TLE2 is associated with favorable prognosis and regulates cell growth and gemcitabine sensitivity in pancreatic cancer
Introduction
Pancreatic ductal adenocarcinoma (PDAC) is the most common pancreatic malignancy and one of the most challenging cancers to treat (1). Despite the substantial therapeutic advancements, the prognosis for patients with PDAC is still dismal (2-4). Therefore, a better understanding of the molecular biology and tumor genetics of PDAC, and new therapeutic targets that can improve patient outcomes are urgently needed.
Transcriptional corepressors are a group of proteins that play vital roles in the transcriptional regulation of various genes (5,6). The Groucho (Gro)/transducin-like enhancer of split (TLE) proteins are a large family of gene regulators that are widely expressed in eukaryotes and influence the transcriptional activity of numerous genes (7). Instead of directly binding to DNA strands, TLE proteins bind to other transcription factors (8,9), usually resulting in transcriptional repression (10,11). TLE proteins have been observed to interact with transcriptional factors, including Hes, c-Myc, and Runx (12,13). Additionally, some studies have reported that TLE1 and TLE3 can participate in tumor development as tumor suppressor genes (14-17).
TLE2 is a member of the TLE gene family located on chromosome 19p13.3. Previous studies have revealed that TLE2 participates in the development of pancreatic tissue through its interactions with a series of transcription factors (18). Furthermore, TLE2 is involved in the ventral telencephalon from the early neural plate stage during neurogenesis (19). Many genes known to be involved in the regulation of histogenesis and tissue development, such as Robo and Wnt, may also perform pivotal roles in neoplastic conditions (20-22). Currently, the role TLE2 plays in most human cancers, especially pancreatic cancer, is a long way from being elucidated. Therefore, this study aimed to examine the prognostic value and cellular function of TLE2 in PDAC through in silico and in vitro study for the first time, in order to explore the role of TLE2 in PDAC and to elucidate the underlying mechanisms. We present the following article in accordance with the MDAR reporting checklist (available at http://dx.doi.org/10.21037/atm-20-5492).
Methods
Cells and reagents
PANC-1 and MiaPaCa-2 human pancreatic cancer cell lines were obtained from American Type Culture Collection (ATCC) and maintained in Dulbecco’s Modified Eagle Medium (DMEM; Invitrogen) with 10% fetal bovine serum (HyClone) at 37 °C with 5% CO2.
Transfection of siRNA
MiaPaCa-2 cells were transfected with siRNAs targeting the sequence 5'-CTCCAGAAATAACAAATACATCG-3' of TLE2. A scramble (5'-GGACGGAGCAGTACAA-3') without known targets was chosen as a non-sense control. Transfection was performed with Lipofectamine 2000 (Thermo Fisher Scientific, USA) in line with the manufacturer’s protocol. siRNA and control oligonucleotides were synthesized by Jennio Biological Technology (Guangzhou, China).
Lentiviral transfection for TLE2 overexpression
The TLE2 overexpression lentiviral vector was established by recombining the pGC-LV-GV287-GFP vector with the TLE2 gene. PANC-1 cells overexpressing TLE2 were constructed via lentiviral-mediated plasmid transfection (MOI =10). An empty vector served as a transfection control. Untransfected cells were used as a normal control. TLE2 expression was validated by quantitative reverse transcription polymerase chain reaction (qRT-PCT) and western blot analysis.
RNA extraction and qRT-PCR
Total RNA was extracted from cells using TRIZOL reagent (Invitrogen), and cDNA was reverse transcribed with the cDNA Reverse Transcription Kit (Takara Biotechnology). Then, qRT-PCR was performed on each cDNA template in 20-µL reaction systems using the SYBR Green Kit (Takara, Japan) on an ABI 7500HT Fast real-time PCR system. The following primers were used for the amplification of TLE2 fragments: forward 5'-TATTTCCGATTACGGCACTCG-3'; reverse 5'-CTACTCTCCATTCCGACCGC-3', GAPDH was used as internal control (forward 5'-TTGTCTCCTGCGACTTCAACAG-3'; reverse 5'-GGTCTGGGATGGAAATTGTGAG-3').
Western blot analysis
Pancreatic cancer cell lines were washed and resuspended with PBS twice. Total protein was isolated and quantified using RIPA extraction buffer and the BCA kit (Pierce). The protein samples were separated by 10% SDS-PAGE and transferred onto polyvinylidene fluoride (PVDF) membranes. The membranes were then blocked with buffer containing 5% non-fat milk and incubated with TLE2 antibody (rabbit polyclonal anti-human, Abcam, USA; 1:200, No. ab206147). Anti-rabbit IgG was used as the secondary antibody (Santa Cruz Biotechnology, No. sc-2357). The blots were visualized with an ELC kit (Millipore, USA).
Cell viability assays
For the evaluation of cell proliferation, cell viability assays were carried out. Briefly, different groups of PANC-1 cells and MiaPaCa-2 cells (control, scramble transfection, empty vector transfection, siTLE2, and TLE2 overexpression) were seeded in 96-well plates at a density of 1,000 cells/well and incubated for 72 hours at 37 °C with 5% CO2. For gemcitabine cytotoxicity experiments, groups of PANC-1 and MiaPaCa-2 cells, as described above, were seeded in 96-well plates at a density of 1,000 cells/well. The cells were treated with different concentrations of gemcitabine for 48 hours. For both assays, the MTT absorbance was finally evaluated at 570 nm to determine cell viability.
Invasion assays
PANC-1 and MiaPaCa-2 cells with the indicated treatments were cultured in 6-well plates and re-suspended in serum-free medium. Cell invasion was studied by performing a Transwell assay with the chamber precoated with Matrigel (8.0 µm pore size, Corning) and a cell density of 2.0×104 cells/well. The numbers of migrated cells on the lower side of the Transwell membrane were counted and compared.
Cell cycle analysis
Flow cytometry was carried out to detect the distribution of cell cycle. Briefly, cells were transfected with either siRNA targeting TLE2 or a plasmid overexpressing TLE2. Single cell suspensions were prepared 48 hours after transfection. Cell aliquots were fixed in 70% ethanol and stained with propidium iodide for flow cytometry analysis using a FACScan instrument (BD Biosciences, USA).
Survival analysis
The prognostic values of TLE1, TLE2, and TLE3 in cancers were analyzed according to the transcriptome data and clinical parameters obtained from The Cancer Genome Atlas (TCGA; http://cancergenome.nih.gov/), which were analyzed with transcripts per million (TPM) as log2 (TPM +1) in Gene Expression Profiling Interactive Analysis (GEPIA; http://gepia.cancer-pku.cn) portal (23).
Functional annotation analysis
The RNA-seq data (FPKM, fraction per kilobase per million reads) of PDAC were downloaded from the TCGA portal (TCGA-PAAD). The correlation between the expressions of TLE2 and other genes at the transcriptional level (FPKM) was estimated by Pearson’s correlation analysis. Genes exhibiting a strong positive or negative correlation with TLE2 expression were involved in the functional annotation analysis. Functional annotation analysis was performed with the clusterProfiler R package (version 3.7.0), gene set enrichment analysis (GSEA), and WEB-based GEne SeT AnaLysis Toolkit (WebGestalt; (http://bioinfo.vanderbilt.edu/webgestalt) (24).
Statistical analysis
In vitro experiments were performed in triplicate. Continuous variables were presented as mean ± SD (standard deviation). Student’s t-test was used for comparisons between two groups, and comparisons between more than three groups were conducted using one-way analysis of variance (ANOVA) with a post hoc least significant difference (LSD) test. Survival outcomes, including overall survival (OS) and disease-free survival (DFS), were compared by Kaplan–Meier curve analysis and the log-rank test. All statistical analyses were performed with SPSS 19.0 (SPSS Inc., Chicago, IL, USA) and R (version 3.7.0). A two-sided P value of <0.05 was considered as statistically significant.
Results
TLE2 was correlated with prognosis in PDAC patients
Survival analysis was performed to investigate the correlations between the expressions of TLE family members (TLE1, TLE2, TLE3, TLE4, and TLE5) and prognosis of human cancers. Our results revealed a certain degree of heterogeneity in the prognostic value of TLEs across different cancer types (Figure 1A,B). However, in PDAC patients, only increased TLE2 expression was significantly correlated with better overall survival (OS) (P<0.001) (Figure 1C) and disease-free survival (DFS) (P=0.022) (Figure 1D). In contrast, no correlation was found between the expression of TLE1, TLE3, and TLE4, and the prognosis of patients with PDAC (Figure 1E,F,G,H,I,J). Increased expression of TLE5 was correlated with improved OS (P=0.019) (Figure 1K), but not with improved DFS (P=0.59) in PDAC patients (Figure 1L). Further, we investigated the relation between TLE2 expression and clinicopathological parameters, including TNM stage and differentiation. As shown in Figure 1M, TLE2 expression was significantly associated with tumor stage (P<0.001) and tumor differentiation (P<0.001).
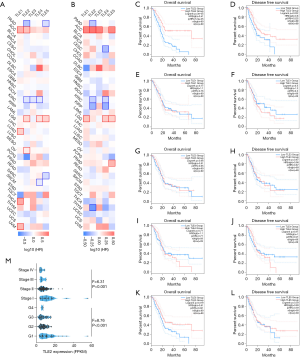
TLE2 expression affects the proliferation and cell cycle of PDAC cells
To further investigate the biological function of TLE2 in PDAC, the expression of TLE2 was investigated across various pancreatic cancer cell lines by qRT-PCR and western blotting. The MiaPaCa-2 cell line was found to have the highest expression of TLE2 among the six pancreatic cancer cell lines, while the PANC-1 cell line exhibited the lowest TLE2 expression level (Figure 2A,B). Consequently, TLE2 overexpression plasmid was constructed and introduced into PANC-1 cells. Also, siRNA oligonucleotides targeting TLE2 were transduced into MiaPaCa-2 cells to inhibit TLE2 expression, as validated by western blotting (Figure 2C).
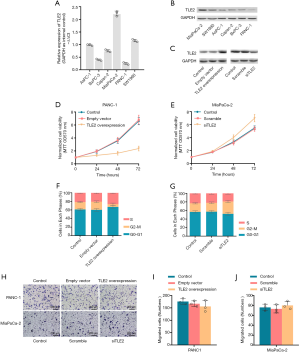
The effect of TLE2 expression on cell proliferation, invasion, and cell cycle were evaluated by MTT assay, Transwell assay, and flow cytometry, respectively. Our data revealed that the exogenous overexpression of TLE2 significantly inhibited the proliferation ability of PANC-1 cells (Figure 2D). In contrast, downregulation of TLE2 significantly promoted the cell proliferation of MiaPaCa-2 cells (Figure 2E). Interestingly, TLE2 overexpression induced delayed M-phase entry and S-phase accumulation (Figure 2F,G). However, no obvious effect on cell invasion was observed upon alternation of TLE2 expression (Figure 2H,I,J).
Gene function and pathway enrichment analysis
To elucidate the potential functional role of TLE2, functional annotation and enrichment analysis was carried out. The correlation between TLE2 and other genes at the transcriptional level was determined by Pearson’s correlation analysis. Next, we selected the 200 genes most positively correlated with TLE2 expression, as well as the 200 genes most negatively correlated with TLE2 expression, for further functional analysis (https://cdn.amegroups.cn/static/application/15d2d5448ba8a81d254cae02e0738b3e/atm-20-5492.pdf). By using clusterProfiler package, 6 Kyoto Encyclopedia of Genes and Genomes (KEGG) pathways were identified for the top 200 genes negatively correlated with TLE2 expression, including “Cell cycle”, “Oocyte meiosis”, “p53 signaling pathway”, “Proteasome”, “On carbon pool by folate”, and “Cellular senescence”, most of which are important pathways/mechanisms involved in the regulation of cell proliferation (Figure 3A).
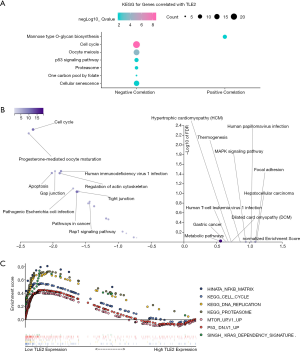
Furthermore, gene enrichment analysis using WEB-based GEne SeT AnaLysis Toolkit also demonstrated that the “Cell cycle” pathway was most significantly enriched in genes negatively correlated with TLE2, which indicated a close relationship between TLE2 and cell cycle (Figure 3B), and was consistent with our above in vitro finding. Moreover, GSEA analysis was performed using all of the gene sets in “oncogenic signatures” and “KEGG subset of Canonical pathways” obtained from the GSEA website (https://www.gsea-msigdb.org/gsea/msigdb). PDAC samples from the TCGA dataset were sorted according to expression of TLE2. As shown in Figure 3C, the enrichment results showed that several oncogenic pathways/mechanisms were enriched in samples with low TLE2 expression. The enrichment of “KEGG_Cell_Cycle” and “KEGG_DNA_Replication” pathways support the findings of our in vitro experiments and functional annotation analysis that TLE2 has an important role in the regulation of cell proliferation and cell cycle in pancreatic cancer cells. Moreover, the enrichment of Kras-related signaling pathways, NF-kb-related mechanisms, and mTOR-related gene sets in samples with low TLE2 expression further indicated the tumor suppressor role of TLE2.
Upregulation of TLE2 enhances the sensitivity to Gemcitabine in PDAC cells
The finding that the S-phase cell ratio was increased by TLE2 overexpression, as well as observations that the cell cycle pathway was enriched in PDAC samples with low TLE2 expression, impelled us to investigate the impact of TLE2 dysregulation on sensitivity to gemcitabine in pancreatic cancer cells. PANC-1 cells and MiaPaCa-2 cells were treated with different concentrations of gemcitabine. Through cell viability assays, we found that the cell viability of gemcitabine-treated PANC-1 cells was significantly inhibited after TLE2 overexpression (Figure 4A), suggesting that increased TLE2 expression was associated with gemcitabine sensitivity. In contrast, the proliferation capacity of MiaPaCa-2 cells treated with gemcitabine was increased after TLE2 silencing (Figure 4B). Overall, compared with the control group, TLE2 overexpression in PANC-1 cells significantly increased their sensitivity to gemcitabine, while knockdown of TLE2 substantially decreased the sensitivity.
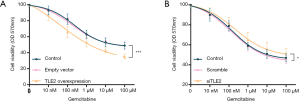
Discussion
In the present study, the role of TLE2 in PAAD was investigated. In silico data revealed that decreased TLE2 expression was associated with a poorer survival outcome in PDAC patients. In vitro experiments showed that TLE2 overexpression impaired the proliferation ability of pancreatic cells, and downregulation of TLE2 improved the proliferation capacity. Moreover, upregulation of TLE2 induced S-phase accumulation and sensitized pancreatic cells to gemcitabine. Functional annotation revealed TLE2 to be closely correlated with several important mechanisms involved in tumor development, particularly cell cycle regulation, which also explained the in vitro findings. Thus, we concluded that TLE2 acts as a tumor suppressor gene in PDAC.
Members of the TLE protein family have structural redundancy but act as repressors for a broad range of gene targets (7,25). Experimental studies have demonstrated that TLE proteins are critical to cell fate determination and influence the differentiation and development of tissue dramatically (26). In neurogenesis, TLE1 has been recognized as a key player participating in multiple mechanisms that repress neuronal differentiation (27-29). Mutation of the TLE1 gene has been discovered in patients with neurodevelopmental disorders, including postnatal microcephaly (27). During bone development, a profound decrease in bone mineralization was displayed in TLE4-deficient, revealing the essential role of TLE4 in the intrinsic regulation of bone development (30). In embryonic development, TLE1 has been shown to regulate the differentiation of chicken embryo fibroblasts through directly binding to the transcription factor Qin (31).
The significant involvement of TLEs in development indicates that they play a role in the regulation of cellular homeostasis and cell fate. Therefore, despite the effect of TLEs on normal cells, some studies have focused on their roles in human cancers. TLE4 acts as a tumor suppressor in leukemia through regulating Wnt-mediated inflammation (32). A large amount of evidence suggests that TLE1 plays a central role in the progression and pathophysiology of many cancers (33). Meanwhile, in prostate cancer, breast cancer, and colorectal cancer, TLE3 acts as a tumor suppressor (17,34,35). Experiments have also revealed that TLE4 acts as a tumor suppressor in myeloid leukemia and colorectal cancer through regulation of the Wnt and JNK/c-Jun pathways, respectively (32,36). Compared with the other TLEs, only recently was an association between TLE2 and cancer revealed. He et al. found that TLE2 could inhibit replication and transcription in Kaposi’s sarcoma-associated herpesvirus (37). Wu et al. identified TLE2 as an independent prognostic factor in patients with muscle invasive bladder cancer (38). As far as we know, there is currently very limited evidence identifying the role of TLE2 in other cancers, including PDAC.
The TLEs have been shown to regulate multiple steps during the development of the pancreas including differentiation of progenitor cells and endocrine cell maturation (18). With respect to pancreatic cancer, we reported for the first time the tumor suppressor role of TLE2 in PDAC. Both in vitro and in silico results demonstrated a close relationship between TLE2 and cell proliferation and cell cycle, which mimics the findings that other TLEs showed a strong growth regulation function in other cell systems. Additionally, multidrug resistance of PDAC cells hinders the effectiveness of chemotherapy, and the relationship between TLE2 and cell cycle inspired us to explore the effect of TLE2 on gemcitabine sensitivity in pancreatic cancer cells. Here, we provided in vitro evidence that upregulating TLEs could enhance the sensitivity of pancreatic cancer cell lines to gemcitabine, which could partly be explained by the specificity of gemcitabine for S-phase cells.
Although our results revealed the important role of TLE2 as a tumor suppressor in PDAC, there were some deficiencies in this study. First, the experiments were in vitro, therefore further in vivo experiments were needed to compensate for the in vitro evidence. Second, we focused on the toughest malignancy of pancreas, the PDAC; considering that the TLEs were widely involved in the regulation of pancreas development and differentiation, the role of TLEs other pancreatic tumors deserves further investigation. Last, our bioinformatic analysis revealed several key pathways associated with TLE2 expression in PDAC, further confirmation of these mechanisms via more in-depth researches is still required.
In summary, high expression of TLE2 is associated with improved survival in patients with PDAC. We found a negative correlation between TLE2 expression and proliferation capacity of PDAC cells, probably through cell cycle regulation. Moreover, our present study revealed that TLE2 expression was positively correlated with gemcitabine sensitivity in PDAC cells. Our study revealed the tumor suppressor role of TLE2 in PDAC for the first time. Although our findings have significant implications for therapeutic development in PDAC, the mechanism that mediates the tumor suppressor effect of TLE2 in PDAC demands further investigation.
Acknowledgments
Funding: None.
Footnote
Reporting Checklist: The authors have completed the MDAR reporting checklist. Available at http://dx.doi.org/10.21037/atm-20-5492
Data Sharing Statement: Available at http://dx.doi.org/10.21037/atm-20-5492
Conflicts of Interest: All authors have completed the ICMJE uniform disclosure form (available at http://dx.doi.org/10.21037/atm-20-5492). The authors have no conflicts of interest to declare.
Ethical Statement: The authors are accountable for all aspects of the work in ensuring that questions related to the accuracy or integrity of any part of the work are appropriately investigated and resolved. The cohort used in this study was based on public data (TCGA data); therefore, there was no need for ethical approval from our institution.
Open Access Statement: This is an Open Access article distributed in accordance with the Creative Commons Attribution-NonCommercial-NoDerivs 4.0 International License (CC BY-NC-ND 4.0), which permits the non-commercial replication and distribution of the article with the strict proviso that no changes or edits are made and the original work is properly cited (including links to both the formal publication through the relevant DOI and the license). See: https://creativecommons.org/licenses/by-nc-nd/4.0/.
References
- Wolfgang CL, Herman JM, Laheru DA, et al. Recent progress in pancreatic cancer. CA Cancer J Clin 2013;63:318-48. [Crossref] [PubMed]
- Strobel O, Neoptolemos J. Optimizing the outcomes of pancreatic cancer surgery. 2019;16:11-26.
- Mollinedo F, Gajate C. Novel therapeutic approaches for pancreatic cancer by combined targeting of RAF→MEK→ERK signaling and autophagy survival response. Ann Transl Med 2019;7:S153. [Crossref] [PubMed]
- Ierardi AM, Biondetti P, Coppola A, Fumarola EM, Biasina AM, Alessio Angileri S, Carrafiello G. Percutaneous microwave thermosphere ablation of pancreatic tumours. Gland Surg 2018;7:59-66. [Crossref] [PubMed]
- Grzenda A, Lomberk G, Zhang JS, et al. Sin3: master scaffold and transcriptional corepressor. Biochim Biophys Acta 2009;1789:443-50.
- Chinnadurai G. The transcriptional corepressor CtBP: a foe of multiple tumor suppressors. Cancer Res 2009;69:731-4. [Crossref] [PubMed]
- Li SS. Structure and function of the Groucho gene family and encoded transcriptional corepressor proteins from human, mouse, rat, Xenopus, Drosophila and nematode. Proc Natl Sci Counc Repub China B 2000;24:47-55. [PubMed]
- Chen G, Courey AJ. Groucho/TLE family proteins and transcriptional repression. Gene 2000;249:1-16. [Crossref] [PubMed]
- Ramakrishnan AB, Sinha A, Fan VB, et al. The Wnt Transcriptional Switch: TLE Removal or Inactivation? Bioessays 2018. [Crossref] [PubMed]
- Chodaparambil JV, Pate KT, Hepler MR, et al. Molecular functions of the TLE tetramerization domain in Wnt target gene repression. EMBO J 2014;33:719-31. [Crossref] [PubMed]
- Flack JE, Mieszczanek J, Novcic N, et al. Wnt-Dependent Inactivation of the Groucho/TLE Co-repressor by the HECT E3 Ubiquitin Ligase Hyd/UBR5. Mol Cell 2017;67:181-93.e5. [Crossref] [PubMed]
- Jennings BH, Ish-Horowicz D. The Groucho/TLE/Grg family of transcriptional co-repressors. Genome Biol 2008;9:205. [Crossref] [PubMed]
- Javed A, Guo B, Hiebert S, et al. Groucho/TLE/R-esp proteins associate with the nuclear matrix and repress RUNX (CBF(alpha)/AML/PEBP2(alpha)) dependent activation of tissue-specific gene transcription. J Cell Sci. 2000;113:2221-31. [PubMed]
- Yao X, Pham T, Temple B, et al. The Anoikis Effector Bit1 Inhibits EMT through Attenuation of TLE1-Mediated Repression of E-Cadherin in Lung Cancer Cells. PLoS One 2016;11:e0163228. [Crossref] [PubMed]
- Yao X, Pham T, Temple B, et al. TLE1 inhibits anoikis and promotes tumorigenicity in human lung cancer cells through ZEB1-mediated E-cadherin repression. Oncotarget 2017;8:72235-49. [Crossref] [PubMed]
- Ring BZ, Murali R, Soslow RA, et al. Transducin-Like Enhancer of Split 3 (TLE3) Expression Is Associated with Taxane Sensitivity in Nonserous Ovarian Carcinoma in a Three-Cohort Study. Cancer Epidemiol Biomarkers Prev 2018;27:680-8. [Crossref] [PubMed]
- Palit SA, Vis D, Stelloo S, et al. TLE3 loss confers AR inhibitor resistance by facilitating GR-mediated human prostate cancer cell growth. Elife 2019;8:e47430. [Crossref] [PubMed]
- Hoffman BG, Zavaglia B, Beach M, et al. Expression of Groucho/TLE proteins during pancreas development. BMC Dev Biol 2008;8:81. [Crossref] [PubMed]
- Roth M, Bonev B, Lindsay J, et al. FoxG1 and TLE2 act cooperatively to regulate ventral telencephalon formation. Development 2010;137:1553-62. [Crossref] [PubMed]
- Wu MF, Liao CY, Wang LY, et al. The role of Slit-Robo signaling in the regulation of tissue barriers. Tissue Barriers 2017;5:e1331155. [Crossref] [PubMed]
- Taciak B, Pruszynska I, Kiraga L, et al. Wnt signaling pathway in development and cancer. J Physiol Pharmacol 2018. [Crossref] [PubMed]
- Steinhart Z, Angers S. Wnt signaling in development and tissue homeostasis. Development 2018;145:dev146589. [Crossref] [PubMed]
- Tang Z, Li C, Kang B, et al. GEPIA: a web server for cancer and normal gene expression profiling and interactive analyses. Nucleic Acids Res 2017;45:W98-102. [Crossref] [PubMed]
- Liao Y, Wang J, Jaehnig EJ, et al. WebGestalt 2019: gene set analysis toolkit with revamped UIs and APIs. Nucleic Acids Res 2019;47:W199-205. [Crossref] [PubMed]
- Yao J, Liu Y, Husain J, et al. Combinatorial expression patterns of individual TLE proteins during cell determination and differentiation suggest non-redundant functions for mammalian homologs of Drosophila Groucho. Dev Growth Differ 1998;40:133-46. [Crossref] [PubMed]
- Metzger DE, Liu C, Ziaie AS, et al. Grg3/TLE3 and Grg1/TLE1 induce monohormonal pancreatic β-cells while repressing α-cell functions. Diabetes 2014;63:1804-16. [Crossref] [PubMed]
- Cavallin M, Maillard C, Hully M, et al. TLE1, a key player in neurogenesis, a new candidate gene for autosomal recessive postnatal microcephaly. Eur J Med Genet 2018;61:729-32. [Crossref] [PubMed]
- Buscarlet M, Hermann R, Lo R, et al. Cofactor-activated phosphorylation is required for inhibition of cortical neuron differentiation by Groucho/TLE1. PLoS One 2009;4:e8107. [Crossref] [PubMed]
- Stewart L, Stifani S. Dendritic localization of the transcriptional co-repressor Groucho/TLE1 in cortical and cerebellar neurons. Brain Res Mol Brain Res 2005;140:106-10. [Crossref] [PubMed]
- Wheat JC, Krause DS, Shin TH, et al. The corepressor Tle4 is a novel regulator of murine hematopoiesis and bone development. PLoS One 2014;9:e105557. [Crossref] [PubMed]
- Sonderegger CK, Vogt PK. Binding of the corepressor TLE1 to Qin enhances Qin-mediated transformation of chicken embryo fibroblasts. Oncogene 2003;22:1749-57. [Crossref] [PubMed]
- Shin TH, Brynczka C, Dayyani F, et al. TLE4 regulation of wnt-mediated inflammation underlies its role as a tumor suppressor in myeloid leukemia. Leuk Res 2016;48:46-56. [Crossref] [PubMed]
- Yuan D, Yang X, Yuan Z, et al. TLE1 function and therapeutic potential in cancer. Oncotarget 2017;8:15971-6. [Crossref] [PubMed]
- Peng LN, Deng XY, Gan XX, et al. Targeting of TLE3 by miR-3677 in human breast cancer promotes cell proliferation, migration and invasion. Oncol Lett 2020;19:1409-17. [PubMed]
- Liu L, Zhang Y, Wong CC, et al. RNF6 Promotes Colorectal Cancer by Activating the Wnt/β-Catenin Pathway via Ubiquitination of TLE3. Cancer Res 2018;78:1958-71. [Crossref] [PubMed]
- Wang SY, Gao K, Deng DL, et al. TLE4 promotes colorectal cancer progression through activation of JNK/c-Jun signaling pathway. Oncotarget 2016;7:2878-88. [Crossref] [PubMed]
- He Z, Liu Y, Liang D, et al. Cellular corepressor TLE2 inhibits replication-and-transcription- activator-mediated transactivation and lytic reactivation of Kaposi's sarcoma-associated herpesvirus. J Virol 2010;84:2047-62. [Crossref] [PubMed]
- Wu S, Nitschke K, Heinkele J, et al. ANLN and TLE2 in Muscle Invasive Bladder Cancer: A Functional and Clinical Evaluation Based on In Silico and In Vitro Data. Cancers (Basel) 2019;11:1840. [Crossref] [PubMed]
(English Language Editor: J. Reynolds)