Discovering biomarkers of radioresistance in a radiosensitive cancer: a clinical paradox in nasopharyngeal carcinoma
Radiotherapy (RT) is an integral modality in the treatment of human cancers, with more than 50% of cancer patients requiring RT in their lifetime. In recent years, the therapeutic ratio of RT has improved dramatically due to the technological advances of RT delivery with image guidance and dosimetric modulation of doses to the tumour and adjacent normal tissues (1). Nonetheless, tumours of different histological subtypes and tissue origins harbour heterogeneous responses to RT, owing to inter-cell type variation in intrinsic cellular radiosensitivity and microenvironment effects (2). While factors such as hypoxia (3,4) and modulation of DNA repair (5) are tumour-agnostic pathways that can lead to radioresistance, distinct mechanisms are also at play in specific tumour types. It is thus important to develop a knowledge bank of molecular profiles that are associated with radioresistance across multiple cancer types and cell lines, such that appropriate drug and RT combinatorial regimes can be investigated pre-clinically, with subsequent transition to early and late phase clinical trials.
It is in this background that Dong and colleagues undertook a study to investigate the involvement of mixed lineage kinase domain-like pseudokinase (MLKL) in modulating radiosensitivity in nasopharyngeal carcinoma (NPC). NPC is a unique head and neck epithelial tumour that is prevalent in specific parts of the world, namely Southern China, Southeast Asia, North Africa and the Artic (6). The endemic variant of NPC is invariably associated with EBV infection, with expression of EBV-associated proteins found in both precursor and malignant lesions (7). NPC is an exquisitely radiosensitive tumour, with excellent locoregional control rates of 70–98% in the era of intensity modulated radiotherapy (IMRT) (8,9). Tumour control is enhanced by the combination of platinum-based chemotherapy with RT (given the radiosensitising properties of cisplatin); locoregional control rates were reported to be 88–90% with the addition of chemotherapy compared to 73–78% with RT alone. However, about 20% NPC patients still experience local failure following IMRT (1,10). For these patients, reirradiation is challenging, given the significantly elevated toxicities caused by cumulative high dose irradiation of normal tissues (11-13). Toxicities are potentially morbid and fatal, which include severe mucosal necrosis and neurological complications, like brachioplexiopathy, cranial nerve palsies, cerebral necrosis and encephalopathy (12). Careful patient selection for reirradiation is thus necessary, given that treatment-related toxicities account for half of all mortality in the reirradiated patients. Nonetheless, a more effective approach should entail early intervention by using biomarker-based identification of radioresistant disease that can be matched to appropriate treatment intensification at the time of first diagnosis.
In their study, Dong et al. showed that depletion of MLKL sensitises NPC tumour cells to radiation through downregulation of the epithelial-mesenchymal transition (EMT) pathways (13). They utilised a radioresistant CNE-2 cell line model, which had been generated by repeated exposure of cells to fractionated RT (two doses of 2, 4, 6, 8, 10 Gy) over a 5-month period (14). They first showed that silencing of MLKL (by siRNA and CRISPR-Cas 9 knockout techniques) reversed the pro-invasiveness and pro-survival phenotypes of the radioresistant CNE-2 cells, but not in the parental cells. Gene enrichment analyses subsequently revealed that MLKL depletion was associated with downregulation of the transforming growth factor-beta (TGF-β) signalling pathway, and upregulation of the adherent junction and the extracellular matrix-receptor interaction pathways. Additional protein-to-protein interaction network analyses showed an increased expression of nine hub genes (EGFR, JUN, CD44, SPP1, VIM, IL-8, BCL2, WDFY2, PIK3CD) and reduced expression of CDH1 in the radioresistant CNE-2 compared to the parental cells. The downstream effects on EGFR, JUN, CD44, SPP1, VIM, MMP1 and CDH1 were reversed with the silencing of MLKL. Based on these findings, the investigators proposed that activation of MLKL potentially underpins radioresistance in NPC.
To date, there is no data linking the MLKL gene to radioresistance in NPC. Admittedly, the study findings by Dong et al. remain preliminary, especially since the results are elucidated in a single cell line radioresistant and parental pair. While there is certainly mechanistic evidence supporting the involvement of MLKL in pro-survival signalling pathways in CNE-2, the authors had not validated their findings either in vivo or using patient-derived samples. For the latter, a simple experiment would be to first test for interpatient variation of MLKL expression in tumour tissues using immunohistochemistry; following which, patients can be stratified by an optimal cut-off for MLKL expression to examine if patients harbouring a “high” expression of MLKL were more likely to recur than those with a “normal or low” expression of the gene. However, given the low rates of locoregional recurrence (<20%) in NPC, a large sample size will be needed for such an analysis, given the need to correct for clinical confounders like T-status, tumour bulk, RT dose and use of concomitant chemotherapy.
There is preliminary experimental evidence linking MLKL to radioresistance in other tumour types, such as breast (15), colorectal (16) and lung cancers (17). Currently, the function of MLKL is largely unknown, except for its role in mediating tumour necrosis factor (TNF)-induced necroptosis by binding to the receptor-interacting protein 3 (RIP-3). Necroptosis is a form of programmed necrotic cell death that can occur following exposure to RT, and has been demonstrated to trigger anti-tumour immunity post-irradiation (16). Paradoxically, in radioresistant human colorectal cancer cell lines, necroptosis was shown to stimulate tumour repopulation after in vitro irradiation (16). Separately, a comparative analyses of the global kinome between radioresistant MCF-7/C6 cells and their parental MCF-7 human breast cancer cells also revealed overexpression of MLKL (15). In the present study by Dong et al., levels of epithelial markers were significantly decreased, while mesenchymal markers were increased in the radioresistant cells. Upon MLKL depletion, these epithelial markers were restored in the radioresistant cells, with corresponding suppression of the EMT pathways, thereby reversing the activation of PI3K/Akt/mTOR pathway, which is culpable for promoting radioresistance (18,19). In addition, MLKL dysfunction has also been suggested as a prognostic biomarker for gastric, ovarian, cervical and pancreatic cancers (20).
All things considered, the findings of this study are relevant, given the modern-day advocacy to perform molecular profiling of patients’ tumour for individualised risk prediction in the era of precision RT—the rationale being understanding the biology can assist the physician in recommending treatment intensification or deintensification (21). High-end molecular diagnostics are now increasingly accessible due to the emergence of low-cost, high-throughput solutions for next-generation sequencing (NGS). Consequently, large-scale, molecular profiling cohort studies have uncovered novel molecular drivers of carcinogenesis and treatment resistance, which could lead to the discovery of novel drugs to combine with RT. As a case in point, we recently identified a germline SNP (rs1131636-T) located at the RPA1 gene that is associated with an inferior survival after IMRT (22). Based on an exome-wide association study of 5,553 NPC patients, we showed that the presence of this SNP led to an overexpression of RPA1, which was associated with radioresistance in vitro and a higher likelihood of locoregional relapse in our cohort of NPC patients. The linkage of RPA1 to radioresistance was further supported by observation of incremental RPA1 expression in post-RT recurrences when compared to the paired pre-RT tumours (23). Interestingly, we found that miR-1253 could bind to the 3'-UTR region of the RPA1 gene and downregulate its expression, consequently reversing the radioresistance phenotype. More work is needed to understand the gene regulatory network of RPA1, so that this genetic mutation can be utilised as both a prognostic biomarker, and to predict the efficacy of potential therapeutics that target the dependency on RPA1 dysregulation (Figure 1).
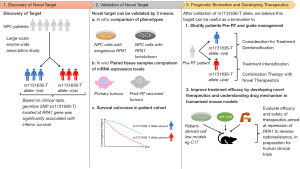
Perhaps, among several reasons for the dormant drug development space in NPC, a notable limitation pertains to the paucity of primary cell line models that maintain the expression of EBV-specific proteins. Among the commercial and patient-derived human cell lines, only a few [C666-1 and C17 (24,25)] are known to express EBV-specific proteins; often, this characteristic is lost with multiple passages, which thus begs the question if the experimental findings at this stage truly mimic the processes in vivo. Moreover, referring to the experimental method of Dong et al. in generating their radioresistant CNE-2 model, it is contentious if the radioresistant tumour clones reflect the characteristics of de novo radioresistant cells that are admixed with the radiosensitive ones in the untreated tumour, following a series of clonal selection and re-expansion of surviving cells post-irradiation. Alternatively, another approach would entail screening of candidate genes for gene silencing via CRISPR-Cas9 or siRNA to induce a reversible radioresistant phenotype. However, this method is thus confined to known pathways of interest and limits the ability to interrogate the interplay of multiple gene networks that underpin radioresistance. Finally, to address the issue of EBV maintenance in patient-derived cell lines, Lin et al. recently reported on the successful engraftment of a patient-derived C17 cell line, which was able to retain the EBV episome and demonstrate lytic reactivation of EBV. Likewise, Liu et al. recently showcased their ability to generate immunocompetent NPC models by engraftment of patient-derived tumour cells onto humanised mouse models (26). Future mechanistic and drug discovery experiments should attempt to utilise such models, where possible.
In conclusion, Dong and colleagues ought to be congratulated for the discovery of MLKL as a regulator of radiosensitivity in NPC. This data adds to the previous studies in colorectal and breast cancers, whereby we now understand that MLKL can exert its effects on radiation response via the EMT and necroptosis pathways. Going forward, the next steps will entail understanding if MLKL-induced radioresistance is exclusive to NPC or rather tumour-agnostic. Additionally, future studies should investigate the utility and implementation of MLKL as a clinically usable biomarker.
Acknowledgments
Funding: None.
Footnote
Provenance and Peer Review: This article was commissioned by the editorial office, Annals of Translational Medicine. The article did not undergo external peer review.
Conflicts of Interest: Both authors have completed the ICMJE uniform disclosure form (available at http://dx.doi.org/10.21037/atm-20-5405). MLKC reports personal fees from Janssen, personal fees from Astellas, personal fees and non-financial support from Varian, personal fees from MSD Oncology, personal fees from Bayer, personal fees and non-financial support from AstraZeneca, personal fees from Illumina, non-financial support from PV Med, from Decipher Biosciences, outside the submitted work. The other author has no conflicts of interest to declare.
Ethical Statement: The authors are accountable for all aspects of the work in ensuring that questions related to the accuracy or integrity of any part of the work are appropriately investigated and resolved.
Open Access Statement: This is an Open Access article distributed in accordance with the Creative Commons Attribution-NonCommercial-NoDerivs 4.0 International License (CC BY-NC-ND 4.0), which permits the non-commercial replication and distribution of the article with the strict proviso that no changes or edits are made and the original work is properly cited (including links to both the formal publication through the relevant DOI and the license). See: https://creativecommons.org/licenses/by-nc-nd/4.0/.
References
- Sun XS, Liu SL, Luo MJ, et al. The Association Between the Development of Radiation Therapy, Image Technology, and Chemotherapy, and the Survival of Patients With Nasopharyngeal Carcinoma: A Cohort Study From 1990 to 2012. Int J Radiat Oncol Biol Phys 2019;105:581-90. [Crossref] [PubMed]
- Karar J, Maity A. Modulating the tumor microenvironment to increase radiation responsiveness. Cancer Biol Ther 2009;8:1994-2001. [Crossref] [PubMed]
- Begg AC, Stewart FA, Vens C. Strategies to improve radiotherapy with targeted drugs. Nat Rev Cancer 2011;11:239-53. [Crossref] [PubMed]
- Chan N, Koritzinsky M, Zhao H, et al. Chronic hypoxia decreases synthesis of homologous recombination proteins to offset chemoresistance and radioresistance. Cancer Res 2008;68:605-14. [Crossref] [PubMed]
- He Y, Jing Y, Wei F, et al. Long non-coding RNA PVT1 predicts poor prognosis and induces radioresistance by regulating DNA repair and cell apoptosis in nasopharyngeal carcinoma. Cell Death Dis 2018;9:235. [Crossref] [PubMed]
- Chua MLK, Wee JTS, Hui EP, et al. Nasopharyngeal carcinoma. Lancet 2016;387:1012-24. [Crossref] [PubMed]
- Pathmanathan R, Prasad U, Sadler R, et al. Clonal proliferations of cells infected with Epstein-Barr virus in preinvasive lesions related to nasopharyngeal carcinoma. N Engl J Med 1995;333:693-8. [Crossref] [PubMed]
- Au KH, Ngan RKC, Ng AWY, et al. Treatment outcomes of nasopharyngeal carcinoma in modern era after intensity modulated radiotherapy (IMRT) in Hong Kong: A report of 3328 patients (HKNPCSG 1301 study). Oral Oncol 2018;77:16-21. [Crossref] [PubMed]
- Gregoire V, Ng WT. Special section on intensity-modulated radiation therapy for head and neck cancer (IMRT). Oral Oncol 2019;88:49-50. [Crossref] [PubMed]
- Chan OS, Sze HC, Lee MC, et al. Reirradiation with intensity-modulated radiotherapy for locally recurrent T3 to T4 nasopharyngeal carcinoma. Head Neck 2017;39:533-40. [Crossref] [PubMed]
- Li YQ, Tian YM, Tan SH, et al. Prognostic Model for Stratification of Radioresistant Nasopharynx Carcinoma to Curative Salvage Radiotherapy. J Clin Oncol 2018;36:891-9. [Crossref] [PubMed]
- Lee AWM, Ng WT, Chan JYW, et al. Management of locally recurrent nasopharyngeal carcinoma. Cancer Treat Rev 2019;79:101890. [Crossref] [PubMed]
- Ng WT, Wong ECY, Cheung AKW, et al. Patterns of care and treatment outcomes for local recurrence of NPC after definite IMRT-A study by the HKNPCSG. Head Neck 2019;41:3661-9. [Crossref] [PubMed]
- Bao C, Sun Y, Dong Y, et al. The relative biological effectiveness of proton and carbon ion beams in photon-sensitive and resistant nasopharyngeal cancer cells. Transl Cancer Res 2018;7:170-9. [Crossref]
- Guo L, Xiao Y, Fan M, et al. Profiling global kinome signatures of the radioresistant MCF-7/C6 breast cancer cells using MRM-based targeted proteomics. J Proteome Res 2015;14:193-201. [Crossref] [PubMed]
- Wang Y, Zhao M, He S, et al. Necroptosis regulates tumor repopulation after radiotherapy via RIP1/RIP3/MLKL/JNK/IL8 pathway. J Exp Clin Cancer Res 2019;38:461. [Crossref] [PubMed]
- Yuan ZY, Meng MB, Wu ZQ, et al. Stereotactic Body Radiation Oncology Exert Anti-Tumor Effect via RIP3-Dependent Necroptosis in Non-Small Cell Lung Cancer Cells Int J Radiat Oncol Biol Phys 2015;93:E533. [Crossref]
- Theys J, Jutten B, Habets R, et al. E-Cadherin loss associated with EMT promotes radioresistance in human tumor cells. Radiother Oncol 2011;99:392-7. [Crossref] [PubMed]
- Chang L, Graham PH, Hao J, et al. Acquisition of epithelial-mesenchymal transition and cancer stem cell phenotypes is associated with activation of the PI3K/Akt/mTOR pathway in prostate cancer radioresistance. Cell Death Dis 2013;4:e875. [Crossref] [PubMed]
- Sun W, Yu W, Shen L, et al. MLKL is a potential prognostic marker in gastric cancer. Oncol Lett 2019;18:3830-6. [Crossref] [PubMed]
- Tan JSH, Lin X, Chua KLM, et al. Exploiting molecular genomics in precision radiation oncology: a marriage of biological and physical precision. Chin Clin Oncol 2017;6:S19. [Crossref] [PubMed]
- Guo YM, Chen JR, Feng YC, et al. Germline Polymorphisms and Length of Survival of Nasopharyngeal Carcinoma: An Exome-Wide Association Study in Multiple Cohorts. Adv Sci (Weinh) 2020;7:1903727. [Crossref] [PubMed]
- Zhang Z, Huo H, Liao K, et al. RPA1 downregulation enhances nasopharyngeal cancer radiosensitivity via blocking RAD51 to the DNA damage site. Exp Cell Res 2018;371:330-41. [Crossref] [PubMed]
- Yip YL, Lin W, Deng W, et al. Establishment of a nasopharyngeal carcinoma cell line capable of undergoing lytic Epstein-Barr virus reactivation. Lab Invest 2018;98:1093-104. [Crossref] [PubMed]
- Cheung ST, Huang DP, Hui AB, et al. Nasopharyngeal carcinoma cell line (C666-1) consistently harbouring Epstein-Barr virus. Int J Cancer 1999;83:121-6. [Crossref] [PubMed]
- Liu WN, Fong SY, Tan WWS, et al. Establishment and Characterization of Humanized Mouse NPC-PDX Model for Testing Immunotherapy. Cancers (Basel) 2020;12:1025. [Crossref] [PubMed]