Vitexin attenuates epithelial ovarian cancer cell viability and motility in vitro and carcinogenesis in vivo via p38 and ERK1/2 pathways related VEGFA
Introduction
Epithelial ovarian cancer (EOC) is a serious type of ovarian tumor that occurs worldwide and affects 3–12/100,000 women per year. EOC is characterized by high mortality rates and there is no effective routine treatment for the advanced stages of this disease (1-3). The leading cause of relapse and death in EOC patients is chemoresistance. Novel and adjuvant therapies have therefore become the new direction of research for EOC treatment.
Blood vessels are the impetus for the rapid growth and metastasis of cancer cells. Neovascularization therefore plays a critical supporting role in the occurrence and development of tumors, and a variety of signaling molecules are involved in its regulation (4,5). Vascular endothelial growth factor (VEGF) is the primary factor that induces endothelial cell proliferation and is closely associated with tumor tissue angiogenesis. VEGFA is a member of the VEGF family, which exert their biological effect when bound to their receptors. The primary VEGF receptors include Flt-4, VEGFR2, and Flt-1 (6,7). VEGFR, VEGF, and their downstream signaling pathways are markers of tumor angiogenesis, and VEGFA/VEGFR2 signal transduction plays a dominant role in vascular endothelial cell formation (8,9). VEGFA/VEGFR2 signal transduction inhibition can therefore suppress tumor cell growth by blocking angiogenesis (10,11).
Lignans, a class of complex polyphenolic antioxidants found in plants, were once considered one of the most promising dietary agents for cancer prevention (12,13). One type of lignan compound is vitexin (chemical structure: Figure 1A), which is isolated from the seed of Vitex negundo. Zhou et al. reported that vitexin exerts extensive antitumor activity in cancer xenograft models, such as inducing caspase activation and promoting apoptosis (14). Numerous evidence demonstrates that vitexin exerts antitumor effects on multiple human cancers, including glioblastoma (15), hepatocellular carcinoma (16), and leukemia (17). It remains unknown whether vitexin possesses antitumor effects on EOC.
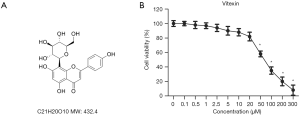
In this study, we investigated the suppression effect of vitexin on EOC cell viability and motility in vitro and carcinogenesis in vivo.
We present the following article in accordance with the ARRIVE reporting checklist (available at http://dx.doi.org/10.21037/atm-20-5586).
Method
Cell culture
The ovarian cancer cell line SKOV-3 was purchased from the Cell Bank of the Chinese Academy of Science (Shanghai, China). Cells were cultivated in complete medium (Gibco, Rockville, MD, USA) with T75 cell culture flasks (Corning, New York, NY, USA) according to the suppliers’ instructions.
Animal model
Athymic nude mice (female) were obtained from laboratories of The First Affiliated Hospital of Xinxiang Medical University. The mice were housedin climate-controlled conditions and fed with free access to chow and water. The environment of the animals was a 12-hour light/dark cycle with a constant temperature of 22±1 °C and humidity of 55%±5%. Tumorigenic assays were performed as described in previous studies (18). SKOV-3 cells were transduced with lentiviral shRNA and treated with puromycin (1.5 mg/mL) for 7 days to select the transduced cells. Transduced cells with a density of 5×106 cells/well we-4re injected subcutaneously into the right thighs of mice to set up the model. Upon tumor formation, the mice were injected intraperitoneally with vitexin (20, 40, or 80 mg/kg) and solvent twice weekly for 4 weeks. The dosage of Vitexin was based on the previous study (19). Tumor sizes were measured weekly, and the mice were sacrificed after 4 weeks.
All animal experiments were carried out in accordance with the NIH Guide for the Care and Use of Laboratory Animals 2018 and were approved by The First Affiliated Hospital of Xinxiang Medical University, Weihui, Henan.
Cell viability assay
Two hundred µL SKOV-3 cells were plated in 96-well plates at a density of 5×103 cells/well. Upon 24-hour culture, the cells were treated with different concentrations of vitexin (0, 0.1, 0.5, 1, 2.5, 5, 10, 20, 50, 100, 200, 300, and 400 µL) for 4 hours in a 5% CO2 incubator at 37 °C. CCK-8 dye was then added to the medium and the cells were incubated at 37 °C for 4 hours. Finally, the absorbance in five parallel wells was measured.
Colony formation assay
SKOV-3 cells at a density of 5×103 cells/well were seeded in triplicate in 6-well plates overnight. After 10 days of culture, visible cells were washed with phosphatebuffered saline (PBS), fixed with methanol, and then stained with Giemsa. Colonies of N50 cells were counted (20).
Flow cytometry
The cell apoptosis rate was measured by flow cytometry; this process is described in previous literature (21). Briefly, after treatment, cells were washed with PBS and incubated with 10 µL Annexin V-FITC (fluorescein isothiocyanate) and 5 µL propidium iodine (PI) for 15 min at room temperature in the dark. Finally, cells were analyzed using a FACSCalibur Flow Cytometer (BD Biosciences).
Western blot analysis
Cells were harvested after 48 hours of vitexin pretreatment. Western blot analyses were carried out according to processes described in previous literature (22). The primary antibodies used in this study were as follows: VEGF (ab32152, 1:250), MMP-9 (ab76003, 1:1,000), E-cadherin (ab76055, 1:1,000), PCNA(ab29, 1:1,000), MMP-14(ab51074, 1:5,000), caspase-3 (ab13847, 1:500), VEGFA (ab52917, 1:10,000), ERK1/2 (b17942, 1:1,000), Ki67 (ab15580, 1:500), p-ERK1/2 (ab214362, 1:1,000), p38 (ab31828, 1:1,000), and p-p38 (ab4822, 1:1,000). The samples were analyzed using an Odyssey Infrared Imaging System (LI-COR Inc., Lincoln, NE, USA).
Cell apoptosis assay
Hoechst 33258 staining was used to detecte apoptosis. In brief, 3×105 cells per well were washed with PBS twice. Then, 1 mL of Hoechst 33258 reagent (Beyotime, Nantong, China) was added to each well, and the cells were incubated at 37 °C for 30 min in the dark. Then, the Hoechst 33258 reagent was removed, and cells were washed with PBS for 3 times (5 min × 3 times). Morphological changes of apoptotic cells were observed under an inverted fluorescence microscope, and images were captured.
Transwell assay
Cells were left either untreated or treated with vitexin for 48 hours. Cell suspensions of 200 mL/chamber in serum-free medium were then seeded into the upper chambers of transwell inserts. Complete medium was added to the lower chambers. After a 36-hour incubation period, cells were fixed with methanol and stained with Giemsa. Cells on the upper surface of the membrane were then cleared, allowing cells on the lower surface to be examined by microscope (Leica, Germany). The average number of invasive cells was recorded.
Microtubule formation assay
Cells were left either untreated or treated with vitexin for 48 hours. Serum-free RPMI medium and matrigel were then added and mixed in a ratio of 1:1 at 4 °C. The mixture was added to the 24-well plates in an amount of 300 µL/well and incubated at 37 °C under 5% CO2 conditions for 30 minutes. The microtubule structures were observed every 4 hours. The images were analyzed with Microvision Saisam software.
Transferase dUTP nick end labeling (TUNEL) assay
The prepared paraffin sections were dewaxed, rehydrated, and washed. Then, the sections were incubated with TUNEL reaction mixture (Roche Diagnostics, Indianapolis, IN, USA) according to the instructions of the manufacturer. Apoptotic cells were detected by fluorescence microscopy (Olympus, Japan).
Immunohistochemistry
Prior to cutting tumor tissue into 3 µm-thick sections, the tissue was fixed with 4% formalin and paraffin embedding. The sections were then routinely deparaffinized in xylene and rehydrated in an alcohol gradient. Sections were sealed with 5% normal goat serum for 1 hour and co-incubated with primary antibodies Ki67 (ab15580, 1:500) and VEGF (ab32152, 1:250) overnight at 4 °C. Sections were then washed three times in PBS and incubated with secondary antibody (ZSGB-BIO, Beijing, China) for 30 minutes at room temperature. Sections were then analyzed using an Olympus BX-UCB light-field microscope according to the manufacturer’s instructions.
Statistical analysis
Analyses were performed using SPSS 21.0 (SPSS, Inc., Chicago, IL, USA). ANOVA and Student’s t-tests were performed to assess statistical significance, as appropriate. Data are presented as mean ± SEM.
Results
Effect of vitexin treatment on SKOV-3 cell viability
Vitexin solutions of varying concentrations (0, 0.1, 0.5, 1, 2.5, 5, 10, 20, 50, 100, 200, 300, and 400 µL) were used to treat SKOV-3 cells and determine the optimal therapeutic dose. Upon 24-hour treatment, compared with the control group cells, vitexin concentrations of 0.1–20 µL caused minimal toxicity to SKOV-3 cells, while concentrations above 20 µL caused significant toxicity (Figure 1B). Three dose concentrations (5, 10, and 20 µL) were selected for use in subsequent experiments in this study.
Vitexin treatment impaired SKOV-3 cell proliferation and promoted SKOV-3 cell apoptosis
As shown in Figure 2A, compared with no vitexin treatment, 5, 10, and 20 µL vitexin treatment significantly suppressed the colony forming rate of SKOV-3 cells. Compared with the control group, treatment of 10 µL Vitexin enhanced tumor cell apoptosis rate (2.6%±0.6% versus 14.6%±6.0%, *P<0.05, Figure 2B). Figure 2C showed that Ki67 expressed highly in SKOV-3 cells, which is consistent with previous report (23). Of note, compared with the control group, 10 µL of vitexin significantly reduced Ki67 levels (0.08%±0.02% versus 0.02%±0.01%, *P<0.05) and accelerated caspase-3 activation. These results suggest that vitexin treatment can greatly impair SKOV-3 cell proliferation and promote SKOV-3 cell apoptosis.
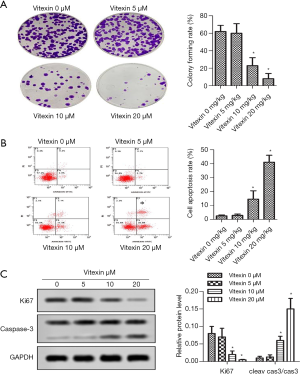
Vitexin treatment reduced SKOV-3 cell invasive ability
Typical pictures of transwell assay was showed in Figure 3A. From Figure 3B, 10 and 20 µL vitexin treatment could significantly decrease the number of invasive cells per field, which revealed that the invasive ability of SKOV-3 cells were suppressed by vitexin treatment. Typical pictures of microtubule formation assay was showed in Figure 3C. From Figure 3D, 10 and 20 µL vitexin treatment could significantly decrease the formation of microtubule, which indicated that the microtubule formation ability of SKOV-3 cells were suppressed by vitexin treatment. In order to make the results more reliable, we used Western blot to detect the relative content of proteins VEGF, MMP-9 and E-cadherin, which related to the microtubule formation and invasion of cancer cells. The results was showed in Figure 3E,F. The data indicated that 10 and 20 µL vitexin treatment could significantly downregulate VEGF and MMP-9 expression, and upregulate E-cadherin expression. These results indicate that vitexin treatment can inhibit SKOV-3 cell invasion and reduce angiogenesis. This suggests that vitexin has the potential to inhibit tumor cell migration and proliferation.
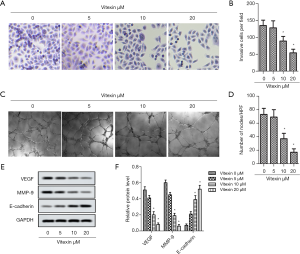
Vitexin treatment attenuated the viability and motility of SKOV-3 cells via VEGFA-dependent ERK1/2 and p38
As shown in Figure 4A, vitexin treatment greatly downregulated the expression of VEGFA and VEGFR2 simultaneously, which in turn downregulated the phosphorylation of ERK1/2 and p38. The relative protein levels of VEGFA, VEGFR2, p-ERK1/2/ERK1/2, and p-p38/p38 decreased significantly (Figure 4A). To further determine the effect of Vitexin treatment on ERK1/2 and p38 pathways, skatole, the activator of ERK1/2 and p38 pathways, was introduced. From Figure 4B,C,D,E,F, skatole treatment partially attenuated the inhibitory effect of Vitexin on the proliferation and invasion of SKOV-3 cells, and counteracted the promoting effect of Vitexin on the apoptosis of SKOV-3 cells. These results suggest that vitexin treatment attenuated the viability and motility of SKOV-3 cells through VEGFA-dependent ERK1/2 and p38.
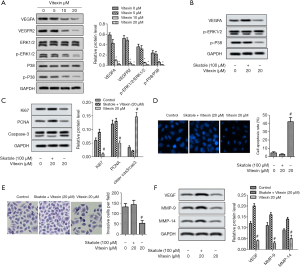
Vitexin treatment suppressed tumor formation in vivo
Vitexin notably inhibited tumor growth (Figure 5A). Tumor weight decreased greatly with increased vitexin dose (Figure 5B). As shown in Figure 5C, vitexin treatment clearly attenuated tissue apoptosis. Figure 5D,E show that vitexin treatment reduced the percentage of apoptotic cells and downregulated VEGF expression in tumor tissues. These results suggest that vitexin inhibited tumor formation in vivo.
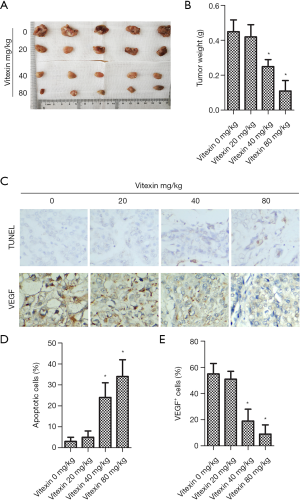
Discussion
Chemotherapy is currently the primary treatment for cancer. This treatment, however, produces strong and unpleasant side effects. Natural products, especially compounds that originate in plants, have recently attracted the attention of researchers due to their potential antitumor properties, and Chinese medicine is now being researched as adjuvant treatment for cancer and other malignant diseases. Numerous studies have shown that Chinese medicine can effectively decrease the side effects and toxicity of chemotherapy, as well as enhance disease resistance and promote apoptosis (24,25). Vitexin, which is isolated from the seed of Vitex negundo, has potential antitumor activity against many human cancers. For example, it can exert cytotoxic effects by inducing apoptosis mediated by the activation of caspases (26). Vitexin has also been shown to inhibit the growth of esophageal cancer cells and induce their apoptosis (27). In this study, we found that low doses of vitexin (≤20 µL) had minimal toxicity on SKOV-3 cells. Vitexin treatment impaired the proliferation of SKOV-3 cells and promoted their apoptosis in vitro, as well as attenuating cell invasion and microtubule formation. Interestingly, vitexin treatment greatly downregulated the expression of VEGFA and VEGFR2 simultaneously. Additionally, vitexin treatment markedly inhibited ERK1/2 and p38 phosphorylation. Vitexin treatment also suppressed tumor formation in vivo.
A typical feature of tumor cells is clonal proliferation. Ki67 is an important indicator of cancer cell proliferation and is a powerful prognostic marker for several types of cancer (28). As apoptosis is a necessary cell death program, activation of this process is an effective anticancer pathway (29). Caspase-3 is an important part of the apoptotic process. In the present study, Ki67 expression was significantly decreased in SKOV-3 cells treated with vitexin, while the cleavage of caspase-3 and caspase-9 were simultaneously enhanced. Bhardwaj et al. reported that vitexin produces good pharmacological effects by inducing the apoptosis of rectal cancer cells (30). Consistent with our results, in this study vitexin treatment induced the cleavage of caspase-9 and caspase-3. This suggests that vitexin may inhibit SKOV-3 cell proliferation and promote SKOV-3 cell apoptosis.
Tumor metastasis is the leading cause of cancer-related death and infiltration plays a critical role in this process. Infiltration involves tumor cells losing their intercellular junctions, followed by cell degradation, remodeling, adhering to the surrounding extracellular matrix (ECM), and finally migrating to distant locations. In our study, transwell assays and microtubule formation assays showed that vitexin played a positive role in preventing tumor cell invasion and microtubule formation, respectively. Consistent with our results, Choi et al. found that vitexin suppressed the rate of invasion and migration of PC12 cells (31). This suggests that vitexin can suppress the invasive ability of SKOV-3 cells.
Tumor growth is closely related with oxygen and blood supply provided by new blood vessels (32). This process is mediated in many tumors by VEGFA, which is a highly specific pro-vascular endothelial growth factor that accelerates increased vascular permeability, extracellular matrix degeneration, vascular endothelial cell migration, proliferation, and angiogenesis. In the present study, vitexin treatment downregulated VEGFA and VEGFR2 expression and inhibited ERK1/2 and p38 phosphorylation. Scarpa et al. found that vitexin-2-O-xylocides (XYXs) and avenanthramides (AVNs) downregulated the expression of VEGFA, which resulted in the inhibition of CaCo-2 and HepG2 cell proliferation (33). Our findings are also in agreement with Rosa et al. These researchers reported that vitexin reduced the expression of p-p38 and p-ERK1/2 in LPS-elicited RAW 264.7 cells (34). Curtarello et al. exposed ovarian cancer cells to anti-VEGF therapy and achieved good results (35). Together, these results indicated that vitexin influenced SKOV-3 cells by affecting VEGFA and VEGFR2 expression, and the related pathways may be ERK1/2 and p38.
To further verify the mitigation effect of vitexin on EOC, we established a xenotransplantation mouse model for experimentation. Our results showed that vitexin treatment significantly inhibited both tumor growth and the percentage of VEGF positive cells while significantly promoting tissue apoptosis.
In conclusion, we found that vitexin treatment inhibited SKOV-3 cell invasion and promoted SKOV-3 cell apoptosis. Vitexin treatment downregulated the expression of VEGFA and VEGFR2, and inhibited the phosphorylation of ERK1/2 and p38. Vitexin also significantly inhibited tumor growth and promoted tumor tissue apoptosis in vivo.
Acknowledgments
Funding: None.
Footnote
Reporting Checklist: The authors have completed the ARRIVE reporting checklist. Available at http://dx.doi.org/10.21037/atm-20-5586
Data Sharing Statement: Available at http://dx.doi.org/10.21037/atm-20-5586
Conflicts of Interest: All authors have completed the ICMJE uniform disclosure form (available at http://dx.doi.org/10.21037/atm-20-5586). The authors have no conflicts of interest to declare.
Ethical Statement: The authors are accountable for all aspects of the work in ensuring that questions related to the accuracy or integrity of any part of the work are appropriately investigated and resolved. All animal experiments were carried out in accordance with the NIH Guide for the Care and Use of Laboratory Animals 2018 and were approved by The First Affiliated Hospital of Xinxiang Medical University, Weihui, Henan (No. HNXX-20191103).
Open Access Statement: This is an Open Access article distributed in accordance with the Creative Commons Attribution-NonCommercial-NoDerivs 4.0 International License (CC BY-NC-ND 4.0), which permits the non-commercial replication and distribution of the article with the strict proviso that no changes or edits are made and the original work is properly cited (including links to both the formal publication through the relevant DOI and the license). See: https://creativecommons.org/licenses/by-nc-nd/4.0/.
References
- Lheureux S, Gourley C, Vergote I, et al. Epithelial ovarian cancer. Lancet 2019;393:1240-53. [Crossref] [PubMed]
- Flores I, Hevia D, Tittarelli A, et al. Dendritic Cells Loaded with Heat Shock-Conditioned Ovarian Epithelial Carcinoma Cell Lysates Elicit T Cell-Dependent Antitumor Immune Responses In Vitro. J Immunol Res 2019;2019:9631515. [Crossref] [PubMed]
- Xue C, Zhu D, Chen L, et al. Expression and prognostic value of PD-L1 and PD-L2 in ovarian cancer. Transl Cancer Res 2019;8:111-9. [Crossref]
- Sakurai T, Kudo M. Signaling pathways governing tumor angiogenesis. Oncology 2011;81 Suppl 1:24-9. [Crossref] [PubMed]
- Carmeliet P, Jain RK. Molecular mechanisms and clinical applications of angiogenesis. Nature 2011;473:298-307. [Crossref] [PubMed]
- Robinson CJ, Stringer SE. The splice variants of vascular endothelial growth factor (VEGF) and their receptors. J Cell Sci 2001;114:853-65. [PubMed]
- Alexander M, Halmos B. VEGF inhibitors in EGFR-mutated lung cancer: a never-ending story? Ann Transl Med 2018;6:446. [Crossref] [PubMed]
- Chatterjee S, Heukamp LC, Siobal M, et al. Tumor VEGF:VEGFR2 autocrine feed-forward loop triggers angiogenesis in lung cancer. J Clin Invest 2013;123:1732-40. [Crossref] [PubMed]
- Hamerlik P, Lathia JD, Rasmussen R, et al. Autocrine VEGF-VEGFR2-Neuropilin-1 signaling promotes glioma stem-like cell viability and tumor growth. J Exp Med 2012;209:507-20. [Crossref] [PubMed]
- Lu KV, Chang JP, Parachoniak CA, et al. VEGF inhibits tumor cell invasion and mesenchymal transition through a MET/VEGFR2 complex. Cancer Cell 2012;22:21-35. [Crossref] [PubMed]
- Takahashi S. Vascular endothelial growth factor (VEGF), VEGF receptors and their inhibitors for antiangiogenic tumor therapy. Biol Pharm Bull 2011;34:1785-8. [Crossref] [PubMed]
- Thompson LU, Chen JM, Li T, et al. Dietary flaxseed alters tumor biological markers in postmenopausal breast cancer. Clin Cancer Res 2005;11:3828-35. [Crossref] [PubMed]
- Kuijsten A, Arts IC, Hollman PC, et al. Plasma enterolignans are associated with lower colorectal adenoma risk. Cancer Epidemiol Biomarkers Prev 2006;15:1132-6. [Crossref] [PubMed]
- Zhou Y, Liu YE, Cao J, et al. Vitexins, nature-derived lignan compounds, induce apoptosis and suppress tumor growth. Clin Cancer Res 2009;15:5161-9. [Crossref] [PubMed]
- Zhang G, Li D, Chen H, et al. Vitexin induces G2/M-phase arrest and apoptosis via Akt/mTOR signaling pathway in human glioblastoma cells. Mol Med Rep 2018;17:4599-604. [Crossref] [PubMed]
- He JD, Wang Z, Li SP, et al. Vitexin suppresses autophagy to induce apoptosis in hepatocellular carcinoma via activation of the JNK signaling pathway. Oncotarget 2016;7:84520-32. [Crossref] [PubMed]
- Lee CY, Chien YS, Chiu TH, et al. Apoptosis triggered by vitexin in U937 human leukemia cells via a mitochondrial signaling pathway. Oncol Rep 2012;28:1883-8. [Crossref] [PubMed]
- Kodama M, Kodama T, Newberg JY, et al. In vivo loss-of-function screens identify KPNB1 as a new druggable oncogene in epithelial ovarian cancer. Proc Natl Acad Sci U S A 2017;114:E7301-10. [Crossref] [PubMed]
- Inamdar S, Joshi A, Malik S, et al. Vitexin alleviates non-alcoholic fatty liver disease by activating AMPK in high fat diet fed mice. Biochem Biophys Res Commun 2019;519:106-12. [Crossref] [PubMed]
- Zhang L, Song Y, Ling Z, et al. R-spondin 2-LGR4 system regulates growth, migration and invasion, epithelial-mesenchymal transition and stem-like properties of tongue squamous cell carcinoma via Wnt/β-catenin signaling. EBioMedicine 2019;44:275-88. [Crossref] [PubMed]
- Min SO, Lee SW, Bak SY, et al. Ideal sphere-forming culture conditions to maintain pluripotency in a hepatocellular carcinoma cell lines. Cancer Cell Int 2015;15:95. [Crossref] [PubMed]
- Poornima P, Quency RS, Padma VV. Neferine induces reactive oxygen species mediated intrinsic pathway of apoptosis in HepG2 cells. Food Chem 2013;136:659-67. [Crossref] [PubMed]
- Wang S, Ma XY, Xia Y, et al. Expressions of Ki67, PCNA and mitotic index in ovarian epithelial tumors. Sichuan Da Xue Xue Bao Yi Xue Ban 2010;41:575-80. [PubMed]
- Liu ML, Chien LY, Tai CJ, et al. Effectiveness of Traditional Chinese Medicine for Liver Protection and Chemotherapy Completion among Cancer Patients. Evid Based Complement Alternat Med 2011;2011:291843. [Crossref] [PubMed]
- Liu X, Xiu LJ, Jiao JP, et al. Traditional Chinese medicine integrated with chemotherapy for stage IV non-surgical gastric cancer: a retrospective clinical analysis. J Integr Med 2017;15:469-75. [Crossref] [PubMed]
- Liu X, Jiang Q, Liu H, et al. Vitexin induces apoptosis through mitochondrial pathway and PI3K/Akt/mTOR signaling in human non-small cell lung cancer A549 cells. Biol Res 2019;52:7. [Crossref] [PubMed]
- An F, Wang S, Tian Q, et al. Effects of orientin and vitexin from Trollius chinensis on the growth and apoptosis of esophageal cancer EC-109 cells. Oncol Lett 2015;10:2627-33. [Crossref] [PubMed]
- Bengtsson E, Ranefall P. Image Analysis in Digital Pathology: Combining Automated Assessment of Ki67 Staining Quality with Calculation of Ki67 Cell Proliferation Index. Cytometry A 2019;95:714-6. [Crossref] [PubMed]
- Portugal J, Bataller M, Mansilla S. Cell death pathways in response to antitumor therapy. Tumori 2009;95:409-21. [Crossref] [PubMed]
- Bhardwaj M, Cho HJ, Paul S, et al. Vitexin induces apoptosis by suppressing autophagy in multi-drug resistant colorectal cancer cells. Oncotarget 2018;9:3278-91. [Crossref] [PubMed]
- Choi HJ, Eun JS, Kim BG, et al. Vitexin, an HIF-1alpha inhibitor, has anti-metastatic potential in PC12 cells. Mol Cells 2006;22:291-9. [PubMed]
- Guyot M, Hilmi C, Ambrosetti D, et al. Targeting the pro-angiogenic forms of VEGF or inhibiting their expression as anti-cancer strategies. Oncotarget 2017;8:9174-88. [Crossref] [PubMed]
- Scarpa ES, Antonini E, Palma F, et al. Antiproliferative activity of vitexin-2-O-xyloside and avenanthramides on CaCo-2 and HepG2 cancer cells occurs through apoptosis induction and reduction of pro-survival mechanisms. Eur J Nutr 2018;57:1381-95. [Crossref] [PubMed]
- Rosa SI, Rios-Santos F, Balogun SO, et al. Vitexin reduces neutrophil migration to inflammatory focus by down-regulating pro-inflammatory mediators via inhibition of p38, ERK1/2 and JNK pathway. Phytomedicine 2016;23:9-17. [Crossref] [PubMed]
- Curtarello M, Tognon M, Venturoli C, et al. Rewiring of Lipid Metabolism and Storage in Ovarian Cancer Cells after Anti-VEGF Therapy. Cells 2019;8:1601. [Crossref] [PubMed]
(English Language Editor: B. Madden)