Unusual images of ischemic stroke with hyperacute spontaneous recanalization: a case report
Introduction
Neuroimaging is essential for stroke assessment. Computed tomography (CT) and magnetic resonance imaging (MRI) protocols are excellent tools for evaluating acute ischemic stroke. On CT scan, several special signs, such as an obscured outline of the lentiform nucleus, a slight decrease in tissue density, and effacement of the cortical sulci, can help detect cerebral infarct in the early stage (1). The detection rate for ischemic stroke within 6 hours has been reported to range from 21.4% (1) to 46.0% (2), but an MRI scan is more sensitive to ischemic lesions than a CT scan, and can demonstrate differentiation in the earlier phases of ischemic stroke.
The general images embrace a high diffusion-weighted magnetic resonance imaging (DWI) signal and a low apparent diffusion coefficient (ADC) signal during the acute phase of infarction. In the hyperacute phase, and as early as 10 minutes after symptomatic onset, a low ADC signal can emerge in the ischemic territory (3). These particular ischemic images will change several days later. The ADC of ischemic regions stays significantly reduced for 3 to 4 days, increases progressively over time to be pseudonormalized between day 5 and 10, and elevates significantly in the chronic state, making the distinction of acute lesions adjacent to chronic infarcts readily apparent (4,5). The high DWI signal persists after the onset of ischemic symptoms for as long as 1 week or more and then decreases gradually (4).
We here present a case of an ischemic stroke with hyperacute spontaneous recanalization, showing a hyperdensity on CT scan 4 hours after onset, and a slightly lower DWI signal and an equal ADC signal on MRI scan 12 hours after onset.
We present the following case in accordance with the CARE reporting checklist (available at http://dx.doi.org/10.21037/atm-20-5055).
Case presentation
This research is a retrospective and observational study. All procedures performed in studies involving human participants were in accordance with the ethical standards of the institutional and/or national research committee(s) and with the Helsinki Declaration (as revised in 2013). Written informed consent was obtained from the patient for publication of this study and any accompanying images.
A 62-year-old man with no vascular risk factors had an acute onset of right-sided weakness associated with speech difficulties 12 hours before presentation. The patient had no medical history of hypertension, diabetes mellitus, heart disease, or hematological disease. On admission, neurological examination showed sleepiness, confusion, dysphasia, dysphagia, sensitive pupillary response reacting directly and consensually, horizontal conjugate gaze palsy to the left, facial droop on the left side, grade 0 muscle strength of the right upper limb, grade 2 muscle strength of the right lower limb, and positive Babinski sign. National Institute of Health stroke scale (NIHSS) score was 18.
The patient underwent CT scans 3 and 4 hours after onset respectively, which demonstrated a hypodensity in the left basal ganglia including caudate and internal capsule with a hyperdense middle cerebral artery (MCA) sign. Unusually, an obviously increased density of the lenticula emerged on CT scan 4 hours after onset, in marked contrast to hypodensity found 1 hour previous (Figure 1).
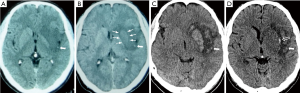
The patient underwent MRI immediately after presentation, which showed abnormal signals in the territory of the left basal ganglia with a disappearance of the anterior part of the left ventricular cavity and an unobstructed lumen of the first segment of the left MCA on magnetic resonance angiography (MRA). Except for the region of the lenticula and head of the caudate, this ischemic territory presented the typical characters of acute infarct on MRI scan, which included lower ADC and T1-weighted images (T1) signals, along with higher DWI, T2-weighted images (T2), and fluid-attenuated inversion recovery (FLAIR) signals. In contrast, a slightly lower DWI signal and an equal ADC signal were found in the region of both the lenticula and caudate (Figure 2).

The patient was diagnosed as acute cerebral infarction according to the patient’s medical history, symptoms, signs and imaging findings, and was given medical and rehabilitated treatments. The patient received conventional medical treatment including dual antiplatelet (100 mg/d aspirin and 75 mg/d clopidogrel, first dose with 300 mg respectively), atorvastatin, ganglioside, and edaravone. On the third day after onset, the patient regained clear consciousness and quick response without improvement of muscle strength, and his NIHSS score decreased to 14. On the 7th day, a CT scan demonstrated significant hyperdensity in both the lenticula and caudate with a hyperdense MCA sign, surrounded by hypodensity tissue. On the 14th day, another CT scan showed that the density of the lenticula and caudate had been restored to be the same as the opposite corresponding site with a new hyperdensity emerging at the edge of the lenticula (Figure 1).
Etiological tests for ischemic stroke were completed during hospitalization. Holter electrocardiography (applied twice) revealed sinus rhythm without atrial fibrillation. Neither transthoracic nor transesophageal color Doppler revealed abnormalities of intracardiac thrombus, ventricular aneurysm, patent foramen ovale, or aortic atherosclerotic plaque. Carotid ultrasonography did not show unstable plaque or arterial dissection. A transcranial Doppler (TCD) saline bubble test was negative. Laboratory examination results of coagulation, antiphospholipid antibody, and the indexes of cancer, rheumatism, and immunity were normal.
Discussion
The evolutional process of cerebral ischemic lesion on MR imaging
Ischemia and hypoxia damage cerebral tissue with ATP depletion and failure of ATP-dependent ion pumps, such as Na+-K+ exchange pumps, which leads to the failure of neuronal electrical function and energy metabolism (6). Dysfunction of cerebral capillaries due to ischemia, hypoxia and post-ischemic reperfusion leads to a progressive deterioration of the permeability of the blood-brain barrier (BBB), resulting in the formation of cytotoxic edema, ionic edema, vasogenic edema, and hemorrhagic conversion (7).
In the stage of cytotoxic edema, only neurons suffer from the failure of Na+-K+ exchange pumps, which allows Na+ from the extracellular space into neurons, resulting in the formation of a new gradient for Na+. The gradient acts as a driving force for the transcapillary movement of edema fluid, but the driving force is not released due to the intact permeability properties of the BBB contained by endothelial cells without edema. Thus, intravascular contents will not move outside, so no new constituent is added in the ischemic territory and no tissue swelling occurs (7). Ionic edema results from the dysfunction of endothelial cells, allowing Na+ from the intravascular compartment to the extracellular space through cation channels located in the capillary wall. Then Cl− and water follow via their own respective channels, completing the process of formation of ionic edema. Vasogenic edema results from the degradation of tight junctions between endothelial cells, allowing extravasation of proteinaceous fluid. When the oncotic death of endothelial cells leads to complete loss of capillary integrity, blood extravasation, namely hemorrhagic conversion, takes place (7).
Thus, tissue swelling resulting from newly added constituents (edema liquids) in ischemic territory occurs in the phases of ionic edema, vasogenic edema, and hemorrhagic conversion. The edema liquids come from the surrounding region without ischemia (8,9). The timing of the tissue swelling depends on the process of edema liquid transferring from the surrounding to the anemic infarct core.
DWI is sensitive to cytotoxic and vasogenic edema, but ADC is predominantly subjected to cytotoxic edema (10). The ADC can fall to 0.4×10−3 mm2/s in acute infarction and increase up to 2.5×10−3 mm2/s [equivalent to the ADC of cerebrospinal fluid (CSF)] in chronic lesions and necrosis (10). ADC ≤0.62×10−3 mm2/s is considered as irreversible ischemic injury (11). In Warach’s study (4), the mean ADC of ischemic regions was 0.493×10−3mm2/s at 6 hours or less and stayed reduced for 3 to 4 days after the onset of ischemia. The relative ADC increased progressively over time to be pseudonormalized at 5 to 10 days and rose as high as 2.5×10−3 mm2/s in a chronic state. The pseudonormalized phenomenon occurred as early as 48 hours after ischemic onset.
In this study, MRI showed a slightly decreased DWI signal and an equal ADC signal in both the lenticula and caudate 12 hours after onset, while the ADC in Warach’s study increased to a pseudonormalized state as early as 48 hours after onset. We speculate that this difference should be due to the spontaneous recanalization in the hyperacute stage of cerebral infarction. As soon as recanalization, the edema liquids reached the infarct score directly and were extravasated immediately without travelling from the surrounding region into the infarct core. Thus, the process of tissue swelling resulting from ischemia accelerated significantly, and the pseudonormalized ADC image of the lenticula and caudate emerged only 12 hours after the onset of ischemia.
The evolutional process of cerebral ischemic lesion on CT scan
The increase in the water content of the brain causes Xray attenuation, which manifests as hypodensity on CT (2). In the cytotoxic edema phase, due to a lack of newly added constituent and tissue swelling, CT does not demonstrate hypodensity. Yet, the loss of the gray-white matter distinction emerges on CT scan because of focal changes of CT attenuation resulting from Na+ transferring from the extracellular space into the neurons. In contrast, CT can detect ionic edema mainly resulting from newly added tissue water and shows hypodensity (12). During the vasogenic edema stage, protein contents leaking into extravascular space from arterial lumen increase the density of ischemic regions on CT scan. During blood extravasation, the high protein content of the hematoma appears on CT as hyperdensity higher than that of the corresponding opposite site (13).
In this study, spontaneous recanalization accelerated the process of tissue swelling; thus, a hypodensity in the ischemic region was seen on CT 3 hours after onset, which reflected the phase of ionic edema. One hour later, the density of the lenticula increased obviously, suggesting vasogenic edema had emerged in the lenticula with protein content increase. The density of the lenticula, head of the caudate, and insula was higher than that of the corresponding opposite site on the seventh day and fell to almost normal on the fourteenth day. Therefore, the series of CT images acquired during the entire duration of ischemic stroke reflected the accelerated progress of tissue edema caused by reperfusion.
The hyperdense sign in the second segment of the middle cerebral artery was key to determining that an embolus had obstructed the proximal MCA before fragmenting and moving forward. However, a definite origin of the embolus could not be confirmed by current etiological tests, and thus the patient was finally diagnosed with cerebral embolism of unknown origin.
Conclusions
Both ischemic injury and reperfusion affect the process of cerebral edema resulting from ischemia, and subsequently contribute to the imaging of ischemic stroke on CT and MRI scans. Reperfusion promotes the development of cerebral edema and accelerates the evolution of infarct images. When hyperacute recanalization occurs, acute ischemic stroke could manifest as hyperdensity on CT, and slightly lower DWI signal and higher ADC signal on MRI.
Acknowledgments
Funding: None.
Footnote
Reporting Checklist: The authors have completed the CARE reporting checklist. Available at http://dx.doi.org/10.21037/atm-20-5055
Conflicts of Interest: All authors have completed the ICMJE uniform disclosure form (available at http://dx.doi.org/10.21037/atm-20-5055). The authors have no conflicts of interest to declare.
Ethical Statement: The authors are accountable for all aspects of the work in ensuring that questions related to the accuracy or integrity of any part of the work are appropriately investigated and resolved. All procedures performed in studies involving human participants were in accordance with the ethical standards of the institutional and/or national research committee(s) and with the Helsinki Declaration (as revised in 2013). Written informed consent was obtained from the patient for publication of this study and any accompanying images.
Open Access Statement: This is an Open Access article distributed in accordance with the Creative Commons Attribution-NonCommercial-NoDerivs 4.0 International License (CC BY-NC-ND 4.0), which permits the non-commercial replication and distribution of the article with the strict proviso that no changes or edits are made and the original work is properly cited (including links to both the formal publication through the relevant DOI and the license). See: https://creativecommons.org/licenses/by-nc-nd/4.0/.
References
- Noriaki T, Kazuo U, Atsushi I, et al. Early CT finding in cerebral infarction: obscuration of the lentiform nucleus. Radiology 1988;168:463-7. [Crossref] [PubMed]
- Merino JG, Warach S. Imaging of acute stroke. Nat Rev Neurol 2010;6:560-71. [Crossref] [PubMed]
- Harris AD, Coutts SB, Frayne R, et al. Diffusion and Perfusion MR Imaging of Acute Ischemic Stroke. Magn Reson Imaging Clin N Am 2009;17:291-313. [Crossref] [PubMed]
- Warach S, Gaa J, Siewert B, et al. Acute human stroke studied by whole brain echo planar diffusion-weighted magnetic resonance imaging. Ann Neurol 1995;37:231-41. [Crossref] [PubMed]
- Schlaug G, Siewert B, Benfield A, et al. Time course of the apparent diffusion coefficient (ADC) abnormality in human stroke. Neurology 1997;49:113-9. [Crossref] [PubMed]
- Kulik T, Kusano Y, Aronhime S, et al. Regulation of cerebral vasculature in normal and ischemic brain. Neuropharmacology 2008;55:281-8. [Crossref] [PubMed]
- Simard JM, Kent TA, Chen M, et al. Brain oedema in focal ischaemia: molecular pathophysiology and theoretical mplications. Lancet Neurol 2007;6:258-68. [Crossref] [PubMed]
- Warach S, Latour LL. Evidence of reperfusion injury, exacerbated by thrombolytic therapy, in human focal brain ischemia using a novel imaging marker of early blood-brain barrier disruption. Stroke 2004;35:2659-61. [Crossref] [PubMed]
- Quast MJ, Huang NC, Hillman GR, et al. The evolution of acute stroke recorded by multimodal magnetic resonance imaging. Magn Reson Imaging 1993;11:465-71. [Crossref] [PubMed]
- Gass A, Niendorf T, Hirsch JG. Acute and chronic changes of the apparent diffusion coefficient in neurological disorders-biophysical mechanisms and possible underlying histopathology. J Neurol Sci 2001;186 Suppl 1:S15-23. [Crossref] [PubMed]
- Purushotham A, Campbell BC, Straka M, et al. Apparent diffusion coefficient threshold for delineation of ischemic core. Int J Stroke 2015;10:348-53. [Crossref] [PubMed]
- von Kummer R, Dzialowski I. Imaging of Cerebral Ischemic Edema and Neuronal Death. Neuroradiology 2017;59:545-53. [Crossref] [PubMed]
- Becker H, Desch H, Hacker H, et al. CT fogging effect with ischemic cerebral infarcts. Neuroradiology 1979;18:185-92. [Crossref] [PubMed]
(English Language Editor: J. Gray)