possibility. It also suggests that our experimental results were true, credible, and reasonable.
In this study, the NEIBM-ELISA method was used to detect the antibody response level of M. tuberculosis antigens, 16kD and 38kD, in both confirmed and clinically diagnosed tuberculosis cases. The detection efficiency in both confirmed and clinically confirmed cases was higher than that of single detection, which is consistent with some studies that improved the diagnosis efficiency through the combination of multiple antigens (27,39,40). However, it is far from meeting the level of clinical application, and the detection rate still needs to be further improved. We will continue to modify these two antigens and search for other antigens of M. tuberculosis. We hope to improve the sensitivity and specificity of serological detection of M. tuberculosis through combined detection of different antigens, and to provide basic immunological information for further research on the immune response to tuberculosis, immunological diagnosis, and vaccine development.
Conclusions
In this study, the NEIBM-ELISA method was used to detect the antibody response of M. tuberculosis antigens, 16kD and 38kD, in both confirmed and clinically diagnosed tuberculosis cases. We found that the positive rate of combined detection of 16kD and 38kD antigens of M. tuberculosis was higher than that of single detection in two cases, but it was not a simple addition. We can thus conclude that there is complementarity between the two antigens and overlap in some cases, which requires a further and more detailed study of clinical cases. It is suggested that the combined detection of multiple antigens can be used in the serological diagnosis of mycobacterium tuberculosis infection.
Acknowledgments
Funding: This work was supported by of the Multi-Dimensional Diagnostic Strategies for Tuberculosis and Research and Development of Innovative Products Program (No. 2018ZX10302301).
Footnote
Reporting Checklist: The authors have completed the MDAR reporting checklist. Available at http://dx.doi.org/10.21037/atm-20-5476
Data Sharing Statement: Available at http://dx.doi.org/10.21037/atm-20-5476
Conflicts of Interest: All authors have completed the ICMJE uniform disclosure form (available at http://dx.doi.org/10.21037/atm-20-5476). The authors have no conflicts of interest to declare.
Ethical Statement: The authors are accountable for all aspects of the work in ensuring that questions related to the accuracy or integrity of any part of the work are appropriately investigated and resolved. All procedures performed in this study involving human participants were in accordance with the Declaration of Helsinki (as revised in 2013). The study was approved by the Ethics Committee of Shanghai Pulmonary Hospital (approval ID: K19-062Y) and informed consent was taken from all the patients.
Open Access Statement: This is an Open Access article distributed in accordance with the Creative Commons Attribution-NonCommercial-NoDerivs 4.0 International License (CC BY-NC-ND 4.0), which permits the non-commercial replication and distribution of the article with the strict proviso that no changes or edits are made and the original work is properly cited (including links to both the formal publication through the relevant DOI and the license). See: https://creativecommons.org/licenses/by-nc-nd/4.0/.
References
- Lawn SD, Zumla AI. Tuberculosis. Lancet 2011;378:57-72. [Crossref] [PubMed]
- Spitaleri A, Ghodousi A, Miotto P, et al. Whole genome sequencing in Mycobacterium tuberculosis. Ann Transl Med 2019;7:S197. [Crossref] [PubMed]
- Esmail A, Sabur NF, Okpechi I, et al. Management of drug-resistant tuberculosis in special sub-populations including those with HIV co-infection, pregnancy, diabetes, organ-specific dysfunction, and in the critically ill. J Thorac Dis 2018;10:3102-18. [Crossref] [PubMed]
- Tahan TT, Gabardo BMA, Rossoni AMO. Tuberculosis in childhood and adolescence: a view from different perspectives. J Pediatr (Rio J) 2020;96 Suppl 1:99-110. [Crossref] [PubMed]
- Richeldi L, Ewer K, Losi M, et al. Early diagnosis of subclinical multidrug-resistant tuberculosis. Ann Intern Med 2004;140:709-13. [Crossref] [PubMed]
- Gandhi NR, Nunn P, Dheda K, et al. Multidrug-resistant and extensively drug-resistant tuberculosis: a threat to global control of tuberculosis. Lancet 2010;375:1830-43. [Crossref] [PubMed]
- Bell LCK, Noursadeghi M. Pathogenesis of HIV-1 and Mycobacterium tuberculosis co-infection. Nat Rev Microbiol 2018;16:80-90. [Crossref] [PubMed]
- Dorman SE. Diagnosis of HIV-associated tuberculosis. Curr Opin HIV AIDS 2018;13:462-8. [Crossref] [PubMed]
- Global tuberculosis report 2019. Geneva: World Health Organization, 2019.
- Glaziou P, Floyd K, Raviglione MC. Global Epidemiology of Tuberculosis. Semin Respir Crit Care Med 2018;39:271-85. [Crossref] [PubMed]
- MacNeil A, Glaziou P, Sismanidis C, et al. Global Epidemiology of Tuberculosis and Progress Toward Meeting Global Targets - Worldwide, 2018. MMWR Morb Mortal Wkly Rep 2020;69:281-5. [Crossref] [PubMed]
- Viñuelas-Bayón J, Vitoria MA, Samper S. Rapid diagnosis of tuberculosis. Detection of drug resistance mechanisms. Enferm Infecc Microbiol Clin 2017;35:520-8. [Crossref] [PubMed]
- Helb D, Jones M, Story E, et al. Rapid Detection of Mycobacterium tuberculosis and Rifampin Resistance by Use of On-Demand, Near-Patient Technology. J Clin Microbiol 2010;48:229-37. [Crossref] [PubMed]
- Nahid P, Pai M, Hopewell PC. Advances in the diagnosis and treatment of tuberculosis. Proc Am Thorac Soc 2006;3:103-10. [Crossref] [PubMed]
- Siddiqi K, Lambert ML, Walley J. Clinical diagnosis of smear-negative pulmonary tuberculosis in low-income countries: The current evidence. Lancet Infect Dis 2003;3:288-96. [Crossref] [PubMed]
- Steingart KR, Ng V, Henry M, et al. Sputum processing methods to improve the sensitivity of smear microscopy for tuberculosis: a systematic review. Lancet Infect Dis 2006;6:664-74. [Crossref] [PubMed]
- Kulkarni S, Jha S. Artificial Intelligence, Radiology, and Tuberculosis: A Review. Acad Radiol 2020;27:71-5. [Crossref] [PubMed]
- Piccazzo R, Paparo F, Garlaschi G. Diagnostic accuracy of chest radiography for the diagnosis of tuberculosis (TB) and its role in the detection of latent TB infection: a systematic review. J Rheumatol Suppl 2014;91:32-40. [Crossref] [PubMed]
- Lim WS. From latent to active TB: are IGRAs of any use? Thorax 2016;71:585-6. [Crossref] [PubMed]
- Browatzki A, Meyer CN. Interferon-gamma release assay on suspicion of active tuberculosis? Ugeskr Laeger 2009;171:2631-5. [PubMed]
- Papay P, Eser A, Winkler S, et al. Predictors of indeterminate IFN-gamma release assay in screening for latent TB in inflammatory bowel diseases. Eur J Clin Invest 2011;41:1071-6. [Crossref] [PubMed]
- Abebe F, Holm-Hansen C, Wiker HG, et al. Progress in serodiagnosis of Mycobacterium tuberculosis infection. Scand J Immunol 2007;66:176-91. [Crossref] [PubMed]
- Steingart KR, Megan H, Suman L, et al. Commercial serological antibody detection tests for the diagnosis of pulmonary tuberculosis: a systematic review. PLoS Med 2007;4:e202. [Crossref] [PubMed]
- Chan ED, Heifets L, Iseman MD. Immunologic diagnosis of tuberculosis: a review. Tuber Lung Dis 2000;80:131-40. [Crossref] [PubMed]
- Zhang L, Wang Q, Wang W, et al. Identification of putative biomarkers for the serodiagnosis of drug-resistant Mycobacterium tuberculosis. Proteome Sci 2012;10:12. [Crossref] [PubMed]
- Pukazhvanthen P, Anbarasu D, Kabeer Basirudeen SA, et al. Assessing humoral immune response of 4 recombinant antigens for serodiagnosis of tuberculosis. Tuberculosis 2014;94:622-33. [Crossref] [PubMed]
- Zhang SL, Zhao JW, Sun ZQ, et al. Development and evaluation of a novel multiple-antigen ELISA for serodiagnosis of tuberculosis. Tuberculosis (Edinb) 2009;89:278-84. [Crossref] [PubMed]
- Cockle PJ, Gordon SV, Lalvani A, et al. Identification of novel Mycobacterium tuberculosis antigens with potential as diagnostic reagents or subunit vaccine candidates by comparative genomics. Infect Immun 2002;70:6996-7003. [Crossref] [PubMed]
- Målen H, Softeland T, Wiker HG. Antigen analysis of Mycobacterium tuberculosis H37Rv culture filtrate proteins. Scand J Immunol 2008;67:245-52. [Crossref] [PubMed]
- Wang S, Wu J, Chen J, et al. Evaluation of Mycobacterium tuberculosis-specific antibody responses for the discrimination of active and latent tuberculosis infection. Int J Infect Dis 2018;70:1-9. [Crossref] [PubMed]
- Imaz MS, Comini MA, Zerbini E, et al. Evaluation of the diagnostic value of measuring IgG, IgM and IgA antibodies to the recombinant 16-kilodalton antigen of mycobacterium tuberculosis in childhood tuberculosis. Int J Tuberc Lung Dis 2001;5:1036-43. [PubMed]
- Jee B, Singh Y, Yadav R, et al. Small Heat Shock Protein16.3 of Mycobacterium tuberculosis: After Two Decades of Functional Characterization. Cell Physiol Biochem 2018;49:368-80. [Crossref] [PubMed]
- Zhou F, Xu X, Wu S, et al. Protein array identification of protein markers for serodiagnosis of Mycobacterium tuberculosis infection. Sci Rep 2015;5:15349. [Crossref] [PubMed]
- Wu X, Yang Y, Zhang J, et al. Comparison of antibody responses to seventeen antigens from Mycobacterium tuberculosis. Clin Chim Acta 2010;411:1520-8. [Crossref] [PubMed]
- Cao J, Chen Q, Zhang H, et al. Novel evolved immunoglobulin (Ig)-binding molecules enhance the detection of IgM against hepatitis C virus. PLoS One 2011;6:e18477. [Crossref] [PubMed]
- Ding Y, Chen X, Qian B, et al. Characterization of the antibody response against EV71 capsid proteins in Chinese individuals by NEIBM-ELISA. Sci Rep 2015;5:10636. [Crossref] [PubMed]
- Jiang SH, Wang JF, Xu R, et al. Alternate arrangement of PpL B3 domain and SpA D domain creates synergistic double-site binding to VH3 and Vkappa regions of fab. DNA Cell Biol 2008;27:423-31. [Crossref] [PubMed]
- Zhang P, Ding Y, Liao W, et al. A simple, universal, efficient PCR-based gene synthesis method: sequential OE-PCR gene synthesis. Gene 2013;524:347-54. [Crossref] [PubMed]
- Feng X, Xiu B, Chen K, et al. Enhanced serodiagnostic utility of novel Mycobacterium tuberculosis polyproteins. J Infect 2013;66:366-75. [Crossref] [PubMed]
- Kumar G, Dagur PK, Singh M, et al. Diagnostic potential of Ag85C in comparison to various secretory antigens for childhood tuberculosis. Scand J Immunol 2008;68:177-83. [Crossref] [PubMed]
(English Language Editor: J. Gray)