Measurements of deposited aerosol dose in infants and small children
Introduction
Pediatric patients with lung disease depend on inhaled medications. This includes bronchodilators and corticosteroids for asthma and childhood wheeze, racemic epinephrine for croup, and antibiotics, hypertonic saline, and mucolytics for cystic fibrosis (CF). These medications are delivered from metered dose inhalers (MDIs), dry powder inhalers (DPIs), and medical nebulizers. Aerosol deposition can vary significantly between children and adults based on differences in airway size and respiratory parameters. Children also vary in their ability to cooperate with treatments and may require delivery system adjuncts such as masks or nasal cannulas. These factors can lead to significant dose variability and potentially affect the efficacy of inhaled therapies. Here we will discuss the techniques used to study aerosol dosing in vivo, review available studies that have measured aerosol dose in children, discuss the factors that adversely affect aerosol dosing for children, and consider potential methods for improving aerosol drug delivery to infants and children.
Techniques for studying aerosol dosing in children
Deposition scintigraphy techniques are used to measure the dose of aerosol medication delivered to the lungs during inhaled therapy treatments (1-3). These studies utilize a radiopharmaceutical that is directly or indirectly labeled to the active drug component of the inhaled medication. Pre-clinical studies are performed to establish a labeling method that provides a direct relationship between radioactive counts emitted by the radiopharmaceutical and the active drug dose. Once this relationship is validated across different aerosol size classes (4), radiation can be used as a quantifiable analog of drug dose. It can easily be measured in aerosol delivery systems and leakage/exhalation filters before and after administration to determine total delivered dose [An example of the use of this “mass balance” technique pediatric subjects can be found in (5)]. With the incorporation of gamma camera imaging, radiation measurements can also be used to quantify deposited dose within the mouth, upper airways, lungs, esophagus, and stomach, using 2D or 3D imaging. These studies can be performed with nebulizers (6), metered dose inhalers (7), dry powder inhalers (8), and condensate aerosol delivery systems (such as electronic cigarettes) (9).
These techniques have been applied less frequently in children and infants, largely based on the radiation exposure associated with their use. However, the use of careful technique can limit radiation exposure, and good images can be attained with deposited lung doses in the range of 1.5 MBq (40–50 µCi) using Technetium 99m based compounds. Technetium 99m has a relatively short half-life (~6 hours) and is available forms that are readily cleared from the body. The risk to benefit ratio associated with this exposure may be favorable in pediatric populations that are significantly dependent on inhaled medications, where patient-specific or generalizable knowledge on dosing is important to improving clinical care. Nuclear medicine techniques are commonly performed in children, including infants, and dosimetry tables for estimating inhaled exposures are available (10).
Aerosol deposition studies in infants and children
Nebulizers
O’Doherty et al. measured the lung doses of nebulized pentamidine delivered to 12 children with HIV. The percent of the nebulized dose deposited in the lungs was 5.5%±2.4% for children ages 8–11, 7.2%±2.2% for ages 12–15, and 7.1%±2.6% for adults (± SD) (11).
Chua et al. measured aerosol deposition from medical nebulizers in 12 infants and 8 children with cystic fibrosis. The 12 infants ranged in age from 0.3–1.4 years old. All were anesthetized during aerosol delivery and a mask was utilized. In the infants, deposited lung doses ranged from 0.3–2.1% of nebulized dose. Deposited head/upper airway doses ranged from 38.8–84.7%. Lung doses after oral inhalation in children from 6–12 years old ranged from 5.3–9.1%. Upper airway doses in that group ranged from 30.1–49.4% (12).
Mallol et al. studied aerosol deposition in 20 infants with cystic fibrosis considering the use of different sized nebulized aerosols and sedation vs. no sedation. Non-sedated infants inhaling a 7.7 µm aerosol deposited 0.76%±0.36% (n=5) of the loaded dose in their lungs vs. 2.0%±0.71% in a group of infants inhaling a 3.6 µm aerosol (P<0.01, ±SD). Oropharyngeal deposition was 6.78%±2.72% vs. 2.4%±0.83% in the same groups (P<0.05). Sedation did not significantly affect aerosol deposition (13).
Fauroux et al. compared the delivery of nebulized aerosols with and without pressure support in children and adults with cystic fibrosis, ages 6–21. Lung deposition was 15.3%±8.3% (of nebulized dose) with pressure support vs. 11.5%±5.7% without (P<0.05 through a paired comparison). When considered based on percentage of loaded dose: 2.4%±1.5% vs. 1.7%±1.2% (P=0.01) (14).
Schueepp et al. measured aerosol deposition in 10 asthmatic children, ages 0.5–3 years using budesonide with Technetium 99m-DTPA added. The solution was delivered using a vibrating mesh nebulizer and a facemask. Lung deposition varied from 8–56.4% of emitted dose. Oropharyngeal dose (including facial deposition) varied from 43.6–92.0%. The study did not differentiate dose on the face from dose in the mouth (15).
Amirav et al. measured aerosol deposition in 12 infants (<12 months old) comparing delivery with a nebulizer and mask to a nebulizer + mask + pacifier combination. Mean right lung deposition (± SD) using a mask with attached pacifier was 1.6%±0.5% which was similar to deposition with a conventional mask: 1.7%±0.9%, P=0.81. Stomach doses were 1.2%±1.2% and 2.0%±1.9% respectively. Upper airway doses were 3.7%±1.3% and 2.0%±1.9% (16).
Corcoran et al. measured the distribution of nebulized Technetium 99m sulfur colloid aerosols delivered by nasal cannula to 18 infants with congenital heart defects who were performing research mucociliary clearance scans. Increases in the percentage of the drug delivered to the lungs vs. the nasopharynx were achieved using lower nasal cannula air flowrates (0.2 L/min). The fraction of the deposited in the lungs was 33.5%±13% at 0.2 L/min vs. 4.5% ±2.2% at 2 L/min of cannula flow (± SD). The remaining fraction of the deposited dose was found in the nasopharynx (17).
Metered dose inhalers
Tal et al. measured the dose of Technetium 99m labeled salbutamol delivered by metered dose inhaler and deposited in the lungs of 15 infants and children with asthma, cystic fibrosis, or bronchopulmonary dysplasia. The average deposited dose was 1.97%±1.4% in the lungs (% of emitted dose ± SD), 1.28%±0.77% in the oropharynx, and 1.11%±2.4% in the stomach. The remainder of the dose was lost in the spacer (18).
Devadason et al. measured the deposition of Technetium 99m-labelled beclomethasone HFA (QVARTM) delivered from a breath-actuated metered dose inhaled (AutohalerTM) to 16 children ages 5–14 with mild asthma. Lung deposition was 36.9%±9.2% (% of emitted dose ± SD) in 5–7 years old children vs. 46.5%±11.6% in ages 8–10 and 54.1%±10.7% in ages 11–14. Oropharyngeal + GI deposition was 59.7%±8.2%, 48.9%±12.3%, and 40.3%±11.8% for the respective age groups. They demonstrated that lung dose increased with FEV1 and FVC and that mouth and throat deposition decreased with FEV1, FVC, height and age. Lung doses in older children (11–14 years) were similar to reported doses from adults (5).
Roller et al. also measured the deposition of Technetium 99m-labelled beclomethasone HFA (QVARTM), delivered from a metered dose inhaler plus spacer to 24 children with asthma. The authors reported a median size of the aerosol of 1.1 µm. Half of the group inhaled the drug using 5 tidal breaths while the other half used slow inhalations followed by a breath hold. Lung deposition in the tidal breathing group was 35.4%±18.3% (% of emitted dose ± SD) in 5–7 years old children vs. 47.5%±13.0% in ages 8–10, and 54.9%±11.2% in ages 11–17. For the same age groups using slow inhalations and breath holds, lung deposition was 58.1%±6.7%, 56.6%±5.2% and 58.4%±9.2%. Oropharyngeal + GI deposition was 24.0%±10.5%, 10.3%±4.4%, and 10.1%±6.2% using tidal breathing, vs. 12.9%±3.2%, 20.1%±9.5%, and 20.8%±8.8% with slow inhalations and breath holds. Spacer losses were 40.2%±9.2%, 41.5%±15.1%, and 30.7%±11.5% with tidal breathing vs. 24.1%±7.0%, 18.2%±1.6%, and 20.3%±4.5% with slow inhalations and breath holds (19).
Erzinger et al. measured the deposition of salbutamol aerosols from a metered dose inhaler and a nebulizer in recurrently wheezy children. All delivery was done by facemask. Pulmonary deposition (% of loaded dose ± SD) was 7.4% in 31 male subjects using the metered dose inhaler vs. 8.2% in 36 male subjects using the nebulizer. In these same subjects, GI deposition was 12.2% vs. 10.1%, deposition on the face was 5.2% vs. 3.6%, and mask losses were 4.4% vs. 0.8%. Any amount of face mask leak or screaming by the children caused significant decreases in pulmonary dose (20).
Fok et al. compared drug delivery from nebulizers and metered dose inhalers in ventilated and non-ventilated infants with bronchopulmonary dysplasia (BPD). Delivery was via facemask (nebulizer) or facemask + spacer (MDI). Delivery for the ventilated infants was done in line. Lung dose in the non-ventilated subjects was 0.67%±0.17% for the MDI and 1.74%±0.21% for the nebulizer (% of actuated dose/% of nebulized dose, ± SEM). In ventilated infants: 0.98%±0.19% (MDI) and 0.95%±0.23% (nebulizer) (21).
Factors adversely affecting aerosol dosing to pediatric patients
Aerosol mechanics in the pediatric airway
Impaction and sedimentation are the primary mechanisms that cause aerosol deposition in the airways and the lung. Impaction occurs when aerosol droplets acquire too much momentum to follow airflows through changes in direction or velocity and instead move in straight line paths that cause them to collide with surfaces. The tendency for impaction increases with aerosol density and size and airflow velocity and decreases with air viscosity and the size of the airway. These factors can be collected into a single dimensionless quantity called the Stokes number which considers the ratio of the inertial force of the aerosol to the viscous steering force of the airflow:
Where ρ is the density of the aerosol, d is aerosol diameter, V is air velocity, µ is air viscosity, and D is airway diameter. A slip correction factor may be added to the numerator when applied with very small aerosols (22). High Stokes numbers are associated with increases in inertial aerosol deposition, especially in regions with high air velocities where flows change direction (back of the mouth, carina, upper airway bifurcations, etc.). Table 1 shows the estimated Stokes numbers for 5 and 3 µm aerosols based on the respiratory characteristics and airway diameters of adults and children of different ages. It demonstrates the trend towards higher Stokes numbers in pediatric subjects which are likely to be associated with higher levels of mouth, throat, and upper airway deposition.
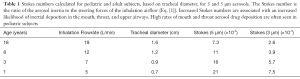
Full table
High rates of mouth and throat aerosol drug deposition have often been demonstrated in pediatric subjects. Figure 1 shows an aerosol deposition scintigraphy image that depicts deposited aerosol in the mouth, lungs, and stomach of an 8-year-old child after a nebulizer treatment. The high levels of stomach activity are associated with aerosol dose deposited in the mouth that has been subsequently swallowed. These effects are likely to explain increased aerosol losses in the mouths and throats of pediatric patients when compared to adult subject.
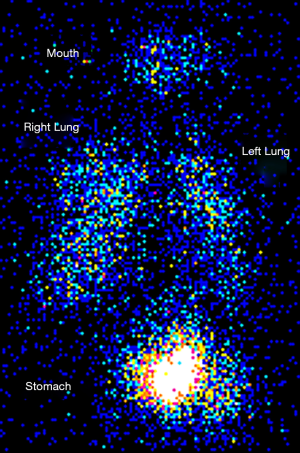
Interfaces
Facemasks are often used to deliver aerosols to infants and younger children. Losses to the environment, within the mask, and onto the patient’s face are inherent with the use of these devices. These losses can contribute to low dosing efficiency and significant variability in dosing. In vitro studies using the Sophia Anatomical Infant Nose-Throat or SAINT model demonstrated deposited lung doses of 7.17%±0.01% vs. 11.42%±0.01% losses in the mask and 71.99%±0.02% losses to the environment (23). The design of the mask and the quality of the seal will significantly affect performance (24-26).
Nasal cannula systems have been considered for aerosol delivery to infants. Nasal cannulas are often used in the pediatric critical care with high gas flowrates to provide oxygenation and potentially airway support as well. Several in vitro studies have demonstrated the viability of delivering aerosol through cannulas (27,28), however, several in vivo studies have demonstrated high rates of deposition within the nose when these systems are used that limit pulmonary delivery (17,29). Nasal deposition may be caused by direct impaction of the aerosol at the nasal valve or by the accumulation of aerosol-laden gas within the nasal cavity. Accumulation occurs when the rate of cannula gas delivery exceeds the minute volume being drawn into the lungs and results in aerosol deposition throughout the nasal cavity which has extensive internal surface area. This deposition can be limited by using decreased cannula flowrates (17,30). However, these flows are well below those used to provide oxygenation.
Cooperation
Child distress and the level of cooperation provided during the treatment can significantly affect the dose delivered during inhaled therapy. Crying and screaming have both been shown to significantly decrease dosing (15,20). As children become older and begin self-administration, the child’s level of attention to the treatment must also be considered, especially for longer nebulizer treatments. Frequent withdraw of the nebulizer from the mouth or nose breathing will limit dose.
Improving aerosol delivery to pediatric subjects
There are significant opportunities to improve inhaled drug delivery to pediatric subjects. High rates of oropharyngeal deposition could potentially be controlled using smaller or less-dense aerosols. Some groups are exploring the use of hygroscopic aerosols that are very small when delivered through the nose but grow within the humid environment of the lungs, allowing for effective sedimentation and deposition in target lung zones (31,32). Efficiency might also be further increased through the use of controlled breathing patterns that limit inhalation velocity, increase tidal volume, deliver aerosol only during specific portions of the breath (bolus delivery), or incorporate slight breath holds (33-35). Incorporation of such controls will be more feasible as more “smart” devices with onboard processors become more available (36). Such devices may also be useful for better engaging children during aerosol therapies through games or other interventions in order to maintain focus and optimize delivery. Previous in vitro studies provide equations that can be used to predict pediatric upper airway deposition and the relative effects of changes in delivery system design (37). Empirical correlations for predicting airway size by age are available (38). Experimental apparatuses for pre-clinical testing of aerosols intended for children have been designed and made available (39). Nasal cannula designs specifically for aerosol delivery to infants have been described and tested using computational fluid dynamics (31). In general, there is a need for the design of aerosol delivery devices specifically for infant and pediatric populations. Such designs would facilitate more efficient delivery of existing therapies and allow for the development of new more dose critical therapies through the inhaled route.
Acknowledgments
Funding: None.
Footnote
Provenance and Peer Review: This article was commissioned by the Guest Editors (Dr. James B. Fink and Dr. Zhe Luo) for the series “Medical Aerosol in Acute and Critical Care” published in Annals of Translational Medicine. The article was sent for external peer review organized by the Guest Editors and the editorial office.
Conflicts of Interest: The author has completed the ICMJE uniform disclosure form (available at http://dx.doi.org/10.21037/atm-20-2045). The series “Medical Aerosol in Acute and Critical Care” was commissioned by the editorial office without any funding or sponsorship. The author has no other conflicts of interest to declare.
Ethical Statement: The author is accountable for all aspects of the work in ensuring that questions related to the accuracy or integrity of any part of the work are appropriately investigated and resolved. The University of Pittsburgh Institutional Review Board approved the study that collected the image depicted in
Open Access Statement: This is an Open Access article distributed in accordance with the Creative Commons Attribution-NonCommercial-NoDerivs 4.0 International License (CC BY-NC-ND 4.0), which permits the non-commercial replication and distribution of the article with the strict proviso that no changes or edits are made and the original work is properly cited (including links to both the formal publication through the relevant DOI and the license). See: https://creativecommons.org/licenses/by-nc-nd/4.0/.
References
- Fleming J, Bailey DL, Chan HK, et al. Standardization of techniques for using single-photon emission computed tomography (SPECT) for aerosol deposition assessment of orally inhaled products. J Aerosol Med Pulm Drug Deliv 2012;25:S29-51. [Crossref] [PubMed]
- Newman S, Bennett WD, Biddiscombe M, et al. Standardization of techniques for using planar (2D) imaging for aerosol deposition assessment of orally inhaled products. J Aerosol Med Pulm Drug Deliv 2012;25:S10-28. [Crossref] [PubMed]
- Corcoran TE. Imaging in Aerosol Medicine. Respir Care 2015;60:850-5; discussion 855-7. [Crossref] [PubMed]
- Devadason SG, Chan HK, Haeussermann S, et al. Validation of radiolabeling of drug formulations for aerosol deposition assessment of orally inhaled products. J Aerosol Med Pulm Drug Deliv 2012;25:S6-9. [Crossref] [PubMed]
- Devadason SG, Huang T, Walker S, et al. Distribution of technetium-99m-labelled QVAR delivered using an Autohaler device in children. Eur Respir J 2003;21:1007-11. [Crossref] [PubMed]
- Corcoran TE, Niven R, Verret W, et al. Lung deposition and pharmacokinetics of nebulized cyclosporine in lung transplant patients. J Aerosol Med Pulm Drug Deliv 2014;27:178-84. [Crossref] [PubMed]
- Ditcham W, Murdzoska J, Zhang G, et al. Lung deposition of 99mTc-radiolabeled albuterol delivered through a pressurized metered dose inhaler and spacer with facemask or mouthpiece in children with asthma. J Aerosol Med Pulm Drug Deliv 2014;27:S63-75. [Crossref] [PubMed]
- Virchow JC, Poli G, Herpich C, et al. Lung Deposition of the Dry Powder Fixed Combination Beclometasone Dipropionate Plus Formoterol Fumarate Using NEXThaler((R)) Device in Healthy Subjects, Asthmatic Patients, and COPD Patients. J Aerosol Med Pulm Drug Deliv 2018;31:269-80. [Crossref] [PubMed]
- Holbrook LT, Zeman KL, Burke A, et al. Radiolabeling an Electronic Cigarette Aerosol Using Technetium Carbon Ultrafine Particles. J Aerosol Med Pulm Drug Deliv 2019;32:47-53. [Crossref] [PubMed]
- . Radiation dose to patients from radiopharmaceuticals. A report of a Task Group of Committee 2 of the International Commission on Radiological Protection. Ann ICRP 1987;18:1-377. [Crossref] [PubMed]
- O'Doherty MJ, Thomas SH, Gibb D, et al. Lung deposition of nebulised pentamidine in children. Thorax 1993;48:220-6. [Crossref] [PubMed]
- Chua HL, Collis GG, Newbury AM, et al. The influence of age on aerosol deposition in children with cystic fibrosis. Eur Respir J 1994;7:2185-91. [Crossref] [PubMed]
- Mallol J, Rattray S, Walker G, et al. Aerosol deposition in infants with cystic fibrosis. Pediatr Pulmonol 1996;21:276-81. [Crossref] [PubMed]
- Fauroux B, Itti E, Pigeot J, et al. Optimization of aerosol deposition by pressure support in children with cystic fibrosis: an experimental and clinical study. Am J Respir Crit Care Med 2000;162:2265-71. [Crossref] [PubMed]
- Schueepp KG, Devadason SG, Roller C, et al. Aerosol delivery of nebulised budesonide in young children with asthma. Respir Med 2009;103:1738-45. [Crossref] [PubMed]
- Amirav I, Luder A, Chleechel A, et al. Lung aerosol deposition in suckling infants. Arch Dis Child 2012;97:497-501. [Crossref] [PubMed]
- Corcoran TE, Saville A, Adams PS, et al. Deposition studies of aerosol delivery by nasal cannula to infants. Pediatr Pulmonol 2019;54:1319-25. [Crossref] [PubMed]
- Tal A, Golan H, Grauer N, et al. Deposition pattern of radiolabeled salbutamol inhaled from a metered-dose inhaler by means of a spacer with mask in young children with airway obstruction. J Pediatr 1996;128:479-84. [Crossref] [PubMed]
- Roller CM, Zhang G, Troedson RG, et al. Spacer inhalation technique and deposition of extrafine aerosol in asthmatic children. Eur Respir J 2007;29:299-306. [Crossref] [PubMed]
- Erzinger S, Schueepp KG, Brooks-Wildhaber J, et al. Facemasks and aerosol delivery in vivo. J Aerosol Med 2007;20:S78-83; discussion S83-4. [Crossref] [PubMed]
- Fok TF, Monkman S, Dolovich M, et al. Efficiency of aerosol medication delivery from a metered dose inhaler versus jet nebulizer in infants with bronchopulmonary dysplasia. Pediatr Pulmonol 1996;21:301-9. [Crossref] [PubMed]
- Das P, Nof E, Amirav I, et al. Targeting inhaled aerosol delivery to upper airways in children: Insight from computational fluid dynamics (CFD). PLoS One 2018;13:e0207711 [Crossref] [PubMed]
- Laube BL, Sharpless G, Shermer C, et al. Deposition of albuterol aerosol generated by pneumatic nebulizer in the Sophia Anatomical Infant Nose-Throat (SAINT) model. Pharm Res 2010;27:1722-9. [Crossref] [PubMed]
- Lin HL, Restrepo RD, Gardenhire DS, et al. Effect of face mask design on inhaled mass of nebulized albuterol, using a pediatric breathing model. Respir Care 2007;52:1021-6. [PubMed]
- Amirav I, Luder AS, Halamish A, et al. Design of aerosol face masks for children using computerized 3D face analysis. J Aerosol Med Pulm Drug Deliv 2014;27:272-8. [Crossref] [PubMed]
- Smaldone GC, Sangwan S, Shah A. Facemask design, facial deposition, and delivered dose of nebulized aerosols. J Aerosol Med 2007;20:S66-75; discussion S75-7. [Crossref] [PubMed]
- Bhashyam AR, Wolf MT, Marcinkowski AL, et al. Aerosol delivery through nasal cannulas: an in vitro study. J Aerosol Med Pulm Drug Deliv 2008;21:181-8. [Crossref] [PubMed]
- Ari A, Harwood R, Sheard M, et al. In vitro comparison of heliox and oxygen in aerosol delivery using pediatric high flow nasal cannula. Pediatr Pulmonol 2011;46:795-801. [Crossref] [PubMed]
- Réminiac F, Vecellio L, Loughlin RM, et al. Nasal high flow nebulization in infants and toddlers: An in vitro and in vivo scintigraphic study. Pediatr Pulmonol 2017;52:337-44. [Crossref] [PubMed]
- Li J, Gong L, Ari A, et al. Decrease the flow setting to improve trans-nasal pulmonary aerosol delivery via "high-flow nasal cannula" to infants and toddlers. Pediatr Pulmonol 2019;54:914-21. [Crossref] [PubMed]
- Bass K, Boc S, Hindle M, et al. High-Efficiency Nose-to-Lung Aerosol Delivery in an Infant: Development of a Validated Computational Fluid Dynamics Method. J Aerosol Med Pulm Drug Deliv 2019;32:132-48. [Crossref] [PubMed]
- Longest PW, Tian G. Development of a new technique for the efficient delivery of aerosolized medications to infants on mechanical ventilation. Pharm Res 2015;32:321-36. [Crossref] [PubMed]
- Anderson M, Svartengren M, Camner P. Human tracheobronchial deposition and effect of a cholinergic aerosol inhaled by extremely slow inhalations. Exp Lung Res 1999;25:335-52. [Crossref] [PubMed]
- Heyder J GJ, Rudolf G, Schiller CF, Stahlhofen W. Deposition of Particles in the Human Respiratory Tract in the Size Range of 0.005-15 micron. J Aerosol Sci 1986;17:811-25. [Crossref]
- Bennett WD, Brown JS, Zeman KL, et al. Targeting delivery of aerosols to different lung regions. J Aerosol Med 2002;15:179-88. [Crossref] [PubMed]
- Corcoran TE, Venkataramanan R, Hoffman RM, et al. Systemic delivery of atropine sulfate by the MicroDose Dry-Powder Inhaler. J Aerosol Med Pulm Drug Deliv 2013;26:46-55. [Crossref] [PubMed]
- Golshahi L, Vehring R, Noga ML, et al. In vitro deposition of micrometer-sized particles in the extrathoracic airways of children during tidal oral breathing. J Aerosol Sci 2013;57:14-21. [Crossref]
- Hofmann W. Mathematical model for the postnatal growth of the human lung. Respir Physiol 1982;49:115-29. [Crossref] [PubMed]
- Golshahi L, Finlay WH. An Idealized Child Throat that Mimics Average Pediatric Oropharyngeal Deposition. J Aerosol Sci Tech 2012;46:1. [Crossref]