Analysis of the strategy of LT4 prescribing and TSH monitoring for thyroid carcinoma after lobectomy
Introduction
Thyrotropin (TSH) suppression is a critical step in the management of DTC. A growing volume of data has suggested that there should not be a one-size-fits-all approach to suppression targets (1-4), and increasing emphasis is now placed on a more comprehensive assessment in which the risk of recurrence is balanced with the potential side effects (4,5). Studies have confirmed that increased TSH suppression for low-risk DTC (lr-DTC) patients does not improve prognosis (5-7). For lr-DTC patient, the current view is that the risk of mortality and recurrence is relatively low, and there is no convincing evidence that aggressive treatment is beneficial (8,9). Updated guidelines from the American Thyroid Association (ATA) recommend thyroid lobectomy as a reasonable surgical option for lr-DTC, including as an alternative to total thyroidectomy for patients with tumors <4 cm without extrathyroidal extension and other high-risk factors (10,11). The goals for post-operative TSH concentrations have also been changed from mild suppression (0.1–0.5 mIU/L) to levels in the mid to lower reference range (0.5–2.0 mIU/L). Consequently, for patients receiving lobectomy alone, the use of levothyroxine (LT4) for TSH suppression may not be necessary if TSH concentrations could be endogenously supported within the new target range (12).
Previously, we routinely prescribed LT4 for patients with DTC after lobectomy, achieving a mild suppression of TSH consistent with goals at that time (13). However, based on the recommendations for less aggressive TSH suppression, this strategy may require adjustment in lr-DTC patients. Evaluating the TSH trend after surgery is the essential step for adjusting the suppression therapy strategy. Studies have concluded that a percentage of patients have TSH values <2.0 mIU/L after lobectomy; however, few data are available to assess the individual changes in TSH and guide providers on the suitable initial dose of LT4 for patients in this cohort (14-16). The purpose of this study is to find out the factors affecting postoperative TSH suppression and to guide reasonable and standardized individualized TSH suppression according to the recommendations of the guidelines. Analyzing the individual TSH level could help us understand its changing trend.
Despite the uncertainty of TSH outcomes, TSH suppression is still routinely employed in many places. Although in clinical practice, total body weight is used to calculate the posology of LT4 to be administered for thyroid diseases, this study aims to determine when it is ideal and when it may be unnecessary to prescribe LT4. Lee et al. reported that TSH suppression is not necessary for patients with lr-DTC who undergo lobectomy. For Lr-DTC patients with TSH suppression after lobectomy, the high preoperative TSH level and the duration of TSH suppression are the key factors affecting the cessation of LT4 (17). However they did not tell us a definite evidence for guiding the choice of postoperative TSH suppression. We performed a retrospective study to analyze changes in TSH concentration before and after thyroid lobectomy in lr-DTC patients to whom we routinely provided an initial dose of LT4 (50 µg) after lobectomy, to evaluate the strategy of TSH monitoring and the initial dose of LT4 for obtaining the recently recommended TSH range in lr-DTC patients. We present the following article in accordance with the MDAR reporting checklist (available at http://dx.doi.org/10.21037/atm-20-4890).
Methods
Study subjects
The medical records of lr-DTC patients who underwent thyroid lobectomy at our hospital between January 1, 2015, and December 31, 2015, for DTC were retrospectively reviewed. All patients with lr-DTC, as defined by ATA risk classification who received lobectomy, were initially included (12). The exclusion criteria were: previous head or neck radiotherapy, chemotherapy, radioiodine, or thyroid surgery, hyperthyroidism, overt hypothyroidism, or preoperative LT4 supplementation. Subjects were also excluded if preoperative thyroid function testing was absent, or follow-up was less than 12 months.
Guided by standard clinical practice at the time, patients with lr-DTC after lobectomy did not undergo completion thyroidectomy or receive radioactive iodine ablation. None of the patients in this group were overweight. An initial dose of levothyroxine 50 µg to be taken daily more than 30 minutes before breakfast was prescribed, and patients underwent TSH assessments at 1 (1 MO), 3 (3 MO), 6 (6 MO), and 12 (12 MO) months postoperatively.
The collected data included demographic and histopathologic parameters, and TSH concentrations preoperatively (Pre-OP) and during follow-up. The presence of lymphocytic infiltration consistent with Hashimoto thyroiditis (HT) was noted on histopathologic evaluation. The dose adjustments in the lr-DTC patients and the reason for this were noted.
The study was conducted in accordance with the Declaration of Helsinki (as revised in 2013), and was approved by the Institutional Review Board of the First Hospital of China Medical University (No.: [2020]25-2). Because of the retrospective nature of the research, the requirement for informed consent was waived.
Laboratory measurements
The reference range for TSH was 0.35–4.94 mIU/L, free thyroxine (FT4) was 9.01–19.05 pmol/L, and free triiodothyronine (FT3) was 2.63–5.7 pmol/L. The intra- and inter-batch variability for TSH, FT4, and FT3 was <5%, <8%, and <7%, respectively. All measurements were performed using immuno-chemiluminescence assays (Abbott Company kit, Chicago, USA). The target range of TSH was set based on the 2015 ATA guidelines for DTC management and standard practice in our hospital’s laboratory. Postoperative TSH levels were defined as: <0.35 mIU/L (oversuppression), 0.35–2.0 mIU/L (at goal), ≥2.0 mIU/L (insufficient suppression). HT was diagnosed by surgical histopathology.
Statistical analysis
Demographic and clinical variables were compared using Student’s t-test or the Mann-Whitney U test as appropriate. The percentages of patients with oversuppression (<0.35 mIU/L), ideal suppression (0.35–2.0 mIU/L), or TSH elevation (≥2.0 mIU/L) were calculated to evaluate the efficacy of the empiric dose of LT4 50 µg daily initiated after lobectomy. Comparisons of TSH changes from the Pre-OP baseline to specified follow-up time points were made using a paired Student’s t-test or repeated measures ANOVA with Dunnett’s post-test. We performed an analysis of risk factors for TSH suppression using binary logistic regression for univariate and multivariate analysis. Pearson’s correlation coefficients were calculated for the TSH correlation between time points. Graphs were generated using GraphPad Prism Software version 5.0 (San Diego, CA, USA). All data were analyzed using a statistical software package (SPSS for Windows version 22.0; IBM Inc., Armonk, NY, USA), and a P value <0.05 represented statistical significance.
Result
Baseline information
A total of 124 patients with lr-DTC who received lobectomy were initially included. After the exclusion criteria was applied, 110 patients who had 12 MO follow-up were included in the analysis. The patients’ baseline characteristics are shown in Table 1. The mean age of the patients was 40.93±10.42 years, and 83/110 (75.5%) were female. The final histopathologic diagnoses were papillary thyroid carcinoma in all cases, of which 80/110 (72.7%) were papillary thyroid microcarcinoma (PTMC). Pathologic evidence of HT was found in 22/110 (20.0%). No postoperative complications occurred in our group.
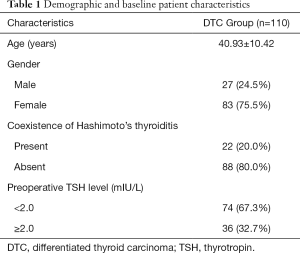
Full table
Individual changes in TSH concentration before and after surgery
All subjects received the initial dose of 50µg LT4 daily. Three patients received a dose reduction at 6 MO for symptoms of hyperthyroidism. No other dose adjustments were made in any other patients, leaving 107 patients for the analysis after 6 MO. The median TSH level Pre-OP was 1.63 mIU/L (range, 0.37–4.64 mIU/L), and 74/110 (67.3%) patients had a TSH <2.0 mIU/L. The median TSH level was 0.90 mIU/L (range, 0.01–3.93 mIU/L) at 1 MO, 0.38 mIU/L (range, 0.004–2.88 mIU/L) at 3 MO, 0.37 mIU/L (range, 0.01–3.26 mIU/L) at 6 MO, and 0.38 mIU/L (range, 0.04–2.53 mIU/L) at 12 MO. There was a significant decrease in TSH level from Pre-OP to 1 MO and 3 MO (P<0.001 for both; Figure 1A), but no significant differences were observed at 3 MO, 6 MO, or 12 MO.
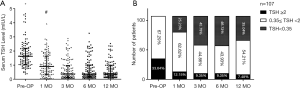
The distributions of postoperative TSH are shown in Figure 1B. Postoperatively, TSH concentration <2.0 mIU/L was present in 87.9%, 90.7%, 90.7%, and 92.5% of patients at 1 MO, 3 MO, 6 MO, and 12 MO, respectively. The distributions of TSH into the categories of <0.35 mIU/L, 0.35–2.0 mIU/L, or ≥2.0 mIU/L were similar at 3 MO, 6 MO, and 12 MO. Pearson correlation revealed a significant positive correlation between TSH at 3 MO and 12 MO (Pearson’s r2=0.484; P<0.0001; n=107; Figure 2A).
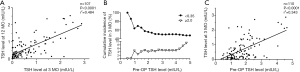
Changing trend in TSH category from Pre-OP to 3 MO
Since these data indicated 3 MO as a suitable time point to predict stable serum TSH level over the first year, further analyses utilized the TSH at 3 MO. Changes in the TSH category (<0.35, 0.35–2.0, or ≥2.0 mIU/L) in DTC patients from Pre-OP to 3 MO are shown in Table 2. At 3 MO, of the patients with a Pre-OP TSH ≥2.0 mIU/L, 18/36 (50%) patients fell into 0.35–2.0 mIU/L, only 7/36 (19.4%) continued to demonstrate a TSH level ≥2.0 mIU/L. In those with Pre-OP TSH between 0.35 to 2.0 mIU/L, 41/74 (55.4%) patients showed a TSH level <0.35 mIU/L, while 30/74 (43.2%) stayed in the same category. The cumulative incidence of patients within each TSH category at 3 MO is shown in Figure 2B. The cumulative incidence of TSH ≥2.0 mIU/L at 3 MO stabilized around 5% when Pre-OP TSH was no higher than 4.0 mIU/L and increased to 9.1% when Pre-OP TSH was higher than 4.0 mIU/L. The cumulative incidence of TSH <0.35 mIU/L at 3 MO decreased when Pre-OP TSH was no higher than 2.0 mIU/L and stabilized around 50% with Pre-OP TSH >2.0 mIU/L. Pearson’s correlation showed a significant positive correlation between Pre-OP TSH level and that in 3 MO (Pearson’s r2=0.343; P<0.0001; n=110) (Figure 2C).
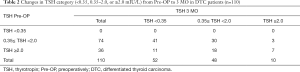
Full table
Risk factors of insufficient and over suppression
Logistic regression analysis was performed to identify risk factors for predicting a Post-OP (3 MO) TSH level ≥2.0 mIU/L (insufficient suppression) or <0.35 mIU/L (oversuppression). The relevant variables were age, gender, preoperative TSH, and the presence of HT. Preoperative TSH ≥2.0 mIU/L (OR, 6.185; 95% CI, 1.369–27.956; P=0.018) and presence of HT (OR, 4.786; 95% CI, 1.129–20.283; P=0.034) were significantly associated with postoperative TSH level ≥2.0 mIU/L (Table 3). Preoperative TSH <2.0 mIU/L (OR, 2.881; 95% CI, 1.167–7.114; P=0.022) was significantly associated with postoperative TSH level <0.35 mIU/L. The presence of HT (OR 0.180; 95% CI, 0.053–0.607; P=0.006) was also found to be negatively associated with the development of oversuppression in the univariate and multivariate analysis (Table 4).
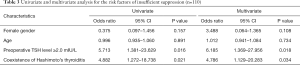
Full table
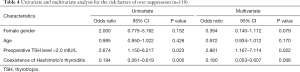
Full table
Discussion
Suppression of TSH after the initial therapy for differentiated thyroid carcinoma (DTC) has been advocated based on a reduction in the risk of recurrence and improved survival (2). More recently, however, studies have suggested adverse effects of LT4-induced TSH suppression (12,18-20,21), and increasing data have shown that such suppression may not achieve improved outcomes for lr-DTC patients (1,5,21-23). Therefore, treatment recommendations emphasize balancing the risk of tumor recurrence with possible side effects and advocate less stringent TSH goals within the low reference range (0.5–2.0 mIU/L) for lr-DTC patients (4,12). Since the remaining thyroid may maintain serum TSH within this target range after lobectomy, LT4 therapy may not be necessary (24) and the frequency at which LT4 is required, factors associated with LT4 requirement, the dose needed, and TSH stability achieved by initial dosing are not well understood in this context. Such information is vital, because surgical treatment with lobectomy alone for lr-DTC is considered to be appropriate not only for microcarcinoma (size <1 cm), but also for larger tumors 1–4 cm (12). Cox et al. reported that the TSH level rose to >2.0 mIU/L within a year in more than 70% of patients after lobectomy, which demonstrated the need for LT4 after lobectomy; however, no analysis of individual TSH trends was performed (14).
In this retrospective cohort study, we similarly found that 3 MO is a more appropriate time point than 1 MO for initially monitoring TSH after lobectomy and that TSH levels were stable between 3 and 12 MO. Measurement of TSH at 1 MO appeared too early to determine the 3MO TSH concentration. Our finding is important because surgeons do check for TSH early, and this could be a clinical recommendation to change practice and not check at that time point. It will help us make a better strategy for active monitoring. With a first dose of LT4 50 µg daily, 45% of these patients achieved the appropriate TSH suppression range (0.35–2.0 mIU/L). A preoperative TSH ≥2.0 mIU/L and the presence of HT were risk factors of insufficient suppression. Similar to our data, Ahn et al. reported that preoperative TSH ≥2.0 mIU/L and Hashimoto's thyroiditis are independent risk factors for hypothyroidism in patients after lobectomy during the mean follow-up period of 56.4 months (15). Lee et al. reported that preoperative TSH was >2.5 mIU/L, and positive thyroid microsomal antibody may show the need for LT4 suppression after lobectomy (25).
Our data may be valuable when attempting to achieve appropriate postoperative TSH concentrations for lr-DTC patients following the 2015 ATA guidelines. Patients with a preoperative TSH ≥2.0 mIU/L and/or HT will require postoperative LT4, and the initial dose of 50 µg may prove inadequate. The initial dose of 75 µg may be more suitable for these patients. Conversely, since oversuppression (TSH <0.35 mIU/L) was more frequent in lr-DTC patients with Pre-OP TSH <2.0 mIU/L and the absence of HT, LT4 may not be prescribed to these patients.
The current study has several limitations, including a lack of comparison with untreated lr-DTC patients and the relatively small sample size. Also, HT was defined by histopathology and not by TPO-Ab, so direct assessment of HT as a pre-operative risk factor could not be made. However, this is the first study to evaluate the first dose and describe the details of individual trends of TSH change after lobectomy. Strict conformity with standard clinical practice with very few dose adjustments limited selection bias and enhanced the significance of this study.
Conclusions
In conclusion, initial TSH assessment might be performed at three months after lobectomy, as it predicts stable serum TSH levels over the first year. An initial dose of LT4 50 µg daily is adequate to maintain TSH lower than 2.0 mIU/L for more than 90% patients, but those with preoperative TSH ≥2.0 mIU/L and/or coexistent HT are more likely to show inadequate suppression. Initial LT4 treatment might not be prescribed for patients with Pre-OP TSH <2.0 mIU/L and the absence of HT.
Acknowledgments
Funding: Natural Science Foundation of Liaoning Province (grant number 2020-MS-143). ZW and HG received a scholarship from the China Scholarship Council (grant number 201608210371 and 201708210108).
Footnote
Reporting Checklist: The authors have completed the MDAR reporting checklist. Available at http://dx.doi.org/10.21037/atm-20-4890
Data Sharing Statement: Available at http://dx.doi.org/10.21037/atm-20-4890
Conflicts of Interest: All authors have completed the ICMJE uniform disclosure form (available at http://dx.doi.org/10.21037/atm-20-4890). The authors have no conflicts of interest to declare.
Ethical Statement: The authors are accountable for all aspects of the work in ensuring that questions related to the accuracy or integrity of any part of the work are appropriately investigated and resolved. The study was conducted in accordance with the Declaration of Helsinki (as revised in 2013), and was approved by the Institutional Review Board of the First Hospital of China Medical University (NO.: [2020]25-2). Because of the retrospective nature of the research, the requirement for informed consent was waived.
Open Access Statement: This is an Open Access article distributed in accordance with the Creative Commons Attribution-NonCommercial-NoDerivs 4.0 International License (CC BY-NC-ND 4.0), which permits the non-commercial replication and distribution of the article with the strict proviso that no changes or edits are made and the original work is properly cited (including links to both the formal publication through the relevant DOI and the license). See: https://creativecommons.org/licenses/by-nc-nd/4.0/.
References
- Jonklaas J, Sarlis NJ, Litofsky D, et al. Outcomes of patients with differentiated thyroid carcinoma following initial therapy. Thyroid 2006;16:1229-42. [Crossref] [PubMed]
- McGriff NJ, Csako G, Gourgiotis L, et al. Effects of thyroid hormone suppression therapy on adverse clinical outcomes in thyroid cancer. Ann Med 2002;34:554-64. [Crossref] [PubMed]
- Diessl S, Holzberger B, Mäder U, et al. Impact of moderate vs stringent TSH suppression on survival in advanced differentiated thyroid carcinoma. Clin Endocrinol (Oxf) 2012;76:586-92. [Crossref] [PubMed]
- Biondi B, Cooper DS. Benefits of thyrotropin suppression versus the risks of adverse effects in differentiated thyroid cancer. Thyroid 2010;20:135-46. [Crossref] [PubMed]
- McLeod DS, Sawka AM, Cooper DS. Controversies in primary treatment of low-risk papillary thyroid cancer. Lancet 2013;381:1046-57. [Crossref] [PubMed]
- Cooper DS, Specker B, Ho M, et al. Thyrotropin suppression and disease progression in patients with differentiated thyroid cancer: results from the National Thyroid Cancer Treatment Cooperative Registry. Thyroid 1998;8:737-44. [Crossref] [PubMed]
- Grani G, Ramundo V, Verrienti A, et al. Thyroid hormone therapy in differentiated thyroid cancer. Endocrine 2019;66:43-50. [Crossref] [PubMed]
- Hay ID, Johnson TR, Kaggal S, et al. Papillary Thyroid Carcinoma (PTC) in Children and Adults: Comparison of Initial Presentation and Long-Term Postoperative Outcome in 4432 Patients Consecutively Treated at the Mayo Clinic During Eight Decades (1936-2015). World J Surg 2018;42:329-42. [Crossref] [PubMed]
- Lamartina L, Leboulleux S, Terroir M, et al. An update on the management of low-risk differentiated thyroid cancer. Endocr Relat Cancer 2019;26:R597-R610. [Crossref] [PubMed]
- Kim SK, Park I, Woo JW, et al. Total thyroidectomy versus Lobectomy in conventional papillary thyroid microcarcinoma: Analysis of 8,676 patients at a single institution. Surgery 2017;161:485-92. [Crossref] [PubMed]
- Kwon H, Jeon MJ, Kim WG, et al. Comparison of Lobectomy and total thyroidectomy in patients with papillary thyroid microcarcinoma: a retrospective individual risk factor-matched cohort study. Eur J Endocrinol 2017;176:371-8. [Crossref] [PubMed]
- Haugen BR, Alexander EK, Bible KC, et al. 2015 American Thyroid Association Management Guidelines for Adult Patients with Thyroid Nodules and Differentiated Thyroid Cancer: The American Thyroid Association Guidelines Task Force on Thyroid Nodules and Differentiated Thyroid Cancer. Thyroid 2016;26:1-133. [Crossref] [PubMed]
- American Thyroid Association (ATA) Guidelines Taskforce on Thyroid Nodules and Differentiated Thyroid Cancer, Cooper DS, Doherty GM, et al. Revised American Thyroid Association management guidelines for patients with thyroid nodules and differentiated thyroid cancer. Thyroid 2009;19:1167-214. [Crossref] [PubMed]
- Cox C, Bosley M, Southerland LB, et al. Lobectomy for treatment of differentiated thyroid cancer: can patients avoid postoperative thyroid hormone supplementation and be compliant with the American Thyroid Association guidelines? Surgery 2018;163:75-80. [Crossref] [PubMed]
- Ahn D, Sohn JH, Jeon JH. Hypothyroidism Following Lobectomy: Incidence, Risk Factors, and Clinical Characteristics. J Clin Endocrinol Metab 2016;101:1429-36. [Crossref] [PubMed]
- Park S, Jeon MJ, Song E, et al. Clinical Features of Early and Late Postoperative Hypothyroidism After Lobectomy. J Clin Endocrinol Metab 2017;102:1317-24. [Crossref] [PubMed]
- Lee YM, Jeon MJ, Kim WW, et al. Optimal Thyrotropin Suppression Therapy in Low-Risk Thyroid Cancer Patients after Lobectomy. J Clin Med 2019;8:1279. [Crossref] [PubMed]
- Klein Hesselink EN, Klein Hesselink MS, de Bock GH, et al. Long-term cardiovascular mortality in patients with differentiated thyroid carcinoma: An observational study. J Clin Oncol 2013;31:4046-53. [Crossref] [PubMed]
- Kim MK, Yun KJ, Kim MH, et al. The effects of thyrotropin-suppressing therapy on bone metabolism in patients with well-differentiated thyroid carcinoma. Bone 2015;71:101-5. [Crossref] [PubMed]
- Tournis S, Antoniou JD, Liakou CG, et al. Volumetric bone mineral density and bone geometry assessed by peripheral quantitative computed tomography in women with differentiated thyroid cancer under TSH suppression. Clin Endocrinol (Oxf) 2015;82:197-204. [Crossref] [PubMed]
- Wang LY, Smith AW, Palmer FL, et al. Thyrotropin suppression increases the risk of osteoporosis without decreasing recurrence in ATA low- and intermediate-risk patients with differentiated thyroid carcinoma. Thyroid 2015;25:300-7. [Crossref] [PubMed]
- Nikiforov YE, Seethala RR, Tallini G, et al. Nomenclature revision for encapsulated follicular variant of papillary thyroid carcinoma: a paradigm shift to reduce overtreatment of indolent tumors. JAMA Oncol 2016;2:1023-9. [Crossref] [PubMed]
- Carhill AA, Litofsky DR, Ross DS, et al. Long-Term outcomes following therapy in differentiated thyroid carcinoma: NTCTCS registry analysis 1987-2012. J Clin Endocrinol Metab 2015;100:3270-9. [Crossref] [PubMed]
- Vaisman F, Shaha A, Fish S, Michael Tuttle R. Initial therapy with either thyroid Lobectomy or total thyroidectomy without radioactive iodine remnant ablation is associated with very low rates of structural disease recurrence in properly selected patients with differentiated thyroid cancer. Clin Endocrinol (Oxf) 2011;75:112-9. [Crossref] [PubMed]
- Lee DY, Seok J, Jeong WJ, Ahn SH. Prediction of thyroid hormone supplementation after thyroid Lobectomy. J Surg Res 2015;193:273-8. [Crossref] [PubMed]
(English Language Editors: J. Reynolds and J. Chapnick)